Abstract
CuO-CeO2 nanocomposite is reported as an efficient and green recyclable catalyst for the synthesis of chromene derivatives under solvent-free conditions. The catalyst was synthesized by co-precipitation method and characterized by X-ray diffraction, Brenauer-Emmett-Teller specific surface area, FESEM and energy dispersive spectroscopy analysis. This catalyst can be recovered by simple filtration and recycled up to 10 consecutive runs without loss of its activity. This process provides some advantages such as simple work-up, clean procedure, short reaction times and high yields of the products.
Similar content being viewed by others
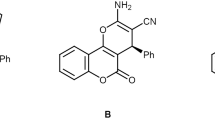
Background
In recent years, development of new catalysts by nanoscale design has emerged as a fertile field for research and innovation [1]. The ability of the nanotechnology to enhance catalytic activity opens the potential to replace expensive catalysts with lower amounts of inexpensive nanocatalysts [2]. In particular, nanocrystalline oxides have proved to be useful to chemists in the laboratory and industry due to the good activation of adsorbed compounds and reaction rate enhancement, selectivity, easier work-up, recyclability of the supports and the eco-friendly reaction conditions [3–7]. Also the practical applications of nanocomposite metal oxides as the catalysts in organic synthesis have been increased due to their high catalytic activity because of the high surface area [8, 9].
Aminochromenes are important class of compounds that received significant attention from many pharmaceutical and organic chemists because of the broad spectrum of their biological and pharmaceutical properties such as antisterility and anticancer agents [10]. Furthermore, these compounds are the main constituents of many natural products and used as cosmetics, pigments, and potential biodegradable agrochemicals [11]. Therefore, the synthesis of aminochromenes is very important in organic chemistry. The most important synthetic procedure for the synthesis of these compounds involves the multi-component condensation of aldehyde, malononitrile and phenol derivatives. Although various methods have been reported for the synthesis of aminochromenes [12–27], however, some of these methods involve the use of expensive reagents, toxic solvents, tedious workup, long reaction times and harsh reaction conditions. Therefore, introduction of new methods and catalysts for the synthesis of these important compounds in terms of potential simplicity, high activity, low cost, high yields and short reaction times are still in demand.
Recently, we have reported the preparation of CuO-CeO2 nanocomposite and its catalytic activity for the synthesis of aryl-14H-dibenzo[a-j]xanthenes and benzopyran derivatives [28, 29]. This catalyst is safe, easy to handle and environmentally benign. In continuation of these studies and our studies on the synthesis of benzochromene derivatives [30], herein, we wish to report the applicability of CuO-CeO2 nanocomposite as a green, efficient and recyclable catalyst for the synthesis of aminochromenes via the one-pot three-component reaction of resorcinol, aromatic aldehydes and malononitrile under solvent-free conditions (Scheme 1).
Results and discussion
The CuO-CeO2 nanocomposite oxide catalyst was prepared by a coprecipitation method. BET specific surface area, emission scanning electron microscopy (ESEM) (Figure 1), X-ray diffraction (XRD) (Figure 2), and energy dispersive spectroscopy (EDS) (Figure 3), were used to characterize the catalyst [28].
At first, for the optimization of the reaction conditions, a mixture of resorcinol, benzaldehyde and malononitrile was investigated as a model and its behavior was studied under a variety of conditions. The best result was achieved by carrying out the reaction of resorcinol, benzaldehyde and malononitrile (with 1:1:1 mol ratio) in the presence of 0.05 g of CuO-CeO2 nanocomposite at 80°C under solvent-free conditions (Table 1, entry 1). Catalytic activity results reveal that the CuO-CeO2 nanocomposite exhibited efficient activity during the synthesis of aminochromenes. Under the optimized conditions, the reaction of a variety of differently substituted aromatic aldehydes, containing electron-donating and electron-withdrawing groups, was explored. In all cases, aromatic aldehydes reacted successfully and desired products achieved in good to high yields. It can be found that the kind of group on aromatic ring has no significant effect on the reaction. Aliphatic aldehydes stay intact under the similar reaction conditions. Therefore, this method can be useful for the chemoselective synthesis of aminochromene derivatives from aromatic aldehydes in the presence of aliphatic ones. All reactions were performed under solvent-free and entirely heterogeneous reaction conditions. Workup procedure is so simple and all products were cleanly isolated by simple filtration and evaporation of the solvent. Another advantage of this process is that CuO-CeO2 nanocomposite is safe, easy to handle and its preparation is simple. Furthermore, very low amount of the catalyst is needed and it can be recovered simply by filtration. Products were easily recrystallized from hot ethanol and were obtained during the short reaction times. Furthermore, our procedure is environmentally friendly as it does not use any toxic auxiliary or solvent. A distinct characterization of this method, illustrated in this work is the synthesis of corresponding products without by-product.
The recyclability of the catalyst is also important. To investigate of this property, the reaction of resorcinol with benzaldehyde and malononitrile was selected again as a model (Table 2). After completion of the reaction, the recovered catalyst was washed with hot ethanol and after dryness was reused in the next similar run. This procedure was repeated for eight consecutive runs and the desired product was obtained in good to high yields after one to eight runs.
Conclusion
In conclusion, we have described a highly efficient method for the synthesis of aminochromenes catalyzed by CuO-CeO2 nanocomposite as a green recyclable catalyst under solvent-free conditions. This catalytic system is stable and can promote the yields and reaction times over eight runs without loss of its activity. Moreover, simple work-up, high yields of products, short reaction times, heterogeneous nature of the reaction conditions, and clean procedure, will make this procedure a useful addition to the available methods.
Methods
General
Chemicals were purchased from Merck Chemical company (Whitehouse Station, NJ, USA). All the NMR spectra were recorded on a Bruker Advance 400 MHz. Melting points were recorded on a Barnstead Electrothermal 9100BZ melting point apparatus (St. Louis, MO, USA). Yields refer to isolated pure products.
Catalyst preparation
CuO-CeO2 nanocomposite was prepared by co-precipitation method using aqueous solution of cerium and copper nitrates and drop-wise KOH as precipitant agent under vigorous mixing while temperature and pH was fixed at unique values. Then acquired sample was filtered, washed and calcined to obtain final catalyst to be used in the synthesis of aminochromenes.
Catalyst characterization
The catalyst structure characterization was performed by XRD, using a Bruker AXS D8 advanced diffractometer equipment (Madison, WI, USA) with CuKα radiation (λ = 1.5406 Å). The Debye-Scherrer equation is used to determine average crystallite size of nanoparticles. ESEM and EDS carried out by a Hitachi S4160 instrument to see the morphology, evaluation of cluster size and metal composition of the catalyst. BET specific surface area was estimated from the N2 adsorption/desorption isotherms, measured at 77 K using a Quantachrome CHEMBET-3000 instrument (Boynton Beach, FL, USA). The infrared (IR) spectra were recorded on a PerkinElmer 781 spectrophotometer (Waltham, MA, USA).
General procedure
A mixture of aromatic aldehyde (1 mmol), malononitrile (1 mmol), resorcinol (1 mmol) and CuO-CeO2 nanocomposite (0.05 g) was heated in an oil bath (80°C) for the appropriate times (Table 1). After completion of the reaction as followed by TLC, the reaction mixture was cooled to room temperature and hot ethanol (10 ml) was added and the catalyst was filtered. Evaporation of the solvent from the filtrate and recrystallization of the solid residue from hot ethanol afforded the pure products in good to high yields. The spectral and analytical data for the new compounds are as follow (see Additional file 1):
-
Table 1, entry 12: White solid, MP: 109°C to 111°C; IR (KBr) νmax/cm-1: 3,434, 3,340, 3,201, 2,808, 2,231, 2,185, 1,648, 1,505, 1,406, 1,306, 1,237, 1,158, 1,107, 1,050. Proton Nuclear Magnetic Resonance (1H NMR) (CDCl3, 400 MHz), δ (ppm): 4.70 (s, 1H, CH), 5.22 (s, 2H, NH2), 6.53 to 6.58 (m, 2H, Ar), 6.67 (d, 1H, J = 8.36 Hz), 7.28 (d, 2H, J = 8.16 Hz, Ar), 7.58 (d, 2H, J = 8.20 Hz, Ar), 9.07 (s, 1H, OH). 13C NMR (CDCl3, 100 MHz): 39.36 (CH), 57.21 (CN), 102.03, 109.45, 110.78, 112.03, 117.66, 118.98, 127.48, 128.66, 131.40, 147.91, 149.39, 156.33, 158.74. Elem. Anal. Found: C, 70.71%; H, 3.89% N, 14.61% (calculated for C17H11N3O2: C, 70.58%; H, 3.83%; N, 14.52%).
-
Table 1, entry 13: Yellow solid, MP: 257°C to 259°C; IR (KBr) νmax/cm-1: 3,476, 3,338, 3,271, 2,191, 1,641, 1,586, 1,504, 1,463, 1,409, 1,341, 1,312, 1,151, 1,105, 1,049, 854, 807. 1H NMR (DMSO-d6, 400 MHz), δ (ppm): 5.11 (s, 1H, CH), 6.39 (s, 1H, Ar), 6.45 to 6.48 (m, 1H, Ar), 6.69 (d, 1H, J = 8.8 Hz, Ar), 6.97 (s, 2H, NH2), 7.18 (d, 1H, J = 8.4 Hz, Ar), 7.38 (d, 1H, J = 8.4, Ar), 7.57 (s, 1H, Ar), 9.76 (s, 1H, OH). 13C NMR (DMSO-d6, 100 MHz): 37.35 (CH), 54.90 (CN), 102.75, 112.36, 113.05, 120.61, 128.51, 129.55, 129.72, 132.62, 132.69, 133.27, 142.37, 149.48, 157.94, 160.96. Elem. Anal. Found: C, 57.74%; H, 3.08% N, 8.47% (calculated for C16H15N2O2Cl2: C, 57.68%; H, 3.02%; N, 8.40%).
-
Table 1, entry 14: Orange solid, MP: 216°C to 218°C; IR (KBr) νmax/cm-1: 3,476, 3,337, 3,270, 2,961, 2,190, 1,639, 1,587, 1,504, 1,463, 1,409, 1,341, 1,311, 1,246, 1,153, 1,105, 1,049, 963, 854, 807. 1H NMR (DMSO-d6, 400 MHz), δ (ppm): 1.13 (d, 6H, 2CH3), 2.77 to 282 (m, 1H, CH), 4.56 (s, 1H, CH), 6.822 (m, 3H, Ar), 7.05 (s, 2H, NH2), 7.13 to 7.15 (m, 2H, Ar), 9.69 (s, 1H, OH). 13C NMR (DMSO-d6, 100 MHz): 25.47 (CH3), 31.09 (CH), 34.61 (CH), 57.97 (CN), 103.75, 113.95, 115.56, 122.37, 128.08, 128.79, 131.50, 145.44, 148.20, 150.45, 158.61, 161.85. Elem. Anal. Found: C, 74.48%; H, 5.97% N, 9.24% (calculated for C19H18N2O2: C, 74.39%; H, 5.92%; N, 9.18%).
The spectral and analytical data for the known compounds are as follow (see Additional file 2):
-
Table 1, entry 1: IR (KBr) νmax/cm-1: 3,450, 3,300, 3,200, 2,200, 1,650, 1,580,1,510, 1,480, 1,380, 1,120, 1,060, 760, 700. 1H NMR (CDCl3, 400 MHz), δ (ppm): 4.61 (s, 1H, CH), 5.47 (s, 2H, NH2), 6.52 (t, 2H, J = 8.88 Hz,Ar), 6.73 (d, 2H, J = 8.28 Hz,Ar), 7.17 to 7.30 (m, 5H, Ar), 9.17 (s, 1H, OH). 13C NMR (CDCl3, 100 MHz): 38.74, 57.55, 101.39, 111.31, 112.01, 119.23, 125.36, 126.30, 127.09, 128.46, 144.07, 147.65, 155.64, 158.42.
-
Table 1, entry 2: IR (KBr) νmax/cm-1: 3,450, 3,300, 3,200, 2,200, 1,650, 1,580, 1,510, 1,480, 1,380, 1,120, 1,060, 760, 690. 1H NMR (DMSO-d6, 400 MHz), δ (ppm): 4.64 (s, 1H, CH), 6.38 (s, 1H,Ar), 6.45 (d, 1H, J = 8.00 Hz,Ar), 6.75 (d, 1H, J = 8.4 Hz, Ar), 6.90 (s, 2H, NH2), 7.15 (d, 2H, J = 8.00 Hz, Ar), 7.33 (d, 2H, J = 8.4 Hz, Ar), 9.71 (s, 1H, OH). 13C NMR (DMSO-d6, 100 MHz): 38.41, 56.30, 102.67, 112.92, 113.64, 120.95, 129.67, 129.01, 129.73, 130.34, 131.67, 145.77, 149.27, 157.66, 160.69.
-
Table 1, entry 5: IR (KBr) νmax/cm-1: 3,400, 3,300, 3,200, 3,050, 2,200, 1,650, 1,580, 1,520, 1,380, 1,360, 1,120, 1,080, 760, 680. 1H NMR (CDCl3, 400 MHz), δ (ppm): 4.79 (s, 1H, CH), 5.61 (s, 2H, NH2), 6.55 to 6.58 (m, 2H, Ar), 6.70 (d, 1H, J = 8.20 Hz, Ar), 7.50 (t, 1H, J = 7.92, Ar), 7.58 (d, 1H, J = 7.63 Hz), 8.01 (s, 1H, Ar), 8.07 (d, 1H, J = 8.08 Hz, Ar), 9.25 (s, 1H, OH). 13C NMR (CDCl3, 100 MHz): 38.00 (CH), 58.95 (CN), 101.86, 110.58, 111.81, 118.90, 120.68, 121.29, 128.31, 128.44, 132.86, 146.33, 147.04, 147.76, 156.29, 158.78, 169.72.
-
Table 1, entry 6: 3,450, 3,300, 3,200, 2,200, 1,650, 1,580, 1,510, 1,480, 1,380, 1,120, 1,060, 750. 1H NMR (CDCl3, 400 MHz), δ (ppm): 4.60 (s, 1H, CH), 5.34 (s, 2H, NH2), 6.54 (t, 2H, J = 8.44 Hz, Ar), 6.70 (d, 1H, J = 8.36 Hz,Ar), 7.06 (d, 2H, J = 8.2 Hz, Ar), 7.40 (2H, J = 8.2 Hz, Ar), 9.20 (s, 1H, OH). 13C NMR (CDCl3, 100 MHz): 38.65, 57.56, 101.70, 111.50, 111.67, 119.23, 119.41, 128.35, 128.62, 130.37, 143.22, 147.74, 155.96, 158.49.
-
Table 1, entry 7: 3,450, 3,300, 3,200, 2,200, 1,650, 1,600, 1,510, 1,380, 1,320, 1,120, 1,060, 760. 1H NMR (DMSO-d6, 400 MHz), δ (ppm): 4.63 (s, 1H, CH), 6.37 (s, 1H,Ar), 6.45 (d, 1H, J = 8.00 Hz, Ar), 6.74 (d, 1H, J = 8.4 Hz, Ar), 6.87 (s, 2H, NH2), 7.10 (d, 2H, J = 8.4 Hz, Ar), 7.16 (s, 3H, Ar), 9.70 (s, 1H, OH). 13C NMR (DMSO-d6, 100 MHz): 39.34, 56.63, 102.66, 112.91, 114.00, 115.66, 115.87, 121.04, 129.67, 129.75, 130.37, 143.02, 149.27, 157.61.
References
Banerjee S, Sereda G: One-step, three-component synthesis of highly substituted pyridines using silica nanoparticle as reusable catalyst. Tetrahedron Lett 2009, 50: 6959–6992. 10.1016/j.tetlet.2009.09.137
Astruc D: Nanoparticles and catalysis. Weinheim: Wiley-VCH; 2008.
Sharghi H, Hosseini M: Solvent-free and one step Beckman rearrangement of Ketones and aldehydes by zinc oxide. Synthesis 2002, 1057–1059.
Hosseini SM, Sharghi H: Reactions on a solid surface. A simple, economical and efficient Friedel-Crafts acylation reaction over zinc oxide (ZnO) as a new catalyst. J. Org. Chem 2004, 69: 6953–6956. 10.1021/jo0494477
Yin SF, Xu BQ, Wang SJ, Ng CF, Au CT: Magnesia-carbon nanotubes (MgO–CNTs) nanocomposite: novel support of Ru catalyst for the generation of C Ox -free hydrogen from ammonia. Catal. Lett 2004, 96: 113–116.
Drexler MT, Amiridis MD: Kinetic investigation of the heterogeneous synthesis of flavanone over MgO. Catal. Lett 2002, 79: 175–181. 10.1023/A:1015320711566
Varma RS: Solvent-free organic syntheses, using supported reagents and microwave irradiation. Green Chem 1999, 1: 43–55. 10.1039/a808223e
Samantaray S, Pradhan DK, Hota G, Mishra BG: Catalytic application of CeO 2 -CaO nanocomposite oxide synthesized using amorphous citrate process toward the aqueous phase one pot synthesis of 2-amino-2-chromenes. Chem. Eng. J 2012, 193: 1–9.
Li S, Zhang W, So MH, Che CM, Wang R, Chen R: One-pot solvothermal synthesis of Pd/Fe 3 O 4 nanocomposite and its magnetically recyclable and efficient catalysis for Suzuki reactions. J Mol Catal A Chem 2012, 359: 81–87.
Kidwai M, Saxena S, Khan MRK, Thukral SS: Aqua mediated synthesis of substituted 2-amino-4 H -chromenes and in vitro study as antibacterial agents. Bioorg. Med. Chem. Lett 2005, 15: 4295–4298. 10.1016/j.bmcl.2005.06.041
Dekamin MG, Eslami M, Maleki A: Potassium phthalimide-N-oxyl: a novel, efficient, and simple organocatalyst for the one-pot three-component synthesis of various 2-amino-4 H -chromene derivatives in water. Tetrahedron 2013, 69: 1074–1085. 10.1016/j.tet.2012.11.068
Naimi-Jamal MR, Mashkouri S, Sharifi A: An efficient, multicomponent approach for solvent-free synthesis of 2-amino-4 H -chromene scaffold. Mol. Divers 2010, 14: 437–477.
Khurana JM, Nand B, Saluja P: DBU: a highly efficient catalyst for one-pot synthesis of substituted 3,4-dihydropyrano[3,2-c]chromenes, dihydropyrano[4,3-b]pyranes, 2-amino-4Hbenzo[h]chromenes and 2-amino-4H benzo[g]chromenes in aqueous medium. Tetrahedron 2010, 66: 5637–5641. 10.1016/j.tet.2010.05.082
Jin TS, Xiao JC, Wang SJ, Li TS: Ultrasound-assisted synthesis of 2-amino-2-chromenes with cetyltrimethylammonium bromide in aqueous media. Ultrason. Sonochem 2004, 11: 393–397.
Makarem S, Mohammadi AA, Fakhri AR: A multi-component electro-organic synthesis of 2-amino-4H-chromenes. Tetrahedron Lett 2008, 49: 7194–7196. 10.1016/j.tetlet.2008.10.006
Dyachenko VD, Chernegam AN: Aliphatic aldehydes in multicomponent syntheses of 4-alkyl-substituted partially hydrogenated quinolines, fused 4H-pyrans, and 2-amino-4-ethyl-5-methylbenzene-1,3-dicarbonitrile. Russ J Org Chem 2006, 42: 567–576. 10.1134/S1070428006040142
Mehrabi H, Kazemi-Mireki M: An efficient and recyclable nanocatalyst for the rapid and green synthesis of 3,4-dihydropyrano[c]chromenes. Chin. Chem. Lett 2011, 22: 1419–1422. 10.1016/j.cclet.2011.06.003
Maggi R, Ballini R, Sartori G, Sartorio R: Basic alumina catalysed synthesis of substituted 2-amino-2-chromenes via three-component reaction. Tetrahedron Lett 2004, 45: 2297–2299. 10.1016/j.tetlet.2004.01.115
Shestopalov AM, Emelianova YM, Nesterov VN: One_step synthesis of substituted 2-amino-4 H -chromenes and 2-amino-4 H -benzo[ f ]chromenes. Molecular and crystal structure of 2-amino-3-cyano-6-hydroxy-4-phenyl-4 H -benzo[ f ]chromene. Russ Chem Bull Int Ed 2002, 51: 2238–2241. 10.1023/A:1022135402451
Banothu J, Bavanthula R: Brønsted acidic ionic liquid catalyzed highly efficient synthesis of chromeno pyrimidinone derivatives and their antimicrobial activity. Chin. Chem. Lett 2012, 23: 1015–1018. 10.1016/j.cclet.2012.06.041
Mohammadzadeh I, Sheibani H: A convenient one-pot synthesis of new chromeno[3,4-c]chromene and chromeno[3,4-c]pyridine derivatives in the presence of high surface area of magnesium oxide. Chin. Chem. Lett 2012, 23: 1327–1330. 10.1016/j.cclet.2012.10.007
Mobinikhaledi A, Moghanian H, Sasani F: Microwave-assisted one-pot synthesis of 2-amino-2-chromenes using piperazine as a catalyst under solvent-free conditions. Synth React Inorg Met-Org Nano-Met Chem 2011, 41: 262–265.
Eshghi H, Damavandi S, Zohouri GH: Efficient one-pot synthesis of 2-amino-4 H- chromenes catalyzed by ferric hydrogen sulfate and Zr-based catalysts of FI. Synth React Inorg Met-Org Nano-Met Chem 2011, 41: 1067–1073. 10.1080/15533174.2011.591347
Raghuvanshi DS, Singh KN: An expeditious synthesis of novel pyranopyridine derivatives involving chromenes under controlled microwave irradiation. Arkivoc 2010, 10: 305–317.
Mosadegh E: Ultrasonic-assisted preparation of nano eggshell powder: a novel catalyst in green and high efficient synthesis of 2-aminochromenes. Ultrason. Sonochem 2013, 20: 1436–1441. 10.1016/j.ultsonch.2013.04.008
Gaikwad DS, Undale KA, Shaikh TS, Pore DM: An efficient multi-component synthesis of (2-amino-3-cyano-4 H -chromen-4-yl) phosphonic acid diethyl ester. C R Chim 2011, 14: 865–868. 10.1016/j.crci.2011.03.001
Datta B, Pasha MA: Glycine catalyzed convenient synthesis of 2-amino-4 H -chromenes in aqueous medium under sonic condition. Ultrason. Sonochem 2012, 19: 725–728. 10.1016/j.ultsonch.2012.01.006
Albadi J, Razeghi A, Mansournezhad A, Abbaszadeh H: CuO-CeO 2 nanocomposite: an efficient recyclable catalyst for the synthesis of aryl-14 H -dibenzo[a-j]xanthenes. J Nanoparticles 2013, 2013: 1–8.
Albadi J, Mansournezhad A, Derakhshandeh Z: CuO–CeO 2 nanocomposite: a highly efficient recyclable catalyst for the multicomponent synthesis of 4H-benzo[b]pyran derivatives. Chin. Chem. Lett 2013, 24: 821–824. 10.1016/j.cclet.2013.05.031
Albadi J, Mansournezhad A, Darvishi-Paduk M: Poly(4-vinylpyridine): as a green, efficient and commercial available basic catalyst for the synthesis of chromene derivatives. Chin. Chem. Lett 2013, 24: 208–210. 10.1016/j.cclet.2013.01.020
Acknowledgements
We are thankful to the Behbahan Khatam Alanbia University of Technology for the partial support of this work.
Author information
Authors and Affiliations
Corresponding author
Additional information
Competing interests
The authors declare that they have no competing interests.
Authors’ original submitted files for images
Below are the links to the authors’ original submitted files for images.
Rights and permissions
Open Access This article is distributed under the terms of the Creative Commons Attribution 2.0 International License (https://creativecommons.org/licenses/by/2.0), which permits unrestricted use, distribution, and reproduction in any medium, provided the original work is properly cited.
About this article
Cite this article
Albadi, J., Razeghi, A., Mansournezhad, A. et al. CuO-CeO2 nanocomposite catalyzed efficient synthesis of aminochromenes. J Nanostruct Chem 3, 85 (2013). https://doi.org/10.1186/2193-8865-3-85
Received:
Accepted:
Published:
DOI: https://doi.org/10.1186/2193-8865-3-85