Abstract
Background
With a preceding scrutiny of bacterial cellular responses against heat shock and oxidative stresses, current research further investigated such impact on yeast cell. Present study attempted to observe the influence of high temperature (44–46 °C) on the growth and budding pattern of Saccharomyces cerevisiae SUBSC01. Effect of elevated sugar concentrations as another stress stimulant was also observed. Cell growth was measured through the estimation of the optical density at 600 nm (OD600) and by the enumeration of colony forming units on the agar plates up to 450 min.
Results
Subsequent transformation in the yeast morphology and the cellular arrangement were noticed. A delayed and lengthy lag phase was observed when yeast strain was grown at 30, 37, and 40 °C, while at 32.5 °C, optimal growth pattern was noticed. Cells were found to lose culturability completely at 46 °C whereby cells without the cytoplasmic contents were also observed under the light microscope. Thus the critical growth temperature was recorded as 45 °C which was the highest temperature at which S. cerevisiae SUBSC01 could grow. However, a complete growth retardation was observed at 45 °C with the high concentrations of dextrose (0.36 g/l) and sucrose (0.18 g/l). Notably, yeast budding was found at 44 and 45 °C up to 270 min of incubation, which was further noticed to be suppressed at 46 °C.
Conclusions
Present study revealed that the optimal and the critical growth temperatures of S. cerevisiae SUBSC01 were 32.5 and 45 °C, respectively; and also projected on the inhibitory concentrations of sugars on yeast growth at that temperature.
Similar content being viewed by others
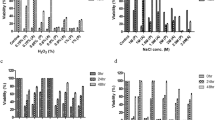
Findings
Stress responses in bacterial cells and to some extent in the yeast cells have been well studied so far [1–18]. Abrupt changes in the environmental and physicochemical stimuli including temperature, pH, sugar/salt concentrations, the redox state, toxic compounds and nutrient exhaustion have been mostly found to elicit a battery of defending response by up-regulating the genes encoding heat shock proteins (HSPs) in bacterial cells [19–27]. Like bacteria, the heat shock response in Saccharomyes cerevisiae, the model experimental yeast species, has been also characterized by the rapid changes in their cellular physiology including the budding manner accompanied with the increased tolerance against elevated salt and sugar concentrations, and against reactive oxygen species (ROS) [1–4, 7, 9–11, 15, 18, 28–30]. In S. cerevisiae, heat-sensitivity is ordinarily prescriptive of defects in protein coding genes which are also essential for maintaining the cell viability [10, 19, 24, 31–34]. The coupling consequence of heat stress together with the osmotic shock has been found to influence the cellular degeneration along with the retardation in cell division in yeast cells [3, 7, 35].
Our earlier studies revealed the bacterial cellular adaptation in response to the heat shock and against the elevated amount of intracellular reactive oxygen species (ROS) [14, 17, 36–38]. However, the work on stress response in yeast cells is scarce in the local perspective. These led us to broaden the research interest in the yeast cells to assess the optimal and critical growth temperatures and further to investigate intensely the growth changes at the critical temperature accompanied with the simulated stressed condition of an ascending osmotic pressure. Thus, apart from our earlier experiments on bacterial stress responses, current study was designed to observe the stress response in S. cerevisiae SUBSC01 towards heat shock and elevated sugar concentrations. The key observation revealed that while at 45 °C the yeast strain could grow, conversely growth inhibition was noticed upon supplementation of high concentrations of sugars.
Methods
On the basis of strain availability, laboratory stock cultures of S. cerevisiae SUBSC01 were used. Sabouraud Dextrose Agar (SDA) (Hi-Media Laboratories Pvt. Ltd., India), Sabouraud dextrose broth (SDB) (Difco Laboratories, Inc. USA) and Sucrose broth (SB) (Sigma-Aldrich Corporation, USA) were used. Pre-cultures were prepared by inoculating 5 ml SDB by a loopful of colony from the freshly prepared yeast culture plates, followed by incubation at 30, 32.5, 37 and 40 °C in static condition up to 72 h. The optical density at 600 nm (OD600) and the capability to form the colony forming units (CFUs) were monitored at the specific time intervals [13]. To determine the critical growth temperature, growth was monitored at 44, 45 and at 46 °C. For morphological observations, an aliquot of 5 µl from each of the culture suspension was removed at 90 min intervals [17, 39, 40]. For spot dilution tests, 1 ml of the culture suspension at same intervals was removed and serially diluted in 9 ml dextrose broth up to 10−4 [17]. An aliquot of 5 µl from each dilution was then spotted onto SDA plates following incubation at 32.5 °C for 24 h. To observe the osmotic effect on cell growth, different concentrations of dextrose including 0.04 g/l (1X), 0.12 g/l (3X), 0.2 g/l (5X), 0.28 g/l (7X), 0.36 g/l (9X) and sucrose, i.e., 0.02 g/l (1X), 0.06 g/l (3X), 0.1 g/l (5X), 0.14 g/l (7X), and 0.18 g/l (9X) were used. All experiments were conducted in triplicates. Statistical analysis regarding yeast growth was performed by determining the P value (~0.3) through t test. Standard deviations were also measured with the aid of statistical hypothesis testing [17].
Results interpretation and analysis
Optimal growth temperature of Saccharomyces cerevisiae (SUBSC01)
The optimal growth temperature for S. cerevisiae SUBSC01 was assessed through the measurement of OD600 and by counting the CFUs up to 450 min. After 90 min of incubation at 32.5 °C the cell number was found to increase rapidly by approximately 4 logs (Fig. 1), whereas at 30 °C such tendency was a bit slower (Fig. 1). Interestingly, compared with the growth state at 32.5 and 30 °C, a relatively lengthy lag phase (~270 min) was observed when cells were grown at 37 and 40 °C, possibly due to the requirement of longer time to cope with temperatures higher than the optimal growth condition (Fig. 1). Besides, under the light microscope, budding yeasts were observed after 90 min at 30 and 32.5 °C, whereas after 180 min such budding was observed at 37 °C. At 40 °C, the budding events were noticed after 270 min (Fig. 2). The optimal growth temperature for S. cerevisiae SUBSC01 was thus noted to be 32.5 °C. However, it is to be mentioned that earlier S. cerevisiae was found to exhibit optimal growth temperature between 25 and 35 °C as reported by the other groups [41–44].
Assessment of the optimum temperature through the examination of growth of Saccharomyces cerevisiae SUBSC01in terms of a Optical Density at 600 nm (OD600) and b the formation of the colony forming units (CFUs). Cells were grown as stated in “Methods”, and aliquots were removed at the specific time intervals (90, 180, 270, 360 and 450 min) for the assay. A prolonged lag phase (~270 min) was observed, when cells were grown at 30, 37, 40 °C. Consequently, the optimum growth temperature of the laboratory stain S. cerevisiae SUBSC01 was estimated to be 32.5 °C
Morphological study of S. cerevisiae SUBSC01 cells at optimal (32.5 °C) and high (40 °C) temperatures. Cells were grown as stated in “Methods”, and aliquots were removed at 90, 270 and 450 min for the assay. Active budding yeast was observed under light microscope at 32.5 °C up to 450 min of incubation. Besides, stressed cells (cells without the cytoplasmic contents) were observed at 40 °C after 450 min of incubation
Critical growth temperature of Saccharomyces cerevisiae (SUBSC01)
The ability of the yeast strain to grow at 40 °C led our interest further to examine the maximal growth temperature limit. While a sharp drop was observed in CFU and a relatively lower reduction in the cell turbidity was noticed at 44 and 45 °C after 180 and 450 min, respectively; notably an inclusive retardation of growth was also observed when cells were grown at 46 °C (Fig. 3). Interestingly, the budding yeasts were found to become dormant when cells were grown at 45 °C (Fig. 4d–f). In addition, all cells were found without the cytoplasmic contents at 46 °C (Fig. 4i).
Effect of high temperatures (44–46 °C) on growth of S. cerevisiae SUBSC01: a impact on cell turbidity and b influence on the formation of CFUs. Cells were grown as stated in “Methods”, and aliquots were removed at the specific time intervals as indicated. A complete decline of both OD600 and CFUs was noticed at 46 °C. The critical temperature of S. cerevisiae SUBSC01 was recorded at 45 °C
Observation of the morphological changes of S. cerevisiae SUBSC01 cells at 44 °C (a–c), 45 °C (d–f) and 46 °C (g–i). Cells were grown as stated in “Methods”, and aliquots were removed at the specific time intervals (90, 270 and 450 min). Interestingly, budding cells were found to be dormant at 45 °C (d–f). Also the cells were found to lose their cytoplasmic content when grown at 46 °C (i)
An important physiological point is to ponder that unlike Escherichia coli cells, the yeast cells grown at high temperature did not exhibit the characteristics of cell lysis, possibly due to the comparatively rigid cell membrane and cell wall [13, 16]. However, as has been seen in the current study, the generation of the cells without the cytoplasmic contents due to the deletion of rpoE gene (encoding the RNA polymerase σE) in the bacterium Escherichia coli W3110 has also been observed earlier through electron microscopy [16]. In the current study, such an observation of the yeasts cells lacking the cytoplasmic contents under the stressed condition has further drawn the interest on the global impact of heat shock on microorganisms at the cellular level, and led us further to cross-check the expected loss of the cell viability at high temperature by means of the spot dilution tests [17, 38]. In consistent with the results from the growth assessment, all yeast cells were found to lose the culturability completely at 46 °C as observed through the spot dilution tests (results not shown). Hence the critical growth temperature of this strain was recorded at 45 °C.
Growth retardation of S. cerevisiae SUBSC01 at critical temperature accompanied with an ascending osmotic shock
In order to achieve the complete stress response consequences of S. cerevisiae SUBSC01 upon critical temperature, different levels of osmotic pressure were simulated onward. A relatively lengthy lag phase (~360 min) was observed in both OD600 and CFU in compliance with the extended dextrose concentrations at 32.5 °C (Fig. 5a, d). Approximately 4 log CFU/ml was found to be abolished in 7X and 9X dextrose concentrations. Besides, even capable of growing at 45 °C, cells were found to lose their culturability completely at this temperature when the culture medium was supplemented with extremely high (9X) dextrose concentration (Fig. 5c, d). Earlier investigation also showed that yeast cells may exhibit an immediate growth arrest when exposed to an increase in external osmolarity [45]. The prolonged lag phase (~360 min) in the culturable cells (Fig. 5) led us to examine the probable morphological changes and impairments in the stressed yeast cells afterward. Interestingly the budding cells were found to become quiescent at the optimal temperature with 5X dextrose concentration (Fig. 6g–i), and additionally cells were also found to be thickened (Fig. 6o) at 9X dextrose concentration (Fig. 6m–o). In cohesion to the current findings, previously, the cell volume of S. cerevisiae was also found to expand at 48 h of incubation periods upon osmotic stress [46]. However, a huge number of cells loosing the cytoplasmic contents were observed at 45 °C with extreme high (9X) dextrose concentration (Fig. 6m′–o′). Consistently, in the absence of osmotic imbalances no stressed cells (cells without the cytoplasmic contents) were observed at 45 °C.
Growth retardation of S. cerevisiae SUBSC01 at 32.5 and 45 °C with different dextrose concentrations. Cell culturability was assessed through OD600 and the formation of CFUs at 32.5 °C (a, b), and at 45 °C (c, d) with different dextrose concentrations, i.e., 0.04 g/l (1X), 0.12 g/l (3X), 0.2 g/l (5X), 0.28 g/l (7X), 0.36 g/l (9X). Cells were grown as stated in “Methods”, and aliquots were removed at the specific time intervals of 90, 180, 270, 360 and 450 min. A complete elimination of culturable cells were observed at 45 °C with 7X and 9X dextrose concentrations (c, d)
Morphological study of S. cerevisiae SUBSC01 at 32.5 °C (a–o) and 45 °C (a’–o’) upon osmotic stresses. Cells were grown as stated in “Methods”, aliquots were removed at 90, 270 and 450 min. Budding cells became dormant at 32.5 °C with 5X (0.14 g/l) dextrose concentration (g–i), and were found to be thickened at 9X dextrose concentration (o). All cells lost cytoplasmic contents at 45 °C with 9X (0.18 g/l) dextrose concentrations (m–o)
As stated earlier, S. cerevisiae SUBSC01 exhibits approximately 360 min long lag phase at 32 °C and complete growth suppression at 45 °C due to osmotic shock. Elimination of such growth was further supported by the appearance of the stressed cells as seen under the microscope (Fig. 6m′–o′). Such results led us to further cross check the stressed physiology of the cells through spot test. At 32.5 °C in different dextrose concentrations (1X–9X), cells were found culturable through spot dilution tests (results not shown). At 45 °C under high (9X) dextrose concentration, cells were found to lose their culturability completely. Earlier research found that the activity of β-fructofuranosidase (SUC2) of S. cerevisiae, which is liable for sucrose degradation; might be repressed by the increased osmotic pressure [28, 32]. This is to be mentioned that when the cells were grown at 32.5 °C in different sucrose concentrations, all were found to grow after a certain incubation period (results not shown). Nevertheless, the current investigation clearly unraveled the heat stress responsive events in S. cerevisiae SUBSC01, which is comprehensible with the existing knowledge on yeast growth phases and stress physiology.
The revelation of the temperature tolerance of yeast cells as revealed from the current study is consistent with the recent findings [15, 21, 47]. Indeed, deviation in temperature is a general stress encountered by yeast cells [47]. S. cerevisiae is well known to generate the protective transcriptional programs in response to elevated temperatures [15, 21]. However, those studies mostly showed the temperature tolerance at around 37 °C while the current study clearly showed that the yeast strain studied here could withstand up to 45 °C. Besides, the findings of the critical growth temperature besides the optimal condition, sugar tolerance level, and a bit interestingly the observation of prolonged lag phase at high temperatures may be of significance in the field of yeast physiology. Presented results may provide further general information on the triggering phase of heat shock events in yeast cells. Further studies regarding the expressional analyses of the stress responsive genes would unveil the involvement of the necessary regulons and chaperons required for the stress defense mechanism in the S. cerevisiae SUBSC01.
References
Brewster JL, de Valoir T, Dwyer ND, Winter E, Gustin MC. An osmosensing signal transduction pathway in yeast. Science. 1993;259:1760–3.
Maeda T, Wurgler-Murphy SM, Saito H. A two-component system that regulates an osmosensing MAP kinase cascade in yeast. Nature. 1994;369:242–5.
Lewis JG, Learmonth RP, Watson K. Induction of heat, freezing and salt tolerance by heat and salt shock in Saccharomyces cerevisiae. Microbiology. 1995;141:687–94.
Turkel S. Effects of various physiological stresses on transcription of the SUC2 gene in the yeast Saccharomyces cerevisiae. Turk J Biol. 2000;24:233–40.
Nitta T, Nagamitsu H, Murata M, Izu H, Yamada M. Function of the σE regulon in dead-cell lysis in stationary phase Escherichia coli. J Bacteriol. 2000;182:5231–7.
Collinet B, Yuzawa H, Chen T, Herrera C, Missiakas D. RseB binding to the periplasmic domain of RseA modulates the RseA: σE interaction in the cytoplasm and the availability of σE RNA polymerase. J Biol Chem. 2000;275:33898–904.
Erasmus DJ, van der Merwe GK, van Vuuren HJJ. Genome-wide expression analyses: metabolic adaptation of Saccharomyces cerevisiae to high sugar stress. FEMS Yeast Res. 2003;3:375–99.
Kabir MS, Yamashita D, Noor R, Yamada M. Effect of σS on σE -directed cell Lysis in Escherichia coli Early stationary phase. J Mol Microbiol Biotechnol. 2004;8:189–94.
Yamamoto A, Mizukami Y, Sakurai H. Identification of a novel class of target genes and a novel type of binding sequence of heat shock transcription factor in Saccharomyces cerevisiae. J Biol Chem. 2005;280:11911–9.
Bond U. Stressed out! Effects of environmental stress on mRNA metabolism. FEMS Yeast Res. 2006;6:160–70.
Yamamoto N, Maeda Y, Ikeda A, Sakurai H. Regulation of thermotolerance by stress-induced transcription factors in Saccharomyces cerevisiae. Eukaryot Cell. 2008;7:783–90.
Yamada M, Noor R, Nagamitsu H, Murta M. The higher temperature, the more oxidative stress and lysis in Escherichia coli. The 3rd International Conference on Fermentation Technology for Value Added Agricultural Products: Khon Kaen. 2009.
Noor R, Murata M, Nagamitsu H, Klein G, Rain S, Yamada M. Dissection of σE dependent cell lysis in Escherichia coli: roles of RpoE regulators RseA, RseB and periplasmic folding catalyst Ppid. Genes Cells. 2009;14:885–99.
Noor R, Murata M, Yamada M. Oxidative stress as a trigger for growth phase-specific σE dependent cell lysis in Escherichia coli. J Mol Microbiol Biotechnol. 2009;17:177–87.
Morano KA, Grant CM, Moye-Rowley WS. The response to heat shock and oxidative stress in Saccharomyces cerevisiae. Genetics. 2012;190:1157–95.
Murata M, Noor R, Nagamitsu H, Tanaka S, Yamada M. Novel pathway directed by σE to cause cell lysis in Escherichia coli. Genes Cells. 2012;17:234–47.
Nur IT, Munna MS, Noor R. Study of exogenous oxidative stress response in Escherichia coli, Pseudomonas spp., Bacillus spp. and Salmonella spp. Turk J Biol. 2014;38:502–9.
Tamari Z, Rosin D, Voichek Y, Barkai N. Coordination of gene expression and growth-rate in natural populations of budding yeast. PLoS ONE. 2014;9:e88801.
Sanchez Y, Lindquist SL. HSP104 required for induced thermotolerance. Science. 1990;248:1112–5.
Virgiliol CD, Pipes P, Boll T, Wiemkenl A. Acquisition of thermotolerance in Saccharomyces cerevisiae without heat shock protein hsp104 and in the absence of protein synthesis. FEBS Lett. 1991;288:86–90.
Verghese J, Abrams J, Wang Y, Morano KA. Biology of the heat shock response and protein chaperones: budding yeast (Saccharomyces cerevisiae) as a model system. Microbiol Mol Biol Rev. 2012;76:115–58.
Raina S, Missiakas D, Georgopoulos C. The rpoE gene encoding the σE (sigma 24) heat-shock sigma factor of Escherichia coli. EMBO J. 1995;14:1043–55.
Missiakas D, Mayer MP, Lemaire M, Georgopoulos C, Rania S. Modulation of the Escherichia coli σE (RpoE) heat-shock transcription factor activity by the RseA, RseB and RseC proteins. Mol Microbiol. 1997;24:355–71.
Glover JR, Lindquist S. Hsp104, Hsp70, and Hsp40: a novel chaperone system that rescues previously aggregated proteins. Cell. 1998;94:73–82.
Ishihama A. Modulation of the nucleoid, the transcription apparatus, and the translation machinery in bacteria for stationary phase survival. Genes Cells. 1999;4:135–43.
Ades SE. Control of the alternative sigma factor σE in Escherichia coli. Curr Opin Microbiol. 2004;7:157–62.
Morimoto RI. The heat shock response: systems biology of proteotoxic stress in aging and disease. Cold Spring Harb Symp Quant Biol. 2012;76:91–9.
Blomberg A. The osmotic hypersensitivity of the yeast Saccharomyces cerevisiae is strain and growth media dependent: quantitative aspects of the phenomenon. Yeast. 1997;13:529–39.
Yamamoto A, Ueda J, Yamamoto N, Hashikawa N, Sakurai H. Role of heat shock transcription factor in Saccharomyces cerevisiae oxidative stress response. Eukaryot Cell. 2007;6:1373–9.
Moraitis C, Curran BPG. Reactive oxygen species may influence the heat shock response and stress tolerance in the yeast Saccharomyces cerevisiae. Yeast. 2004;21:313–23.
Parsell DA, Kowal AS, Singer MA, Lindquist S. Protein disaggregation mediated by heat-shock protein Hsp104. Nature. 1994;372:475–8.
Hampsey M. A review of phenotypes in Saccharomyces cerevisiae. Yeast. 1997;13:1099–133.
Liu CL, Ge P, Zhang F, Hu BR. Co-translational protein aggregation after transient cerebral ischemia. Neuroscience. 2005;134:1273–84.
Gibney PA, Lu C, Caudya AA, Hess DC, Botstein D. Yeast metabolic and signaling genes are required for heat-shock survival and have little overlap with the heat-induced genes. PNAS. 2013; E4393–E4402.
Rowley A, Johnston GC, Butler B, Werner-Washburne M, Singer RA. Heat shock-mediated cell cycle blockage and G1 cyclin expression in the yeast Saccharomyces cerevisiae. Mol Cell Biol. 1993;13:1034–41.
Noor R, Islam Z, Munshi SK, Rahman F. Influence of temperature on Escherichia coli growth in different culture media. J Pure Appl Microbiol. 2013;7:899–904.
Munna MS, Nur IT, Rahman T, Noor R. Influence of exogenous oxidative stress on Escherichia coli cell growth, viability and morphology. Am J Biosci. 2013;1:59–62.
Munna MS, Tamanna S, Afrin MR, Sharif GA, Mazumder C, Kana KS, Urmi NJ, Uddin MA, Rahman T, Noor R. Influence of aeration speed on bacterial colony forming unit (CFU) formation capacity. Am J Microbiol Res. 2014;2:47–51.
Cappuccino JG, Shermen N. Microbiology; Laboratory manuals. San Francisco: Benjamin/Cummings Publishing Company Incorporated; 1996.
McKenzie CGJ, Koser U, Lewis LE, Bain JM, Mora-Montes HM, Barker RN, Gow NAR, Erwig LP. Contribution of Candida albicans cell wall components to recognition by and escape from murine macrophages. Infect Immun. 2010;78:1650–8.
Walsh RM, Martin PA. Growth of Saccharomyces cerevisiae and S Saccharomyces uvarum in a temperature gradient incubator. J Inst Brew. 1977;83:169–72.
Tai LS, Daran-Lapujade P, Walsh MC, Pron JT, Daran JM. Acclimation of Saccharomyces cerevisiae to low temperature: a chemostat-based transcriptome analysis. Mol Biol Cell. 2007;18:5100–12.
Salvado Z, Arroyo-Lopez FN, Guillamon JM, Salazar G, Querol A, Barrio E. Temperature adaptation markedly determines the growth and evolution within the genus Saccharomyces. Appl Environ Microbiol. 2011;77:2292–302.
Lopez-Malo M, Querol A, Guillamon JM. Metabolomic comparison of Saccharomyces cerevisiae and the cryotolerant species S. bayanus var. uvarum and S. kudriavzevii during wine fermentation at low temperature. PLoS ONE. 2013;8:e60135.
Wuytswinkel OV, Reiser V, Siderius M, Kelders MC, Ammerer G, Ruis H, Mager WH. Response of Saccharomyces cerevisiae to severe osmotic stress: evidence for a novel activation mechanism of the HOG MAP kinase pathway. Mol Microbiol. 2000;37:382–97.
Pratt PL, Bryce JH, Stewart GG. The effects of osmotic pressure and ethanol on yeast viability and morphology. J Inst Brew. 2003;109:218–28.
Kanshin E, Kubiniok P, Thattikota Y, D’Amours D, Thibault P. Phosphoproteome dynamics of Saccharomyces cerevisiae under heat shock and cold stress. Mol Syst Biol. 2015;11(6):813.
Authors’ contributions
This work was carried out in collaboration between all authors. MSM managed the literature searches and wrote the first draft, SH performed the experiments, and RN designed the study, analyzed the results and revised the manuscript. All authors read and approved the final manuscript.
Authors’ information
All the authors are from Department of Microbiology, Stamford University Bangladesh. Authors MSM and SH are the thesis students of MS program. The corresponding author RN is working as Associate Professor and Chairman.
Acknowledgements
Authors thank Stamford University Bangladesh for the logistic supports. However, the authors received no specific funding for this work.
Compliance with ethical guidelines
Competing interests The authors declare that they have no conflict of interest.
Author information
Authors and Affiliations
Corresponding author
Rights and permissions
Open Access This article is distributed under the terms of the Creative Commons Attribution 4.0 International License (http://creativecommons.org/licenses/by/4.0/), which permits unrestricted use, distribution, and reproduction in any medium, provided you give appropriate credit to the original author(s) and the source, provide a link to the Creative Commons license, and indicate if changes were made. The Creative Commons Public Domain Dedication waiver (http://creativecommons.org/publicdomain/zero/1.0/) applies to the data made available in this article, unless otherwise stated.
About this article
Cite this article
Munna, M.S., Humayun, S. & Noor, R. Influence of heat shock and osmotic stresses on the growth and viability of Saccharomyces cerevisiae SUBSC01. BMC Res Notes 8, 369 (2015). https://doi.org/10.1186/s13104-015-1355-x
Received:
Accepted:
Published:
DOI: https://doi.org/10.1186/s13104-015-1355-x