Abstract
Purpose
The purpose of the present study was to determine the extent and seasonal prevalence of Arcobacter spp. in domestic poultry and wild birds in the Kars region of Turkey using multiplex polymerase chain reaction (m-PCR).
Methods
In this study, 1570 samples were collected from domestic poultry and wild avian species. The numbers of collected samples were as follows: 182 fecal samples from chickens, geese, and turkeys from family farms in the Kars region in Turkey; 1089 cloacal swab samples from chickens, geese, ducks, turkeys, and quails from family farms in this region; and 299 fecal samples from wild pigeons, crows, and owls in the same region.
Results
Arcobacter spp. were isolated from 17.43%, 35.77%, 3.63%, 6.87%, and 3.33% of the cloacal swab samples obtained from geese, ducks, chickens, turkeys, and quails, respectively. In the stool samples, Arcobacter spp. were isolated from 9.62%, 13.33%, and 4% of chicken, goose, and turkey samples, respectively. In wild birds, the isolation rates of Arcobacter spp. were 6.6%, 12.15%, and 0% in pigeons, crows, and owls, respectively. Using m-PCR, among 171 Arcobacter spp. isolates obtained from poultry and wild birds, 67, 78, 24, and 2 were identified as Arcobacter cryaerophilus, Arcobacter butzleri, Arcobacter skirrowii, and Arcobacter cibarius, respectively.
Conclusions
Both poultry and wild avian species exhibited variable rates of Arcobacter species positivity. The presence of Arcobacter spp. in the digestive tracts of healthy poultry and wild birds may serve as a potential reservoir for the dissemination of these microbes in the environment and their transmission to other animals and humans.
Similar content being viewed by others
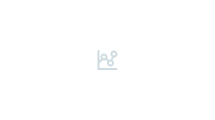
Introduction
Arcobacter spp. are small (0.2–0.9 × 0.5–3 μm), spiral-shaped, and Gram-negative bacteria. They are non-spore forming and show corkscrew-like motility, aided by nonshielded polar flagella (Kayman 2012). In contrast to the genus Campylobacter, Arcobacter species are mostly aerotolerant and able to grow at temperatures below 30 °C (Brückner et al. 2020), although Arcobacter anaerophilus is an obligate anaerobe in the genus (Sasi Jyothsna et al. 2013). Therefore, Arcobacter species are separated from campylobacters due to differences in their structural properties and fatty acid profiles (Gonulalan and Ertas Onmaz 2015), together with their ability to grow at temperatures of 15–30 °C and under aerobic conditions (Kayman 2012). The Arcobacter genus is a member of the Campylobacteraceae family and rRNA superfamily VI in the Epsilon division of Proteobacteria (Vandamme and De Ley 1991, On 2001).
To date, 28 Arcobacter species have been identified and characterized (Kim et al. 2019), which have been isolated from various hosts, foods, and environmental sources (Talay et al. 2016), such as sewage (Collado and Figueras 2011), streams and rivers (Laishram et al. 2016; Talay et al. 2016), drinking water, and municipal water (Ertas et al. 2010; Jalava et al. 2014). Arcobacter spp. have been isolated from various clinical samples from humans and animals (Petersen et al. 2007; Adesiji et al. 2011). They have been found in intestinal and fecal samples from a range of farm animals (Levican et al. 2013; Piva et al. 2013; Shakira et al. 2012); cloacal swabs and fecal samples from domestic poultry such as chickens, ducks, geese, and turkeys (Collado and Figueras 2011; Goni et al. 2016); and intestinal and fecal samples from numerous wild avian species (Fernández et al. 2007; Wesley and Schroeder-Tucker 2011; Di Francesco et al. 2014).
Within the genus, Arcobacter butzleri, Arcobacter cryaerophilus, and Arcobacter skirrowii are the most common pathogenic species and are associated with various infections in humans and animals (Van den Abeele et al. 2014). These infections mostly appear clinically as abortion, enteritis, and mastitis in domestic animals (Patyal et al. 2011) and as gastroenteritis, bacteriemia, endocarditis, peritonitis, diarrhea, and septicemia in humans (Vandenberg et al. 2004; Samie et al. 2007). The primary potential transmission sources of these agents are various foods, especially poultry and products thereof, together with contaminated water (Shah et al. 2012).
Healthy domestic and wild avian species that harbor Arcobacter spp. in their digestive tracts serve as important hosts for these pathogens (Atabay et al. 1998; Van Driessche et al. 2003). Feces of avian Arcobacter reservoirs play a major role in the transmission of arcobacters to the environment, other animals, and humans. Therefore, both domestic poultry and wild birds are important in the carriage and spread of arcobacters (Di Francesco et al. 2014).
There have been no previous studies aimed at the isolation and identification of Arcobacter spp. in domestic poultry and wild birds in the Kars region in Turkey. The aim of the present study was to determine the extent and seasonal prevalence of Arcobacter spp. in domestic poultry (geese, chickens, ducks, turkeys, and quails) and wild birds (crows, pigeons, and owls) in the Kars region using the m-PCR protocol.
Material and methods
Reference strains
The Arcobacter butzleri (CIP 103493), A. cryaerophilus (CIP 104014), and A. skirrowii (CIP 103588) reference strains used during the stages of isolation and molecular identification were kindly provided by Prof. Francis Megraud (Bacteriology Laboratory of Victor Sagalen Bordeaux Hospital, France).
Domestic poultry and wild bird samples
In this study, 1089 cloacal swab samples (chickens, n = 358; geese, n = 327; turkeys, n = 131; ducks, n = 123; quails, n = 150) and 182 fecal samples (chickens, n = 52; geese, n = 105; turkeys, n = 25) were obtained from domestic poultry raised on family farms in the Kars region from October 2013 to June 2015. Fecal samples were not collected from ducks or quails. In addition, 299 fresh fecal samples were collected from wild birds (pigeons, n = 167; crows, n = 107; owls, n = 25) in the same region from March 2011 to December 2015.
Isolation and identification of arcobacters
Cloacal swab and fresh fecal samples from domestic poultry and wild birds were placed in 5 ml of arcobacter selective broth (Fluka, 59848, India) containing cefoperazone, amphotericin B, and teicoplanin (CAT selective supplement) (Oxoid, SR174E, UK) and transferred to the microbiology laboratory within 3 to 4 h under cold chain conditions. Swabs were incubated at 30 °C for 48 h under microaerobic conditions using an Anaerocult C kit (Merck, 1.16275, Germany) for pre-enrichment. After incubation, the membrane filtration method recommended by Atabay and Corry (1997) was performed for enriched samples. All plates were incubated at 30 °C for 2 to 7 days in a microaerobic atmosphere.
Phenotypic tests such as indoxyl acetate hydrolyzation, nitrate reduction, and H2S reduction tests together with Gram staining, motility examination, and catalase, oxidase and urease tests were carried out on colonies grown on blood agar plates. Aerotolerance (25 °C and O2, 30 °C and O2-CO2, 37 °C and O2-CO2, 42 °C and CO2), growth in MacConkey agar (Oxoid, CM1169, England), and growth in the presence of 2–3.5% sodium chloride were evaluated. Arcobacter spp. isolates were kept at − 20 °C to be used for molecular identification.
DNA extraction
A modified version of the boiling method of Dashti et al. (2009) was used for DNA extraction from suspected Arcobacter isolates. For this purpose, a few colonies belonging to the isolates incubated at 30 °C under microaerobic conditions in blood agar were maintained at 99.9 °C for 10 min in Tris-EDTA buffer (Sigma, 93283, Germany), after which the tubes were placed at +4 °C for 10 min and then centrifuged for 10 min at 10,000 rpm. The obtained supernatants were used as template DNA suspensions.
M-PCR
First, the m-PCR methods described by Houf et al. (2000) and Douidah et al. (2010) were applied for the species-level identification of Arcobacter spp. isolates. For these assays, a specific primer set (Arco, Skir, Butz, Cry1 and Cry2) targeting 16S rRNA and 23S rRNA sequences under the m-PCR method described by Houf et al. (2000) was used. Then, the m-PCR method described by Douidah et al. (2010) was applied for the identification of the A. cryaerophilus isolates. In this m-PCR assay, species-specific primers for A. butzleri, A. cibarius, A. cryaerophilus, A. skirrowii, and Arcobacter thereuis (ButR, CibR, SkiR, TheR, ArcoF, CryF, CryR, GyrasF, GyrasR) were used.
For the m-PCR assay described by Houf et al. (2000), each PCR assay was carried out in a 20-μl volume consisting of 10 μl of Taq Master Mix (Qiagen, Cat No./ID: 201443), 20 pmol of primers (Arco, Skir, Butz, Cry1 and Cry2), 1.5 μl of DNase-free water, and 4 μl of template DNA. The thermal cycling conditions for each m-PCR assay were as follows: 94 °C for 2 min (predenaturation), followed by 35 cycles of 94 °C for 45 s (denaturation), 61 °C for 45 s (annealing), and 72 °C for 30 s (extension) and a final extension at 72 °C for 10 min. For the m-PCR assay described by Douidah et al. (2010), each PCR assay was carried out in a 25-μl volume consisting of 5 μl of Taq Master Mix (Qiagen, Cat No./ID: 201443), 50 pmol of primers (ButR, CibR, SkiR, TherR, ArcoF, CryF, CryR, GyrasF and GyrasR), 4.3 μl of DNase-free water, and 4 μl of template DNA. Prior to cycling, the samples were heated at 94 °C for 3 min. The PCR assay involved 30 cycles of denaturation (94 °C, 45 s), primer annealing (58 °C, 45 s), and chain extension (72 °C, 2 min). The amplified products were evaluated by electrophoresis in 1.5% agarose gels in 1X Tris-Boric Acid-EDTA buffer. The gels were run at 120 volts and 300 milliamperes for 25 min.
Results
Isolation results
In this study, Arcobacter spp. were isolated at different rates in domestic poultry and wild birds. Arcobacter spp. were isolated from 121 (11.11%) out of 1089 cloacal swab samples and 20 (10.99%) out of 182 feces samples from domestic poultry. The rates of positive cloacal swab samples were 34.95% (43/123) for ducks, 16.21% (53/327) for geese, 6.87% (9/131) for turkeys, 3.33% (5/150) for quails, and 3.07% (11/358) for chickens. The positive rates for feces samples were 12.38% in geese, 9.62% in chickens, and 4% in turkeys. However, A. butzleri and A. cryaerophilus were isolated together from three goose cloacal swab samples and one duck sample.
In the analysis of fecal samples from wild birds, no Arcobacter spp. were isolated in the owl (n = 25) samples. The Arcobacter isolation rates in crows and pigeons were 12.15% (13/107) and 6.6% (11/167), respectively.
Identification results
The m-PCR assays identified the isolates as A. cryaerophilus (395 bp, Douidah et al. 2010), A. butzleri (401 bp, Houf et al. 2000), A. skirrowii (641 bp, Houf et al. 2000), and A. cibarius (1125 bp, Douidah et al. 2010), and the identification results are summarized in Table 1.
The m-PCR method defined by Houf et al. (2000) was used to identify the isolates obtained in the present study at the species level. As a result of this method, 84 A. cryaerophilus, 78 A. butzleri, and 7 A. skirrowii isolates were identified. Then, the m-PCR method proposed by Douidah et al. (2010) was applied to identify A. cryaerophilus isolates. As a result of this m-PCR method, 65 of the 84 A. cryaerophilus isolates were identified as A. cryaerophilus again, but 17 isolates were identified as A. skirrowii and 2 as A. cibarius.
Seasonal evaluation results
The isolation rates of Arcobacter spp. varied according to season and poultry type. In the cloacal swab samples from geese, the rate was highest in winter (23.53%), followed by summer (21.17%), autumn (18.52%), and spring (9.80%). In fecal samples from geese, the highest rate was observed in summer (20.75%), followed by spring (9.1%) and autumn (5%). No isolates were detected in winter. For ducks, sampling was performed only in spring and summer, and the isolation rate was higher in summer than in spring (37.74% and 33.33%, respectively). In the cloacal swab samples from chickens, the highest isolation percentage was detected in summer (6.12%), followed by winter (2.8%), and no isolation was carried out in autumn and spring. For turkeys, the bacterial isolation rate was highest in winter (17.39%), followed by summer (7.41%), and no Arcobacter isolates were detected in autumn and spring. In the fecal samples, isolation (10%) was carried out only in spring. For quails, sampling was performed only in autumn and winter periods. The isolation rate was 7.14% in autumn. Among wild birds, fecal samples were obtained from crows and pigeons only in summer and spring periods. The highest isolation rate was observed in summer (13.97% for crows and 7.4% for pigeons). The variation in the isolation rates of Arcobacter spp. according to the season and avian species are shown in Table 2.
Discussion
The Arcobacter spp. isolation rate varied in both domestic poultry and wild birds. In cloacal swab samples, the highest isolation rate was found in ducks (35.77%), followed by geese (17.43%), turkeys (6.87%), quails (3.33%), and chickens (3.63%). The rates detected in fecal samples were 13.33%, 9.62%, and 4% in geese, chickens, and turkeys, respectively.
The isolation rate observed in cloacal swab samples from geese was similar to that reported by Atabay et al. (2008) (18%) and higher than that reported by Bogantes et al. (2015) (0%). The Arcobacter isolation rate detected in duck cloacal swab samples in the present study (35.77%) was very close to that reported by Fernández et al. (2007) (40%), whereas it was much higher than that found by Bogantes et al. (2015) (5%) and Silha et al. (2015) (4.2%). In contrast, A. cibarius was identified in two goose cloacal swab samples by m-PCR. The differences in the isolation rates in geese and ducks may be attributed to the sampling times, feeding conditions, contact with other animals, and access to contaminated water. Nevertheless, compared with those in other domestic poultry species, the Arcobacter isolation rate was highest in samples from geese and ducks. This finding is in accordance with those of other studies (Atabay et al. 2006; Gonzalez et al. 2007) that have concluded that geese and ducks are potential reservoirs of arcobacters. However, these discrepancies may reflect different methodological aspects, such as the amount of sample analyzed, type of culture medium, incubation atmosphere, and even the geographic area where sampling was performed.
In the present study, the Arcobacter isolation rate obtained from cloacal swab samples from chickens (3.63%) was lower than the rate found by Van Driessche and Houf (2007) (10%). Ho et al. (2008) detected no Arcobacter isolates in chicken cloacal swab samples. The findings of the present study on the Arcobacter isolation rate from chicken fecal samples (9.62%) were compatible with those found by Atabay et al. (2006) (4.3%) and Mohan et al. (2014) (8%). They were lower than those reported by Ho et al. (2008) (16.7%), Bogantes et al. (2015) (16%), and Fernandez et al. (2015) (30%). Previous studies that have examined samples of chicken intestinal contents have reported differences in isolation rates ranging between 0% and 100% (Van Driessche and Houf 2007; Ho et al. 2008).
In this study, the Arcobacter isolation rates in turkey cloacal swabs and fecal samples were 6.87% and 4%, respectively. These rates were higher than those found by Andersen et al. (2007) (2%), and the isolation rate in fecal samples was lower than that reported by Fernández et al. (2007) (28.6%).
Only a few studies have examined the presence of Arcobacter spp. in quails (Ok Anadut and Gumussoy 2005; Rahimi 2014). In the present study, the Arcobacter isolation rate in quails was 3.33%. The different isolation rates in samples obtained from various kinds of poultry may depend on geographic- and region-specific characteristics, farming styles, the isolation and identification methods used, sample collection, and transportation conditions and proximity to other animals. In the present study, Arcobacter isolation rates were considerably lower in quails than in ducks and geese (i.e., aquatic poultry). As noted in previous studies (Wesley and Miller 2010; Fernandez et al. 2015), this finding supports the hypothesis that arcobacters are not permanent commensals of the intestinal flora of poultry because of the high body temperatures of these animals (40.5–42 °C).
In this study, the identification of Arcobacter isolates using two m-PCR assays in domestic poultry was performed, and the isolates were initially identified as A. cryaerophilus, followed by A. butzleri and A. skirrowii. Similar to the findings of other studies (Rahimi et al. 2012), in this study, the most commonly isolated species in all the samples was initially A. cryaerophilus and were subsequently A. butzleri and A. skirrowii. In accordance with the findings of previous studies (Rahimi et al. 2012), the results of species-specific m-PCR revealed that the most common species in the samples from wild birds was A. butzleri, followed by A. cryaerophilus and A. skirrowii. Only a few studies have detected the presence of A. skirrowii in wild birds (Van Driessche and Houf 2007; Adesiji et al. 2011). In the present study, A. skirrowii was isolated and identified, albeit at a low rate, in ducks and chickens compared to geese. The distribution of Arcobacter spp. in different organisms may change depending upon the relationships of the hosts with other organisms and their environment as well as the ecological features and environmental conditions to which they are adapted.
In the present study, the samples were first assessed according to the presence of A. cryaerophilus, A. butzleri, and A. skirrowii. For this purpose, the m-PCR method described by Houf et al. (2000) was used for identification of the obtained Arcobacter isolate sat the species level. Nevertheless, according to data obtained from other studies, while this m-PCR method presents the advantage of 100% reliable identification of A. butzleri, it produces false-positive identification results for A. cryaerophilus (Levican and Figueras 2013). Therefore, an m-PCR assay developed by Douidah et al. (2010) that detects 5 Arcobacter species (A. butzleri, A. cryaerophilus, A. skirrowii, and A. cibarius and A. thereius) was used. This m-PCR assay was preferred in the second step in the current study. The confirmation of the A. cryaerophilus isolates identified in the first m-PCR assay was thus performed, and other species that might be in the samples were then identified. Nevertheless, it is thought that different assays are necessary for the correct identification of these species and other potential pathogenic Arcobacter spp.
Although many studies have examined the presence of arcobacters in domestic poultry (Bogantes et al. 2015; Hassan 2017), only a few such studies have included wild birds (Di Francesco et al. 2014; Giacometti et al. 2015). In a study in Italy where cloacal swab samples were taken from 95 Eurasian collared doves (Streptopelia decaocto), Arcobacter spp. were detected in 18 (19%) samples by nested PCR amplification of the 16S rRNA gene (Di Francesco et al. 2014). However, Giacometti et al. (2015) detected no Arcobacter spp. in a study of fecal samples from 47 wild pigeons (Columbia livia). In a study in Chile of fecal samples from 60 pelicans and 60 sparrows, Arcobacter spp. were isolated at a rate of 13.3% in pelicans and 6.7% in sparrows, and the obtained species was identified as A. butzleri (Fernández et al. 2007). In the present study, the analysis of fecal samples from wild birds detected no Arcobacter spp. isolates in samples from owls (n = 25). The isolation rates in crows and pigeons were 12.15% (13/107) and 6.6% (11/167), respectively. The differences among isolation rates may be derived from different avian species or their association with water.
In the present study, according to the results of the m-PCR assay, 10 wild pigeon isolates were identified as A. butzleri, and one isolate was identified as A. cryaerophilus, while 12 crow isolates were identified as A. butzleri, and one was identified as A. cryaerophilus. The bacterial isolation rate in wild pigeons in the present study was lower than that reported by Di Francesco et al. (2014) (19%). However, Di Francesco et al. (2014) utilized the direct PCR technique to identify Arcobacter spp. in cloacal swab samples from Eurasian collared doves, whereas the present study employed culture methods. There are difficulties in detecting Arcobacter spp. via culture methods. It was not possible to compare the findings regarding Arcobacter isolation rates in wild birds obtained in the present study with those in the literature due to the limited number of studies involving Arcobacter isolation and identification in wild birds (Di Francesco et al. 2014; Giacometti et al. 2015). However, the discovery of Arcobacter isolates in wild avian species in the Kars region suggests that they may play an important role in transferring arcobacters to humans and other birds or animals via the contamination of the environment and water.
In this study, it was not possible to compare the seasonal distribution of Arcobacter isolation rates across all four seasons, as samples could not be obtained from the studied poultry in each season. However, where seasonal isolation rates in domestic poultry could be evaluated, the highest values were found in winter for geese, in summer for ducks, summer for chickens, in winter for turkeys, and in autumn for quails. Therefore, the seasonal distribution of arcobacters in domestic poultry seems to vary. Such variation may be associated with the bird’s age, growth stage, and type of poultry farming. Therefore, more extensive studies are needed to shed light on the seasonal distribution of Arcobacter isolation rates.
In wild birds (wild pigeon and crow), the seasonal distribution of Arcobacter could be examined only in spring and summer. No Arcobacter spp. were isolated from fecal samples collected in spring months, while Arcobacter was isolated from samples collected in summer.
Conclusion
The present study revealed that most domestic poultry (goose, duck, chicken, turkey, and quail) and some wild birds (crow and pigeon) carried Arcobacter, with varying bacterial isolation rates. By harboring Arcobacter species in their digestive systems, these birds may serve as potential reservoirs for the dissemination of Arcobacter species in the environment and their transmission to other birds, animals, and humans. Duck, goose, and chicken farming in the Kars region are mainly based on traditional practices, in which birds are housed with other farm animals. This may give rise to a significant risk of Arcobacter infections in domestic animals and humans in the region.
References
Adesiji YO, Coker AO, Oloke JK (2011) Detection of Arcobacter in feces of healthy chickens in Osogbo, Nigeria. J Food Prot 74:119–121
Andersen MME, Wesley IV, Nestor E, Trampel DW (2007) Prevalence of Arcobacter species in market-weight commercial turkeys. Anton Leeuw 92:309–317
Atabay HI, Corry JE (1997) The prevalence of campylobacters and arcobacters in broiler chickens. J Appl Microbiol 83:619–626
Atabay HI, Corry JEL, On SLW (1998) Diversity and prevalence of Arcobacter spp. in broiler chickens. J Appl Microbiol 84:1007–1016
Atabay HI, Unver A, Sahin M, Otlu S, Elmali M, Yaman H (2008) Isolation of various Arcobacter species from domestic geese (Anser anser). Vet Microbiol 128:400–405
Atabay HI, Wainø M, Madsen M (2006) Detection and diversity of various Arcobacter species in Danish poultry. Int J Food Microbiol 109:139–145
Bogantes EV, Fallas-Padilla KL, Rodriguez-Rodriguez CE, Jaramillo HF, Echandi MLA (2015) Zoonotic species of the genus Arcobacter in poultry from different regions of Costa Rica. J Food Prot 78:808–811
Brückner V, Fiebiger U, Ignatius R, Friesen J, Eisenblätter M, Höck M, Alter T, Bereswill S, Heimesaat MM, Gölz G. Characterization of Arcobacter strains isolated from human stool samples: results from the prospective German prevalence study Arcopath. Gut Pathog. 2020;12(3):1–10.
Collado L, Figueras MJ (2011) Taxonomy, epidemiology, and clinical relevance of the genus Arcobacter. Clin Microbiol Rev 24:174–192
Dashti AA, Jadaon MM, Abdulsamad AM, Dashti HM (2009) Heat treatment of bacteria: a simple method of DNA extraction for molecular techniques. Kuwait Med J 41:117–122
Di Francesco A, Delogu M, Giacometti F, Stancampiano L, Grilli E, Guarniero I, Serraino A (2014) First detection of Arcobacter sp. in Eurasian collared doves (Streptopelia decaocto). Vet Ital 50:313–315
Douidah L, De Zutter L, Vandamme P, Houf K (2010) Identification of five human and mammal associated Arcobacter species by a novel multiplex-PCR assay. J Microbiol Methods 80:281–286
Ertas N, Dogruer Y, Gonulalan Z, Guner A, Ulger I (2010) Prevalence of Arcobacter species in drinking water, spring water, and raw milk as determined by multiplex PCR. J Food Prot 73:2099–3102
Fernández H, Vera F, Villanueva MP (2007) Arcobacter and Campylobacter species in birds and mammals from southern Chile. Arch Med Vet 39:163–165
Fernandez H, Villanueva MP, Mansilla I, Gonzalez M, Latif F (2015) Arcobacter butzleri and A. cryaerophilus in human, animals and food sources, in southern Chile. Braz J Microbiol 46:145–147
Giacometti F, Lucchi A, Di Francesco A, Delogu M, Grilli E, Guarniero I, Stancampiano L, Manfreda G, Merialdi G, Serrainoa A (2015) Arcobacter butzleri, Arcobacter cryaerophilus and Arcobacter skirrowii circulation in a dairy farm and sources of milk contamination. Appl Environ Microbiol 81:5055–5063
Goni DM, Abdulaziz S, Dhaliwal GK, Zakaria Z, Muhammad IJ, Mohamed MA, Bello AA, Bitrus AA (2016) Occurrence of Arcobacter in dogs and cats in Selangor, Malaysia, and associated risk factors. Turk J Vet Anim Sci 40:1–7
Gonulalan Z, Ertas Onmaz N (2015) Arcobacter. Review. Turkiye Klinikleri J Food Hyg and Technol-Special Topics 1:42–48
Gonzalez AI, Botella S, Montes RM, Moreno Y, Ferrus MA (2007) Direct detection and identification of Arcobacter species by multiplex PCR in chicken and wastewater samples from Spain. J Food Prot 70:341–347
Hassan AK (2017) Detection and identification of Arcobacter species in poultry in Assiut governorate, upper Egypt. J Adv Vet Res 2:53–58
Ho HTK, Lipman LJA, Gaastra W (2008) The introduction of Arcobacter spp. in poultry slaughterhouses. Int J Food Microbiol 125:223–229
Houf K, Tutenel A, De Zutter L, Van Hoof J, Vandamme P (2000) Development of a multiplex PCR assay for the simultaneous detection and identification of Arcobacter butzleri, Arcobacter cryaerophilus and Arcobacter skirrowii. FEMS Microbiol Lett 193:89–94
Jalava RH, Ollgren J, Maunula L, Gomez-Alvarez V, Revez J, Palander M, Antikainen J, Kauppinen A, Rasanen P, Siponen S, Nyholm O, Kyyhkynen A, Hakkarainen S, Merentie J, Parnanen M, Loginov R, Ryu H, Kuusi M, Siitonen A, Miettinen I, Domingo JWS, Hanninen ML, Pitkanen T (2014) Novel microbiological and spatial statistical methods to improve strength of epidemiological evidence in a community-wide waterborne outbreak. PLoS One 9:e104713
Kayman T (2012) Arcobacter cinsi: Genel özellikleri, epidemiyoloji ve laboratuvar tanısı. Turk Mikrobiyol Cem Derg 42:43–50
Kim NH, Park SM, Kim HW, Cho TJ, Kim SH, Choi C, Rhee MS (2019) Prevalence of pathogenic Arcobacter species in South Korea: comparison of two protocols for isolating the bacteria from foods and examination of nine putative virulence genes. Food Microbiol 78:18–24
Laishram M, Rathlavath S, Lekshmi M, Kumar S, Nayak BB (2016) Isolation and characterization of Arcobacter spp. from fresh seafood and the aquatic environment. Int J Food Microbiol 232:87–89
Levican A, Collado L, Figueras MJ (2013) Arcobacter cloacae sp. nov. and Arcobacter suis sp. nov., two new species isolated from food and sewage. Syst Appl Microbiol 36:22–27
Levican A, Figueras MJ (2013) Performance of five molecular methods for monitoring Arcobacter spp. BMC Microbiol 13:220
Mohan HV, Rathore RS, Dhama K, Ramees TP, Patyal A, Bagalkot PS, Wani MY, Bhilegaonkar KN, Kumar A (2014) Prevalence of Arcobacter spp. in humans, animals and foods of animal origin in India based on cultural isolation, antibiogram, PCR and multiplex PCR detection. Asian J AnimVet Adv 9:452–466
Ok Anadut F, Gumussoy KS (2005) Isolatıon of Arcobacter spp. From Poultry Carcasses Put on Consumption in Kayseri. J Health Sci 14:125–131
On SLW. Taxonomy of Campylobacter, Arcobacter, Helicobacter and related bacteria: current status, future prospects and immediate concerns. J Appl Microbiol. 2001;90:1–15.
Patyal A, Rathore RS, Mohan HV, Dhama K, Kumar A (2011) Prevalence of Arcobacter spp. in humans, animals and foods of animal origin including sea food from India. Transbound Emerg Dis 58:402–410
Petersen RF, Harrington CS, Kortegaard HE, On SL (2007) A PCR-DGGE method for detection and identification of Campylobacter, Helicobacter, Arcobacter and related Epsilobacteria and its application to saliva samples from humans and domestic pets. J Appl Microbiol 103:2601–2615
Piva S, Serraino A, Florio D, Giacometti F, Pasquali F, Manfreda G, Zanoni ZG (2013) Isolation of Arcobacter species in water buffaloes (Bubalus bubalis). Foodborne Pathog Dis 10:475–477
Rahimi E (2014) Prevalence and antimicrobial resistance of Arcobacter species isolated from poultry meat in Iran. Br Poultry Sci 55:174–180
Rahimi E, Hormozipoor H, Gholami Ahangaran M, Yazdi F (2012) Prevalence of Arcobacter species on chicken carcasses during processing in Iran. J Appl Poultry Res 21:407–412
Samie A, Obi CL, Barrett LJ, Powell SM, Guerrant RL (2007) Prevalence of Campylobacter species, Helicobacter pylori and Arcobacter species in stool samples from the Venda region, Limpopo, South Africa: studies using molecular diagnostic methods. J Inf Secur 54:558–566
Sasi Jyothsna TS, Rahul K, Ramaprasad EV, Sasikala C, Ramana CV (2013) Arcobacter anaerophilus sp. nov., isolated from an estuarine sediment and emended description of the genus Arcobacter. Int J Syst Evol Microbiol 63:4619–4625
Shah AH, Saleha AA, Murugaiyah M, Zunita Z, Memon AA (2012) Prevalence and distribution of Arcobacter spp. in raw milk and retail raw beef. J Food Prot 75:1474–1478
Shakira N, Noh M, Murugaiyah M, Abdül Aziz S (2012) Occurrence of Campylobacter spp. and Arcobacter spp. in goats. In: 7th proceedings of the seminar on veterinary sciences 27 February - 02 March
Silha D, Silhova-Hruskova L, Vytrasova J (2015) Modified isolation method of Arcobacter spp. from different environmental and food samples. Folia Microbiol 60:515–521
Talay F, Celenk M, Atabay HI (2016) Isolation and identification of Arcobacter species from environmental and drinking water samples. Folia Microbiol 61:479–484
Van den Abeele AM, Vogelaers D, Van Hende J, Houf K (2014) Prevalence of Arcobacter species among humans, Belgium, 2008-2013. Emerg Infect Dis 20:1731–1734
Van Driessche E, Houf K (2007) Discrepancy between the occurrence of Arcobacter in chickens and broiler carcass contamination. Poult Sci 86:744–751
Van Driessche E, Houf K, Van Hoof J, De Zutter L, Vandamme P (2003) Isolation of Arcobacter species from animal feces. FEMS Microbiol Lett 229:243–248
Vandamme and De Ley. Proposal a New Family, Campylobacteraceae. Int J Syst Bacteriol. 1991;41(3):451–5.
Vandenberg O, Dediste A, Houf K, Ibekwem S, Souayah H, Cadranel S, Douat N, Zissis G, Butzler JP, Vandamme P (2004) Arcobacter species in humans. Emerg Infect Dis 10:1863–1867
Wesley IV, Miller WG (2010) Arcobacter: an opportunistic human foodborne pathogen? In: Scheld WM, Grayson ML, Hughes JM (eds) Emerging infections, 9. ASM Press, Washington, pp 185–211
Wesley IV, Schroeder-Tucker L (2011) Recovery of Arcobacter ssp. from nonlivestock species. J Zoo Wildl Med 42:508–512
Acknowledgements
This doctoral thesis was supported as project number 2015-TS-10 by the Kafkas University Scientific and Technological Research Fund.
Research involving human participitants and/or animals
N/A
Informed consent
N/A
Funding
This doctoral thesis was supported as project number 2015-TS-10 by the Kafkas University Scientific and Technological Research Fund.
Author information
Authors and Affiliations
Contributions
The authors read and approved the final manuscript.
Corresponding author
Ethics declarations
Ethics approval and consent to participate
The research was carried out in accordance with the report numbered 2014-005 of the Faculty of Veterinary Medicine Experimental Animals Ethics Committee of the Kafkas University.
Competing interests
The authors declare that they have no conflict of interest.
Additional information
Publisher’s Note
Springer Nature remains neutral with regard to jurisdictional claims in published maps and institutional affiliations.
Rights and permissions
Open Access This article is licensed under a Creative Commons Attribution 4.0 International License, which permits use, sharing, adaptation, distribution and reproduction in any medium or format, as long as you give appropriate credit to the original author(s) and the source, provide a link to the Creative Commons licence, and indicate if changes were made. The images or other third party material in this article are included in the article's Creative Commons licence, unless indicated otherwise in a credit line to the material. If material is not included in the article's Creative Commons licence and your intended use is not permitted by statutory regulation or exceeds the permitted use, you will need to obtain permission directly from the copyright holder. To view a copy of this licence, visit http://creativecommons.org/licenses/by/4.0/.
About this article
Cite this article
Çelik, E., Otlu, S. Isolation of Arcobacter spp. and identification of isolates by multiplex PCR from various domestic poultry and wild avian species. Ann Microbiol 70, 60 (2020). https://doi.org/10.1186/s13213-020-01603-7
Received:
Accepted:
Published:
DOI: https://doi.org/10.1186/s13213-020-01603-7