Abstract
Background
DNA hypermethylation is reported as a frequent event and prognostic marker in head and neck squamous cell carcinomas (HNSCC). Methylation has been commonly assessed with non-quantitative methodologies, such as methylation-specific PCR (MSP). We investigated previously reported hypermethylated genes with quantitative methodology in oral tongue squamous cell carcinomas (OTSCC).
Results
The methylation status of 12 genes in 115 OTSCC samples was assessed by one or more of three quantitative analyses: methylation sensitive high resolution melting (MS-HRM), sensitive-melting analysis after real time-methylation specific PCR (SMART-MSP), and bisulfite pyrosequencing.
In contrast to much of the literature, either no or infrequent locus-specific methylation was identified by MS-HRM for DAPK1, RASSF1A, MGMT, MLH1, APC, CDH1, CDH13, BRCA1, ERCC1, and ATM. The most frequently methylated loci were RUNX3 (18/108 methylated) and ABO (22/107 methylated). Interrogation of the Cancer Genome Atlas (TCGA) HNSCC cohort confirmed the frequency of significant methylation for the loci investigated.
Heterogeneous methylation of RUNX3 (18/108) and ABO (22/107) detected by MS-HRM, conferred significantly worse survival (P = 0.01, and P = 0.03). However, following quantification of methylation levels using pyrosequencing, only four tumors had significant quantities (>15%) of RUNX3 methylation which correlated with a worse patient outcome (P <0.001), while the prognostic significance of ABO hypermethylation was lost. RUNX3 methylation was not prognostic for the TCGA cohort (P = 0.76).
Conclusions
We demonstrated the critical need for quantification of methylation levels and its impact on correlative analyses. In OTSCC, we found little evidence of significant or frequent hypermethylation of many loci reported to be commonly methylated. It is likely that previous reports have overestimated the frequency of significant methylation events as a consequence of the use of non-quantitative methodology.
Similar content being viewed by others
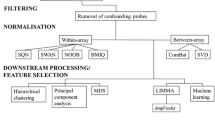
Background
DNA methylation, which is characterized by 5-methylcytosines in CpG dinucleotides, can mediate an epigenetic mechanism of altering gene expression. This epigenetic change provides both an opportunity for therapeutic manipulation, as well as serving as a candidate predictive or prognostic marker [1]. For example, DNA methylation of MGMT is considered as both a prognostic marker for patients with glioblastomas as well as a predictive marker for benefit of the use of the DNA alkylating agent, temozolomide, in the management of this disease [2]. Additionally, the use of demethylating agents has transformed the standard management of high-risk myelodysplastic disorders [3].
As epimutations are more frequent than genetic mutations in the cancer genome [4], DNA methylation forms an ideal putative biomarker. Indeed, DNA hypermethylation is a commonly reported phenomenon for multiple genes in head and neck squamous cell carcinomas (HNSCC). For example, CDKN2A disruption is recognized as an early event in carcinogenesis of HNSCC that can arise due to promoter methylation [5], and additionally may be predictive of disease recurrence [6, 7]. Many other genes have been reported to be frequently methylated across head and neck subsites, including DAPK1, RASSF1A and MGMT[8–10]. However, considerable variation in the frequency of reported methylation events for most loci is described, and correlation with clinicopathological features within heterogeneous cohorts is unclear.
In addition, the common use of non-quantitative methodology hinders the interpretation of the relevance of reported hypermethylated loci. Non-quantitative methodology is prone to the inclusion of false positive results [11–15]. Furthermore, the failure to quantify methylation incorrectly assumes homogeneity of levels and the significance of all detected methylation [14, 15]. One of the most frequently used, non-quantitative techniques is methylation-specific polymerase chain reaction (MSP), which relies only on gel electrophoresis resolution of PCR products to determine the presence of methylation [16].
Thus, in order to identify putative prognostic markers for oral tongue squamous cell carcinomas (OTSCC), we assessed a panel of literature-identified genes reported to be hypermethylated in HNSCC with only quantitative methodology, and we sought to determine any correlation with patient outcome. The loci interrogated included the promoter region of the DNA repair genes: MGMT, MLH1, ATM, BRCA1 and ERCC1[6, 9, 10, 17–20]; genes mediating control of cellular proliferation: RASSF1A, APC and RUNX3[8, 9, 21–23]; the pro-apoptotic tumor suppressor gene: DAPK1[6, 9, 10, 24]; and genes involved in invasion and metastases: CDH1, CDH13 and ABO[9, 24–27]. Table 1 provides a summary of the HNSCC literature in terms of the frequency of methylation events for each gene, the methodology used for the detection of methylation, and the univariate correlative analysis with patient survival.
The variety of loci has been selected for two reasons; firstly, to investigate the large variation in the reported frequency of methylation events, and secondly, to investigate loci relevant to carcinogenesis. Specifically, APC, ATM, CDH1, CDKN2A, DAPK1, MLH1, MGMT, and RASSF1A were chosen due to the high frequency of methylation events reported in the literature and their plausible roles in HNSCC carcinogenesis. In addition, other loci of relevance to cancer were selected. As concurrent cisplatin-based chemoradiotherapy is a standard of care for advanced HNSCC, methylation of ERCC1 was investigated due to reports of the prognostic significance of expression of this nucleotide excision repair protein in other head and neck subsites (laryngeal and nasopharyngeal) [80, 81] and its association with resistance to cisplatin therapy [81–83]. ERCC1 has also been reported by us as being methylated in low frequency in lung cancer [84]. ABO was selected due to reports specifically in oral carcinomas that detected methylation of the gene, loss of expression of these epithelial antigens [28], and frequent alteration of chromosome 9q34 where the ABO gene resides [85, 86]. Methylation of ATM was investigated due to a number of reasons: patients who suffer ataxia telangiectasia defined by ATM gene dysfunction are known to be predisposed to the development of oral carcinomas [87]; there are reports demonstrating high frequencies of ATM methylation in HNSCC [18, 31, 33]; ATM plays a crucial role in double-stranded DNA repair, and thus, gene dysfunction contributes to exquisite sensitivity to radiation treatment, which is a key therapeutic modality for the management of HNSCC [88, 89]; and due to our previous investigation of the impact of other mechanisms of ATM gene loss in HNSCC [90]. BRCA1 was investigated due to its role in homologous DNA repair, and as part of the Fanconi Anemia/BRCA pathway [35, 91, 92], with the known genetic predisposition of patients with Fanconi Anemia to develop malignancies including oral tongue and oral cavity squamous cell carcinomas [93]. CDH13 was of interest as part of the cadherin family, with reports suggesting that methylation of this gene was relevant in HNSCC [24, 30, 32, 94]. Additional reports suggest that methylation of CDH13 has a prognostic role in other smoking-related carcinomas [95].
Therefore, in a uniform head and neck subsite, the oral tongue, this study used quantitative methodologies to investigate a panel of genes previously reported to be hypermethylated in HNSCC.
Results
Clinical details
Details of the full cohort of 131 patients have previously been described [96]. The clinical and tumor characteristics of the 115 patients with bisulfite modified DNA available for methylation analyses are presented in Table 2. Median time of follow-up for the whole cohort was 5.1 (range 0.5 to 9.8) years.
Methylation Sensitive High Resolution Melting (MS-HRM) analyses
A summary of the methylation sensitive high resolution melting (MS-HRM) results for the panel of interrogated genes is presented in Table 3, including results from our previous study on CDKN2A[96].
In comparison to much of the previously published literature, no methylation or infrequent methylation was detected for the majority of loci examined. No significant methylation (0%) was detected in any of the samples for the following genes: DAPK1 (91/91), RASSF1A (49/49), MGMT (50/50), MLH1 (49/49), BRCA1 (45/45), ERCC1 (47/47) and ATM (48/48). For the following loci, the majority of samples had no methylation, but definite methylation was present in a subset of samples: RUNX3 (18/108 methylated), ABO (22/107 methylated), APC (3/105 methylated), CDH1 (13/114 methylated) and CDH13 (4/112 methylated). Both homogeneous and heterogeneous methylation, as ascertained by melting profiles, was observed for these loci.
Heterogeneous melting patterns were detected for ABO (19/107), CDH1 (13/114), CDH13 (3/112) and RUNX3 (18/108). Homogeneous melting patterns were observed for APC in three samples (3/105), all of which had a methylation level of greater than 25%. Homogeneous methylation of ABO was also observed for three samples, which all demonstrated methylation levels of 10 to 25%. Including the samples with heterogeneous methylation, the frequency of methylation events for ABO and RUNX3 were similar to the literature although numerically less [21, 26].
Sensitive-melting analysis after real time-methylation specific PCR (SMART-MSP)
On the basis of reports suggesting frequencies of methylation greater than 20% for both DAPK1 and RASSF1A[8, 9, 68], sensitive-melting analysis after real time-methylation specific PCR (SMART-MSP) analyses were used to confirm a subset of the MS-HRM findings [97]. Utilizing this quantitative modification of MSP permitted the concurrent investigation of possible reasons for discordance between MS-HRM results and the MSP-based literature. SMART-MSP incorporates the use of a DNA intercalating dye, which permits both real-time quantification and melting curve analysis (MCA), which can minimize the number of false positives [97].
The promoter status of DAPK1 for 50 samples was investigated using SMART-MSP. On quantification, the median level of methylation present was 0.0015% (range 0 to 1.3%), with only one sample demonstrating a level of methylation above 1%. However, despite the low levels of methylation quantified, on MCA analyses 41/50 samples demonstrated a pattern consistent with the presence of methylation, emphasizing the sensitivity of MSP. Gel electrophoresis of SMART-MSP products was also performed (Figure 1), and positive bands were detected for samples with calculated quantification of methylation below 0.2%. False positive, similar sized bands were also observed for some samples that failed to amplify during PCR and also for samples with a MCA pattern consistent with unmethylated DNA. SMART-MSP analysis for RASSF1A was performed for ten samples, and no (0%) methylation was detected in these.
Sensitive-melting analysis after real time-methylation specific PCR (SMART-MSP) assessment of DAPK1 . Gel electrophoresis of representative SMART-MSP products in duplicate, assessing methylation of the DAPK1 promoter 1 region. High resolution melting curve analysis (MCA) is represented by + (indicating methylation) and - (indicating no methylation). Quantification of methylation is reported in %. (100% - fully methylated control, 0.1% - 0.1% dilution control, WGA - unmethylated control, NTC – non-template control). The band sizes observed for the 100% methylated control and 0.1% control were appropriately of identical size, due to the inability of this methodology to differentiate quantities of methylation. Sample 6-7 and sample 6-6 demonstrated the presence of methylation on MCA, with gel electrophoresis of PCR products producing band sizes similar to the methylated controls. However, when quantified, the levels of methylation measured below 0.1%. For sample 6-9 and sample 5-5, bands similar to the methylated controls were observed, despite MCA and quantification indicating no detectable levels of methylation. These bands represent false positive results.
Thus, we confirmed with a second quantitative methodology that no significant level of DNA promoter methylation for DAPK1 and RASSF1A was found in the cohort examined. Gel electrophoresis revealed similar sized bands for samples with extremely low levels of methylation or no methylation, illustrating a likely cause in the literature for overestimation of significant methylation events.
Clinical correlation between methylation sensitive high resolution melting (MS-HRM) analyses and patient outcome
Analyses correlating the presence of promoter methylation with patient outcome was possible only for the following genes for which methylation was detected; RUNX3, CDH13, APC, ABO and CDH1. Patients with promoter methylation detected for RUNX3 (18/108, all heterogeneously methylated) had a significantly worse overall survival (OS) compared with patients without methylation, with a five-year OS rate of 32% versus 57% (HR = 2.22, 95% CI: 1.15-4.29, P value = 0.01). This effect was maintained for progression-free survival (PFS) (33% versus 51% five-year PFS; HR = 2.07, 95% CI: 1.03-4.18, P value = 0.04).
While a much smaller number of events was observed for samples demonstrating ABO promoter hypermethylation, for the 3/87 patient samples that demonstrated homogeneous methylation, this significantly correlated with a worse five-year OS rate of 0% versus 57% (HR = 6.51, 95% CI: 1.94-21.8, P value = 0.014). Similar results were found for PFS. The effect was maintained when samples with heterogeneous methylation were included in the analysis (3/107, 10 to 25% homogeneously methylated and 19/107 heterogeneously methylated), with a worse five-year OS rate of 32% versus 57% for methylated samples (HR = 1.98, 95% CI: 1.06-3.70, P value = 0.03). Significant correlation with PFS was not observed.
Bisulfite pyrosequencing analyses and correlation with patient outcome
Given the statistically significant correlation with outcome for patient samples with methylation of RUNX3 and ABO, which included unquantified heterogeneously methylated samples, we sought to investigate this further with bisulfite pyrosequencing.
For the RUNX3 pyrosequencing analysis, 83 samples had sufficient bisulfite modified DNA available for assessment. These samples included the 16/18 samples with heterogeneous methylation identified by MS-HRM. Five samples that failed to produce an adequate signal on pyrosequencing were not included in the analysis. On pyrosequencing, only four samples (4/78) demonstrated mean methylation quantities greater than 15%. The rest of the cohort (74/78), including samples that previously had heterogeneous methylation identified on MS-HRM, had mean quantities of methylation below 12% and thus were scored as negative according to our predefined criteria (Figure 2). A significantly worse outcome was found for the four patients with RUNX3 promoter hypermethylation greater than 15%, with a five-year OS rate of 0% versus 56% (HR = 10.2, 95% CI: 3.23-32.4, P value = 0.001). Given the small number of observed events, firm conclusions could not be made despite the significance of the P value, and further analyses were not pursued.
Pyrosequencing results for all samples demonstrating heterogeneous methylation of RUNX3 when assessed by methylation sensitive high resolution melting (MS-HRM). Each analyzed sample is annotated for the quantity of methylation detected at each CpG site interrogated, and the mean methylation value for all CpG sites analyzed is also provided. This figure demonstrates that the vast majority of samples with heterogeneous methylation for this locus assessed by MS-HRM, had low levels of methylation when quantified by pyrosequencing.
For the ABO pyrosequencing analysis, 85 samples had sufficient bisulfite modified DNA available. Of these, 20/85 samples demonstrated levels of mean methylation greater than 15%. For the statistical analysis, two samples that had demonstrated quantities of methylation between 10 to 25% on MS-HRM but had insufficient DNA for pyrosequencing were also included. For the 22 patients with significant methylation of ABO identified, no statistically significant clinical correlation with survival was found (HR = 1.43, 95% CI: 0.73-2.80, P value = 0.29).
Thus, we demonstrate the impact of quantification of methylation levels. For the investigated loci, though heterogeneous methylation was identified on MS-HRM, the vast majority of these indicated low level methylation events. Of particular note, the initial MS-HRM analysis indicated a frequency of (heterogeneous) RUNX3 methylation of 17% of samples consistent with the literature. However, when quantified by pyrosequencing, the actual frequency of significant methylation events for RUNX3 was considerably less (5%). The presence of RUNX3 promoter methylation was found to correlate with a worse patient outcome, although due to the small numbers robust conclusions cannot be made. In contrast, when ABO promoter methylation was quantified with pyrosequencing, correlation with patient outcome was lost with a minor alteration in the frequency of observed events (22/107, 21% for MS-HRM, and 22/85, 26% for pyrosequencing). These observations highlight the impact of methodology and sample size on correlative analyses.
External validation of results through interrogation of the Cancer Genome Atlas (TCGA) database
To externally validate our findings, which suggested that the frequency of significant methylation events of selected loci was less common than the literature suggested, the Cancer Genome Atlas (TCGA) head and neck cohort were examined. At the time of download (24 June 2013; clinical data download date 22 July 2013), there were 373 TCGA head and neck tumor samples with publically available Infinium HumanMethylation450 beadchip array (HM450K) data available. A total of 26 patients were excluded due to the presence of metastatic disease or an unknown “M-category” (metastasis-category), a history of previous malignancy, or primary site documented as the ‘lip’. Of the remaining 346 patients, 305 patients had both clinical details and methylation data available. Of these 305 patients, 86 patients had OTSCC.
The summary of significant methylation events (β value >0.2) for the examined loci within the TCGA HNSCC cohort is presented in Table 3. For the HM450K probes that overlapped the MS-HRM amplicons, a similar frequency of methylation was observed (see Additional file 1: Figure S1). Methylation levels from probes that flanked the MS-HRM amplicons also indicated a similar frequency of methylation events (see Additional file 1: Figure S2), although comparison across different regions is difficult.
Interestingly, DAPK1 methylation for the probes flanking the MS-HRM amplicon demonstrated a frequency of methylation events of 15% in the heterogeneous HNSCC TCGA cohort and 9% in the TCGA OTSCC subset. Samples with DAPK1 methylation in the whole HNSCC cohort were distributed between multiple head and neck subsites. Methylation of RASSF1A was identified in 4% of samples in the HNSCC TCGA cohort, but none of these were OTSCC samples.
To assess whether RUNX3 promoter methylation had prognostic value in the TCGA cohort, methylation reported from the probes flanking the MS-HRM amplicon was correlated with patient outcome for the whole TCGA HNSCC cohort, and for the subset of patients with OTSCC. There were no statistically significant relationships between methylation and survival identified, when methylation from individual probes or methylation averaged across the two probes were used (HR = 1.08, 95% CI: 0.65-1.82, P = 0.76 for averaged methylation for the whole TCGA cohort, and HR = 1.27, 95% CI: 0.56-2.88, P = 0.57 for averaged methylation for only the OTSCC TCGA subset).
Thus, in an independent, external heterogeneous HNSCC cohort assessed by an orthogonal quantitative methodology, we confirmed the infrequent methylation events for the loci examined. RUNX3 methylation was not a prognostic marker in the TCGA cohort.
Discussion
Due to the known limitations of non-quantitative methodology, we evaluated biomarkers frequently reported to be hypermethylated in HNSCC with three quantitative methodologies, in order to identify meaningful correlations with patient outcome. In contrast to much of the published literature, the majority of loci interrogated in our cohort displayed infrequent or no significant methylation. Independent external validation of these findings was provided by the TCGA cohort of HNSCC samples that were assessed by an orthogonal quantitative methodology. Our findings suggest that previous reports may have overestimated the frequency of significant methylation events with the use of non-quantitative but highly sensitive methodology. Furthermore, this study highlights that the use of quantitative methodology to detect significant methylation events impacts upon the identification of meaningful clinicopathological correlations.
In HNSCC, DNA methylation of a wide number of genes has been reported, with considerable variation in the frequency of detected events. For example, promoter methylation of DAPK1 and RASSF1A have been reported to occur with frequencies between 7 and 81%, and 0 and 44%, respectively, most commonly assessed by non-quantitative MSP (Table 1) [9, 27, 36, 38, 45, 60, 63, 68]. Whether variability has arisen due to methodological or anatomical subsite differences is difficult to assess due to the use of heterogeneous cohorts. For example, in a cohort of 41 laryngeal squamous cell carcinomas, 76% (31/41) of the samples demonstrated methylation of DAPK1 and 32% (13/41) of the samples demonstrated methylation of RASSF1A[68]. In comparison, in 47 oral cavity tumors, a frequency of 30% for DAPK1 and 38% for RASSF1A were reported [29]. Even lower frequencies of methylation have been reported in a heterogeneous HNSCC cohort, where DAPK1 methylation was detected in 12.5% (4/32) samples (excluding two samples with methylation additionally found in matched normal, indicating background methylation or contamination), and 0% (0/32) of samples demonstrated methylation of RASSF1A[36]. In our examination of a single head and neck subsite, using quantitative methodologies, we did not find evidence for frequent methylation of either DAPK1 or RASSF1A. The lower than reported frequency of events detected within our OTSCC cohort was externally validated in the heterogeneous TCGA head and neck cohort (Table 3).
With the use of four orthogonal methodologies, our results demonstrate that one cause for the variability in the frequency of reported methylation events is likely due to the method of assessment of methylation events. Though highly sensitive, techniques such as MSP are well known to be prone to generating false positive results due to the presence of incomplete bisulfite conversion, primer dimer formation, nonspecific primer binding and the subjective nature of determining adequate band intensity representative of a methylated amplicon [11–13]. The limitation of highly sensitive non-quantitative MSP versus quantitative methodology has previously been reported to impact on clinical correlations [98]. Non-quantitative methodology provides no mechanism to account for the detection of low level methylation that can arise from normal infiltrating cells or stromal tissue. In comparison, MS-HRM and SMART-MSP are highly sensitive, semi-quantitative methodologies that provide an advantage for PCR-based assays with the use of melting curve analysis (MCA) [97, 99]. MCA can minimize the inclusion of false positives, allows the quantification of homogeneous methylation, and facilitates the detection of heterogeneous methylation [100].
Gel electrophoresis resolution of generated PCR products from the SMART-MSP analysis permitted the concurrent investigation of whether the use of a non-quantitative methodology may have artificially contributed to the higher frequency of promoter methylation being reported. Using both PCR amplification and MCA to analyze samples for evidence of DAPK1 promoter methylation, we found that 82% (41/50) of samples demonstrated evidence of methylation. Thus, if reported in isolation, these results would be supportive of the literature. However on quantification, samples demonstrated a median quantity of methylation of 0.0015% (range 0 to 1.320%), with only one sample demonstrating methylation above 1%. Furthermore, gel electrophoresis of products demonstrated similar sized bands for insignificant levels of methylation as well as unamplified DNA, emphasizing the limitations of non-quantitative but highly sensitive methodology. Thus, quantification of methylation levels is an important consideration for the identification of significant methylation events and the interpretation of correlative analyses.
Not surprisingly, quantification of methylation influenced the total number of significant events identified defined by the a priori cut-off criteria. However, the use of quantification introduces a number of assumptions. Firstly, the choice of cut-off level is arbitrary and assumes low-level methylation is always irrelevant. The correlation between methylation levels and its impact on gene expression is under investigation, with recent studies demonstrating that the quantity of methylation that alters gene expression differs according to gene and tissue histotype [101]. Our study sought to investigate the significance of methylation as an isolated biomarker, with the use of a cut-off to ensure exclusion of methylation events that arose from normal tissue or were below the sensitivity of the assays used. Secondly, quantitative methodology may not be necessary to identify clinical correlations if the tissue examined demonstrates uniform high levels of methylation that has biological impact.
Our analyses also attempted to account for the potential impact of heterogeneous methylation. Heterogeneous methylation is a complex phenomenon suggestive of epigenetic instability due to incomplete methylation of alleles; however, it is poorly understood and variably detected by existing methodologies [102]. Future research is required to assess methylation levels at the allelic level to enable a comprehensive understanding [103]. Our results demonstrated that if heterogeneous methylation was ignored, then the frequency of reported methylation events for RUNX3 would have been consistent with the literature. However, pyrosequencing results indicated that a large number of these heterogeneous methylation events were not substantially greater than background levels of methylation. Nevertheless, it is important to note that bisulfite pyrosequencing has limitations quantifying methylation, as methylation is averaged at each CpG site and the amount of templates showing methylation cannot be deduced [14].
External validation of our results was provided by interrogation of the methylation status of the TCGA head and neck samples. While comparison with a heterogeneous HNSCC cohort is difficult, as well as comparisons across different loci, our findings were quite similar in regard to the frequency of methylation events identified overall. The absolute differences in the frequency of methylation events between cohorts may have arisen due to anatomical subsite differences in methylation profiles, the limitation of the smaller size of our cohort overall to detect methylation events, the challenges of identifying and quantifying heterogeneous methylation that may be undetected even with MS-HRM and SMART-MSP, and significantly, the different loci examined within similar regions.
Understanding the specific role of promoter DNA methylation in carcinogenesis is complex and many issues require further research. These include the need to identify which genes according to tissue types that have transcription altered by a specific threshold quantity of methylation, to identify if methylation alters gene expression [101]. The identification of appropriate promoter regions or key CpG dinucleotides that influence transcription for individual genes is also important [104, 105]. Though the use of high throughput methylation analyses have rapidly advanced the field, a thorough understanding of the limitations of each platform is critical. For example, the HM450K probes require hybridization over 50 base pairs, which limits the identification of heterogeneous methylation and informative single CpG sites [104, 106]. Validation and translation of putative methylated biomarkers into clinical practice requires the use of reproducible, appropriately sensitive, quantitative methodology.
Overall, we do not suggest that one method of DNA methylation assessment is necessarily superior. However, quantification of methylation is an important consideration to eliminate very low levels of methylation that are unlikely to confer biological impact, and also to minimize the inclusion of false positives. Ultimately, the choice of methodology is likely to impact the interpretation of the results.
Conclusions
In conclusion, in a cohort of OTSCC, we demonstrated that there is limited evidence of DNA hypermethylation at significant quantity or frequency for many genes previously reported to be commonly methylated in HNSCC. Our results presented here and recently presented for other cancers [84], suggest that one reason for the overestimation of the frequency of significant methylation events is from the use of non-quantitative methodology. Non-quantitative methylation analyses in the literature for HNSCC should be cautiously interpreted.
Methods
Patient information
The patient cohort of 131 OTSCC patients has been described previously [96]. Briefly, patients included consisted of those who we could confirm had OTSCC, had comprehensive clinicopathological and outcome data available, and had pre-treatment FFPE blocks containing invasive squamous cell carcinoma. Of these, 115/131 patient samples had sufficient DNA for bisulfite modification and methylation analyses.
DNA extraction, bisulfite modification and preparation of control DNA
A hematoxylin and eosin stained slide representative of each pretreatment tumor specimen was labeled by an expert oral pathologist for areas of invasive tumor. Two millimeter representative cores were punched to extract DNA. After deparaffinization with xylene and daily proteinase K digestion over three days at 56°C, DNA was extracted using the QIAamp DNA Blood Mini Kit (Qiagen, Hilden, Germany). A pre-analytical quality-assurance polymerase chain reaction (QA-PCR) [107] was performed that demonstrated the presence of 300 to 600-bp fragments, confirming the quality of DNA was adequate for methylation assessment. For the generation of a dilution series of control DNA standards, fully methylated human genomic DNA (FMD, Merck Millipore, Billerica, US) was purchased, and secondary whole genome amplification (WGA) product was used as unmethylated DNA. The primary WGA product was generated using the Illustra GenomiPhi V2 DNA Amplification Kit (GE Healthcare, Little Chalfont, UK) and then purified with the QIAquick PCR Purification Kit (Qiagen, Hilden, Germany), according to the manufacturers’ instructions. Tumor DNA and control DNA were quantified using the Qubit 2.0 Fluorometer (Life Technologies, Invitrogen, Oregon, US). Bisulfite modification of one microgram of input DNA was performed according to the manufacturer’s instructions with the Methyl Easy Xceed kit (Human Genetic Signatures, Sydney, Australia) and eluted into two aliquots of 50 μL, to make a theoretical concentration of 10 ng/μL.
Methylation sensitive high resolution melting (MS-HRM)
Methylation was assessed in samples initially with MS-HRM [108]. Amplifiable bisulfite modified FMD was normalized against bisulfite modified unmethylated DNA (WGA) using a real-time quantitative PCR assay that amplified a CpG dinucleotide free region of the COL2A1 gene. Standard dilutions of FMD and WGA were made to 50%, 25%, 10% and 5%. Primers, reaction mixtures and conditions are listed for each promoter region interrogated in Additional file 2: Table S1. MS-HRM analyses were performed on each tumor sample at least in duplicate, with duplicates of the DNA methylation standards, FMD, WGA and non-template control (NTC). Initially, 50 patient samples were assessed for each gene, and then the analysis was extended to the rest of the cohort if any methylation was identified. Heterogeneous methylation was determined as previously described [14, 15, 100]. Briefly, heterogeneous methylation refers to the overall different patterns of methylation that can be observed on any given allele [14], similar to that of genetic mosaicism [109]. When homogeneous methylation is assessed by MS-HRM, melting curves replicate that observed for the FMD or the dilution series of standards. In contrast, when heterogeneous methylation is present, melting curve analysis reveals complex melting patterns as a consequence of heteroduplex formation [14]. If methodology is not specifically chosen to assess the presence of heterogeneous methylation, it will remain undetected. Thus in these instances, the quantity of methylation reported actually represents the mean level of methylation averaged across the entire number of CpG dinucleotides assessed within the amplicon. Importantly, MS-HRM can identify but not quantify heterogeneous methylation.
Sensitive-melting analysis after real time-methylation specific PCR (SMART-MSP)
We sought to confirm a subset of our findings with a second methodology, and concurrently investigate potential reasons for the discordance between our MS-HRM findings and reported literature. SMART-MSP is a quantitative adaption of MSP, which is readily performed with the added benefit of a melting curve analysis step for quality control [97]. The primers for DAPK1 were located within the same region interrogated by the MS-HRM primers and produced a 61-bp amplicon. Due to the requirements of primer design, the SMART-MSP primers were located downstream of the MS-HRM primers. PCR cycling and HRM analysis were performed on a Rotor-Gene Q (Qiagen). Each assay was performed with duplicates of the bisulfite modified standard dilution series of FMD and WGA (50%, 25%, 10%, 5%, 1% and 0.1%), tumor samples, NTC and unmodified genomic DNA. The primer sequences, reaction mixtures and conditions are listed in Additional file 2: Table S2.
Quantification of methylation using Sensitive-melting analysis after real time-methylation specific PCR (SMART-MSP)
The quantity of input DNA of the FMD, WGA and each tumor sample was calibrated against a CpG dinucleotide free region of the COL2A1 gene. The raw run data for COL2A1, DAPK1 and RASSF1A were generated by the Rotor-Gene Q (Qiagen) and exported into LinRegPCR (version 2012.3.0.0) for the estimation of amplification efficiency, after accounting for bias introduced by variable baseline fluorescence [110]. The proportion of methylated templates was then quantified according to methodology previously described [111].
Gel electrophoresis analysis
Four microliters of the SMART-MSP product were resolved on a 3.5% agarose gel stained with SYBR Safe (Invitrogen), at 80 mV over 1.5 hours.
Bisulfite pyrosequencing
The preliminary statistical analysis of the MS-HRM results indicated a significant correlation with worse outcome for samples demonstrating mostly heterogeneous methylation of RUNX3 and ABO. Thus, as MS-HRM cannot quantify heterogeneous methylation, bisulfite pyrosequencing analyses were also performed for these genes. Bisulfite pyrosequencing can quantify the averaged methylation level for each individual CpG dinucleotide within a region of interest [14]. MS-HRM was repeated with the reverse MS-HRM primer containing a 5′-biotin label, and products of duplicates were pooled for bisulfite pyrosequencing [100]. Forward pyrosequencing primers, the interrogated sequence and dispensation order are listed in Additional file 2: Table S3. Analyses were performed on a PyroMark Q96 instrument (Qiagen, Hilden, Germany) with PyroMark Gold Q96 SQA Reagents (Qiagen) according to manufacturer’s instructions, and data was analyzed with the Pyro Q-CpG software (Qiagen, version 1.0.9).
TCGA data and pre-processing
The TCGA database of head and neck cancers was accessed for publically available methylation data assessed by the Infinium HumanMethylation450 beadchip array (HM450K, Illumina inc., San Diego, US).
Methylation data from Level 1 raw IDAT files were obtained from the heterogeneous TCGA HNSCC samples (http://cancergenome.nih.gov/; HM450K download date 24 June 2013; clinical data download date 22 July 2013). Utilizing R software (version 3.0.1; http://cran.r-project.org/), background correction and probe specific correction with SWAN normalization was performed utilizing Methylumi and Minfi packages found in Bioconductor [112–114]. Probes mapping to common variant SNPs or non-unique mappings were removed from the analysis, leaving 330,557 probes for the downstream analysis [115]. Our MS-HRM amplicons interrogating the APC, BRCA1, CDH1, CDH13 and MLH1 genes, overlapped with CpG probes assessed by the HM450K. For the seven genes (ABO, ATM, DAPK1, RASSF1A, MGMT, ERCC1 and RUNX3) that did not have overlapping CpGs on the HM450K, the two probes immediately flanking upstream and downstream of our MS-HRM amplicon were assessed. A β-value greater than 0.2 was used to define the presence of significant methylation [116].
Statistical analysis
In order to account for the sensitivity of assays utilized and background methylation from normal stroma, significant methylation was defined a priori as a quantity 10% and greater for MS-HRM, and 15% and greater for pyrosequencing. A higher cut-off was chosen for pyrosequencing to ensure that only significant quantities of methylation were included on the basis that unmethylated DNA controls (WGA) demonstrated quantities of methylation up to 8% at individual CpG sites (see Additional file 2: Table S4).
As heterogeneous methylation can be identified but not quantified on MS-HRM analysis [100], two analyses were performed: one for samples with an estimated level of methylation 10% and greater while excluding samples with heterogeneous methylation, and the second analysis included all heterogeneously methylated samples in the same category.
Following bisulfite pyrosequencing which can quantify the average methylation at individual CpG dinucleotides for both homogenously and heterogeneously methylated samples, correlation with patient outcome was investigated. The analysis included only samples that had 15% and greater mean methylation on pyrosequencing, or for samples with insufficient DNA for pyrosequencing, those with 10% and greater methylation on MS-HRM were also included.
The Kaplan-Meier product-limit method was used to estimate OS and PFS. OS was defined as the time (in years) from the date of diagnosis to the date of death from any cause. PFS was considered only for patients treated with curative intent and was defined as the time (in years) from the date of diagnosis to the date of progression (loco-regional or distant) or death from any cause. A close-out date of 31 January, 2011, was set for the purpose of analysis. Associations between the presence of methylation and survival outcome were assessed using the exact Log-rank test and Cox proportional hazards regression, to estimate hazard ratios (HR) and associated 95% confidence intervals (95% CIs). P values <0.05 were regarded as statistically significant.
Abbreviations
- CI:
-
confidence intervals
- dHPLC:
-
denaturing high-performance liquid chromatography
- ECOG:
-
Eastern Cooperative Oncology Group
- FMD:
-
fully methylated DNA
- HM450K:
-
Infinium HumanMethylation450 beadchip array
- HNSCC:
-
head and neck squamous cell carcinomas
- HR:
-
hazards ratio
- MCA:
-
melting curve analysis
- MS-HRM:
-
methylation sensitive high resolution melting
- MS-MLPA:
-
methylation specific multiplex ligation-dependent probe amplification
- MSP:
-
methylation-specific PCR
- N-category:
-
Node category
- NTC:
-
non-template control
- OTSCC:
-
oral tongue squamous cell carcinomas
- OS:
-
overall survival
- PCR:
-
polymerase chain reaction
- PFS:
-
progression-free survival
- QA-PCR:
-
quality-assurance PCR
- RE-MSP:
-
restriction enzyme MSP
- SMART-MSP:
-
sensitive-melting analysis after real time-methylation specific PCR
- T-category:
-
Tumor category
- TCGA:
-
the Cancer Genome Atlas
- WGA:
-
whole genome amplified.
References
Sandoval J, Esteller M: Cancer epigenomics: beyond genomics. Curr Opin Genet Dev. 2012, 22: 50-55. 10.1016/j.gde.2012.02.008.
Hegi ME, Diserens AC, Gorlia T, Hamou MF, de Tribolet N, Weller M, Kros JM, Hainfellner JA, Mason W, Mariani L, Bromberg JE, Hau P, Mirimanoff RO, Cairncross JG, Janzer RC, Stupp R: MGMT gene silencing and benefit from temozolomide in glioblastoma. N Engl J Med. 2005, 352: 997-1003. 10.1056/NEJMoa043331.
Fenaux P, Mufti GJ, Hellstrom-Lindberg E, Santini V, Finelli C, Giagounidis A, Schoch R, Gattermann N, Sanz G, List A, Gore SD, Seymour JF, Bennett JM, Byrd J, Backstrom J, Zimmerman L, McKenzie D, Beach CL, Silverman LR: Efficacy of azacitidine compared with that of conventional care regimens in the treatment of higher-risk myelodysplastic syndromes: a randomised, open-label, phase III study. The Lancet Oncology. 2009, 10: 223-232. 10.1016/S1470-2045(09)70003-8.
Baylin SB, Jones PA: A decade of exploring the cancer epigenome - biological and translational implications. Nat Rev Cancer. 2011, 11: 726-734. 10.1038/nrc3130.
Reed AL, Califano J, Cairns P, Westra WH, Jones RM, Koch W, Ahrendt S, Eby Y, Sewell D, Nawroz H, Bartek J, Sidransky D: High frequency of p16 (CDKN2/MTS-1/INK4A) inactivation in head and neck squamous cell carcinoma. Cancer Res. 1996, 56: 3630-3633.
Carvalho AL, Henrique R, Jeronimo C, Nayak CS, Reddy AN, Hoque MO, Chang S, Brait M, Jiang WW, Kim MM, Claybourne Q, Goldenberg D, Khan Z, Khan T, Westra WH, Sidranksy D, Koch W, Califano JA: Detection of promoter hypermethylation in salivary rinses as a biomarker for head and neck squamous cell carcinoma surveillance. Clin Cancer Res. 2011, 17: 4782-4789. 10.1158/1078-0432.CCR-11-0324.
Sinha P, Bahadur S, Thakar A, Matta A, Macha M, Ralhan R, Gupta SD: Significance of promoter hypermethylation of p16 gene for margin assessment in carcinoma tongue. Head Neck. 2009, 31: 1423-1430. 10.1002/hed.21122.
Huang KH, Huang SF, Chen IH, Liao CT, Wang HM, Hsieh LL: Methylation of RASSF1A, RASSF2A, and HIN-1 is associated with poor outcome after radiotherapy, but not surgery, in oral squamous cell carcinoma. Clin Cancer Res. 2009, 15: 4174-4180. 10.1158/1078-0432.CCR-08-2929.
Dikshit RP, Gillio-Tos A, Brennan P, De Marco L, Fiano V, Martinez-Penuela JM, Boffetta P, Merletti F: Hypermethylation, risk factors, clinical characteristics, and survival in 235 patients with laryngeal and hypopharyngeal cancers. Cancer. 2007, 110: 1745-1751. 10.1002/cncr.22975.
Rosas SL, Koch W, da Costa Carvalho MG, Wu L, Califano J, Westra W, Jen J, Sidransky D: Promoter hypermethylation patterns of p16, O6-methylguanine-DNA-methyltransferase, and death-associated protein kinase in tumors and saliva of head and neck cancer patients. Cancer Res. 2001, 61: 939-942.
Kristensen LS, Hansen LL: PCR-based methods for detecting single-locus DNA methylation biomarkers in cancer diagnostics, prognostics, and response to treatment. Clin Chem. 2009, 55: 1471-1483. 10.1373/clinchem.2008.121962.
Brandes JC, Carraway H, Herman JG: Optimal primer design using the novel primer design program: MSPprimer provides accurate methylation analysis of the ATM promoter. Oncogene. 2007, 26: 6229-6237. 10.1038/sj.onc.1210433.
Lee ES, Issa JP, Roberts DB, Williams MD, Weber RS, Kies MS, El-Naggar AK: Quantitative promoter hypermethylation analysis of cancer-related genes in salivary gland carcinomas: comparison with methylation-specific PCR technique and clinical significance. Clin Cancer Res. 2008, 14: 2664-2672. 10.1158/1078-0432.CCR-07-1232.
Mikeska T, Candiloro IL, Dobrovic A: The implications of heterogeneous DNA methylation for the accurate quantification of methylation. Epigenomics. 2010, 2: 561-573. 10.2217/epi.10.32.
Wojdacz TK: The limitations of locus specific methylation qualification and quantification in clinical material. Front Genet. 2012, 3: 21-
Herman JG, Graff JR, Myohanen S, Nelkin BD, Baylin SB: Methylation-specific PCR: a novel PCR assay for methylation status of CpG islands. Proc Natl Acad Sci U S A. 1996, 93: 9821-9826. 10.1073/pnas.93.18.9821.
Tawfik HM, El-Maqsoud NM, Hak BH, El-Sherbiny YM: Head and neck squamous cell carcinoma: mismatch repair immunohistochemistry and promoter hypermethylation of hMLH1 gene. Am J Otolaryngol. 2011, 32: 528-536.
Ai L, Vo QN, Zuo C, Li L, Ling W, Suen JY, Hanna E, Brown KD, Fan CY: Ataxia-telangiectasia-mutated (ATM) gene in head and neck squamous cell carcinoma: promoter hypermethylation with clinical correlation in 100 cases. Cancer Epidemiol Biomarkers Prev. 2004, 13: 150-156. 10.1158/1055-9965.EPI-082-3.
Lima LM, de Souza LR, da Silva TF, Pereira CS, Guimaraes AL, de Paula AM, de Andrade CH: DNA repair gene excision repair cross complementing-group 1 (ERCC1) in head and neck squamous cell carcinoma: analysis of methylation and polymorphism (G19007A), protein expression and association with epidemiological and clinicopathological factors. Histopathology. 2012, 60: 489-496. 10.1111/j.1365-2559.2011.04062.x.
Szaumkessel M, Richter J, Giefing M, Jarmuz M, Kiwerska K, Tonnies H, Grenman R, Heidemann S, Szyfter K, Siebert R: Pyrosequencing-based DNA methylation profiling of Fanconi anemia/BRCA pathway genes in laryngeal squamous cell carcinoma. Int J Oncol. 2011, 39: 505-514.
Supic G, Kozomara R, Jovic N, Zeljic K, Magic Z: Hypermethylation of RUNX3 but not WIF1 gene and its association with stage and nodal status of tongue cancers. Oral Dis. 2011, 17: 794-800. 10.1111/j.1601-0825.2011.01838.x.
Gao S, Eiberg H, Krogdahl A, Liu CJ, Sorensen JA: Cytoplasmic expression of E-cadherin and beta-Catenin correlated with LOH and hypermethylation of the APC gene in oral squamous cell carcinomas. J Oral Pathol Med. 2005, 34: 116-119. 10.1111/j.1600-0714.2004.00275.x.
Tsunematsu T, Kudo Y, Iizuka S, Ogawa I, Fujita T, Kurihara H, Abiko Y, Takata T: RUNX3 has an oncogenic role in head and neck cancer. PLoS One. 2009, 4: e5892-10.1371/journal.pone.0005892.
Worsham MJ, Chen KM, Meduri V, Nygren AO, Errami A, Schouten JP, Benninger MS: Epigenetic events of disease progression in head and neck squamous cell carcinoma. Arch Otolaryngol Head Neck Surg. 2006, 132: 668-677. 10.1001/archotol.132.6.668.
Chang HW, Chow V, Lam KY, Wei WI, Yuen A: Loss of E-cadherin expression resulting from promoter hypermethylation in oral tongue carcinoma and its prognostic significance. Cancer. 2002, 94: 386-392. 10.1002/cncr.10211.
Gao S, Worm J, Guldberg P, Eiberg H, Krogdahl A, Liu CJ, Reibel J, Dabelsteen E: Genetic and epigenetic alterations of the blood group ABO gene in oral squamous cell carcinoma. Int J Cancer. 2004, 109: 230-237. 10.1002/ijc.11592.
Supic G, Kozomara R, Brankovic-Magic M, Jovic N, Magic Z: Gene hypermethylation in tumor tissue of advanced oral squamous cell carcinoma patients. Oral Oncol. 2009, 45: 1051-1057. 10.1016/j.oraloncology.2009.07.007.
Gao S, Bennett EP, Reibel J, Chen X, Christensen ME, Krogdahl A, Dabelsteen E: Histo-blood group ABO antigen in oral potentially malignant lesions and squamous cell carcinoma–genotypic and phenotypic characterization. APMIS. 2004, 112: 11-20. 10.1111/j.1600-0463.2004.apm1120103.x.
Supic G, Kozomara R, Jovic N, Zeljic K, Magic Z: Prognostic significance of tumor-related genes hypermethylation detected in cancer-free surgical margins of oral squamous cell carcinomas. Oral Oncol. 2011, 47: 702-708. 10.1016/j.oraloncology.2011.05.014.
Stephen JK, Chen KM, Shah V, Havard S, Kapke A, Lu M, Benninger MS, Worsham MJ: DNA hypermethylation markers of poor outcome in laryngeal cancer. Clin Epigenetics. 2010, 1: 61-69. 10.1007/s13148-010-0005-3.
Rigi-Ladiz MA, Kordi-Tamandani DM, Torkamanzehi A: Analysis of hypermethylation and expression profiles of APC and ATM genes in patients with oral squamous cell carcinoma. Clin Epigenetics. 2011, 3: 6-10.1186/1868-7083-3-6.
Yalniz Z, Demokan S, Suoglu Y, Ulusan M, Dalay N: Simultaneous methylation profiling of tumor suppressor genes in head and neck cancer. DNA Cell Biol. 2011, 30: 17-24. 10.1089/dna.2010.1090.
Bolt J, Vo QN, Kim WJ, McWhorter AJ, Thomson J, Hagensee ME, Friedlander P, Brown KD, Gilbert J: The ATM/p53 pathway is commonly targeted for inactivation in squamous cell carcinoma of the head and neck (SCCHN) by multiple molecular mechanisms. Oral Oncol. 2005, 41: 1013-1020. 10.1016/j.oraloncology.2005.06.003.
Shaw RJ, Hall GL, Lowe D, Liloglou T, Field JK, Sloan P, Risk JM: The role of pyrosequencing in head and neck cancer epigenetics: correlation of quantitative methylation data with gene expression. Arch Otolaryngol Head Neck Surg. 2008, 134: 251-256. 10.1001/archoto.2007.50.
Marsit CJ, Liu M, Nelson HH, Posner M, Suzuki M, Kelsey KT: Inactivation of the Fanconi anemia/BRCA pathway in lung and oral cancers: implications for treatment and survival. Oncogene. 2004, 23: 1000-1004. 10.1038/sj.onc.1207256.
Maruya S, Issa JP, Weber RS, Rosenthal DI, Haviland JC, Lotan R, El-Naggar AK: Differential methylation status of tumor-associated genes in head and neck squamous carcinoma: incidence and potential implications. Clin Cancer Res. 2004, 10: 3825-3830. 10.1158/1078-0432.CCR-03-0370.
Shaw R, Hobkirk A, Nikolaidis G, Woolgar JA, Triantafyllou A, Brown JS, Liloglou T, Risk JM: Molecular staging of surgical margins in oral squamous cell carcinoma using promoter methylation of p16(INK4A), cytoglobin, E-cadherin, and TMEFF2. Ann Surg Oncol. 2013, 20: 2796-2802. 10.1245/s10434-012-2713-8.
Calmon MF, Colombo J, Carvalho F, Souza FP, Filho JF, Fukuyama EE, Camargo AA, Caballero OL, Tajara EH, Cordeiro JA, Rahal P: Methylation profile of genes CDKN2A (p14 and p16), DAPK1, CDH1, and ADAM23 in head and neck cancer. Cancer Genet Cytogenet. 2007, 173: 31-37. 10.1016/j.cancergencyto.2006.09.008.
Marsit CJ, Posner MR, McClean MD, Kelsey KT: Hypermethylation of E-cadherin is an independent predictor of improved survival in head and neck squamous cell carcinoma. Cancer. 2008, 113: 1566-1571. 10.1002/cncr.23770.
De Schutter H, Geeraerts H, Verbeken E, Nuyts S: Promoter methylation of TIMP3 and CDH1 predicts better outcome in head and neck squamous cell carcinoma treated by radiotherapy only. Oncol Rep. 2009, 21: 507-513.
Steinmann K, Sandner A, Schagdarsurengin U, Dammann RH: Frequent promoter hypermethylation of tumor-related genes in head and neck squamous cell carcinoma. Oncol Rep. 2009, 22: 1519-1526.
Kordi-Tamandani DM, Moazeni-Roodi AK, Rigi-Ladiz MA, Hashemi M, Birjandian E, Torkamanzehi A: Promoter hypermethylation and expression profile of MGMT and CDH1 genes in oral cavity cancer. Arch Oral Biol. 2010, 55: 809-814. 10.1016/j.archoralbio.2010.06.017.
Nakayama S, Sasaki A, Mese H, Alcalde RE, Tsuji T, Matsumura T: The E-cadherin gene is silenced by CpG methylation in human oral squamous cell carcinomas. Int J Cancer. 2001, 93: 667-673. 10.1002/ijc.1386.
Shaw RJ, Liloglou T, Rogers SN, Brown JS, Vaughan ED, Lowe D, Field JK, Risk JM: Promoter methylation of P16, RARbeta, E-cadherin, cyclin A1 and cytoglobin in oral cancer: quantitative evaluation using pyrosequencing. Br J Cancer. 2006, 94: 561-568. 10.1038/sj.bjc.6602972.
Hasegawa M, Nelson HH, Peters E, Ringstrom E, Posner M, Kelsey KT: Patterns of gene promoter methylation in squamous cell cancer of the head and neck. Oncogene. 2002, 21: 4231-4236. 10.1038/sj.onc.1205528.
Su PF, Huang WL, Wu HT, Wu CH, Liu TY, Kao SY: p16(INK4A) promoter hypermethylation is associated with invasiveness and prognosis of oral squamous cell carcinoma in an age-dependent manner. Oral Oncol. 2010, 46: 734-739. 10.1016/j.oraloncology.2010.07.002.
Viswanathan M, Tsuchida N, Shanmugam G: Promoter hypermethylation profile of tumor-associated genes p16, p15, hMLH1, MGMT and E-cadherin in oral squamous cell carcinoma. Int J Cancer. 2003, 105: 41-46. 10.1002/ijc.11028.
Weiss D, Basel T, Sachse F, Braeuninger A, Rudack C: Promoter methylation of cyclin A1 is associated with human papillomavirus 16 induced head and neck squamous cell carcinoma independently of p53 mutation. Mol Carcinog. 2011, 50: 680-688. 10.1002/mc.20798.
Sasiadek MM, Stembalska-Kozlowska A, Smigiel R, Ramsey D, Kayademir T, Blin N: Impairment of MLH1 and CDKN2A in oncogenesis of laryngeal cancer. Br J Cancer. 2004, 90: 1594-1599. 10.1038/sj.bjc.6601679.
Wong TS, Man MWL, Lam AKY, Wei WI, Kwong YL, Yuen APW: The study of p16 and p15 gene methylation in head and neck squamous cell carcinoma and their quantitative evaluation in plasma by real-time PCR. Eur J Cancer. 2003, 39: 1881-1887. 10.1016/S0959-8049(03)00428-3.
Puri SK, Si L, Fan CY, Hanna E: Aberrant promoter hypermethylation of multiple genes in head and neck squamous cell carcinoma. Am J Otolaryngol. 2005, 26: 12-17. 10.1016/j.amjoto.2004.06.007.
Kato K, Hara A, Kuno T, Mori H, Yamashita T, Toida M, Shibata T: Aberrant promoter hypermethylation of p16 and MGMT genes in oral squamous cell carcinomas and the surrounding normal mucosa. J Cancer Res Clin Oncol. 2006, 132: 735-743. 10.1007/s00432-006-0122-8.
Taioli E, Ragin C, Wang XH, Chen J, Langevin SM, Brown AR, Gollin SM, Garte S, Sobol RW: Recurrence in oral and pharyngeal cancer is associated with quantitative MGMT promoter methylation. BMC Cancer. 2009, 9: 354-10.1186/1471-2407-9-354.
Kaur J, Demokan S, Tripathi SC, Macha MA, Begum S, Califano JA, Ralhan R: Promoter hypermethylation in Indian primary oral squamous cell carcinoma. Int J Cancer. 2010, 127: 2367-2373. 10.1002/ijc.25377.
Khor GH, Froemming GR, Zain RB, Abraham MT, Omar E, Tan SK, Tan AC, Vincent-Chong VK, Thong KL: DNA methylation profiling revealed promoter hypermethylation-induced silencing of p16, DDAH2 and DUSP1 in primary oral squamous cell carcinoma. Int J Med Sci. 2013, 10: 1727-1739. 10.7150/ijms.6884.
O’Regan EM, Toner ME, Finn SP, Fan CY, Ring M, Hagmar B, Timon C, Smyth P, Cahill S, Flavin R, Sheils OM, O’Leary JJ: p16(INK4A) genetic and epigenetic profiles differ in relation to age and site in head and neck squamous cell carcinomas. Hum Pathol. 2008, 39: 452-458. 10.1016/j.humpath.2007.08.004.
Lin SC, Chang KW, Chang CS, Liu TY, Tzeng YS, Yang FS, Wong YK: Alterations of p16/MTS1 gene in oral squamous cell carcinomas from Taiwanese. J Oral Pathol Med. 2000, 29: 159-166. 10.1034/j.1600-0714.2000.290403.x.
Farias LC, Fraga CA, De Oliveira MV, Silva TF, Marques-Silva L, Moreira PR, De-Paula AM, Gomez RS, Guimaraes AL: Effect of age on the association between p16CDKN2A methylation and DNMT3B polymorphism in head and neck carcinoma and patient survival. Int J Oncol. 2010, 37: 167-176.
Martone T, Gillio-Tos A, De Marco L, Fiano V, Maule M, Cavalot A, Garzaro M, Merletti F, Cortesina G: Association between hypermethylated tumor and paired surgical margins in head and neck squamous cell carcinomas. Clin Cancer Res. 2007, 13: 5089-5094. 10.1158/1078-0432.CCR-07-0119.
Laytragoon-Lewin N, Chen F, Castro J, Elmberger G, Rutqvist LE, Lewin F, Turesson I, Lundgren J: DNA content and methylation of p16, DAPK and RASSF1A gene in tumour and distant, normal mucosal tissue of head and neck squamous cell carcinoma patients. Anticancer Res. 2010, 30: 4643-4648.
de Freitas C-SM, Stur E, Agostini LP, de Podesta JR, de Oliveira JC, Soares MS, Mendonca EF, Gouvea SA, Von Zeidler SV, Louro ID: Promoter hypermethylation in primary squamous cell carcinoma of the oral cavity and oropharynx: a study of a Brazilian cohort. Mol Biol Rep. 2012, 39: 10111-10119. 10.1007/s11033-012-1885-4.
Gonzalez MV, Pello MF, Lopez-Larrea C, Suarez C, Menendez MJ, Coto E: Deletion and methylation of the tumour suppressor gene p16/CDKN2 in primary head and neck squamous cell carcinoma. J Clin Pathol. 1997, 50: 509-512. 10.1136/jcp.50.6.509.
Ogi K, Toyota M, Ohe-Toyota M, Tanaka N, Noguchi M, Sonoda T, Kohama G, Tokino T: Aberrant methylation of multiple genes and clinicopathological features in oral squamous cell carcinoma. Clin Cancer Res. 2002, 8: 3164-3171.
Sailasree R, Abhilash A, Sathyan KM, Nalinakumari KR, Thomas S, Kannan S: Differential roles of p16INK4A and p14ARF genes in prognosis of oral carcinoma. Cancer Epidemiol Biomarkers Prev. 2008, 17: 414-420. 10.1158/1055-9965.EPI-07-0284.
Sanchez-Cespedes M, Esteller M, Wu L, Nawroz-Danish H, Yoo GH, Koch WM, Jen J, Herman JG, Sidransky D: Gene promoter hypermethylation in tumors and serum of head and neck cancer patients. Cancer Res. 2000, 60: 892-895.
Shiga K, Ogawa T, Katagiri K, Yoshida F, Tateda M, Matsuura K, Kobayashi T: Differences between oral cancer and cancers of the pharynx and larynx on a molecular level. Oncol Lett. 2012, 3: 238-243.
Wu CL, Roz L, McKown S, Sloan P, Read AP, Holland S, Porter S, Scully C, Paterson I, Tavassoli M, Thakker N: DNA studies underestimate the major role of CDKN2A inactivation in oral and oropharyngeal squamous cell carcinomas. Genes Chromosomes Cancer. 1999, 25: 16-25. 10.1002/(SICI)1098-2264(199905)25:1<16::AID-GCC3>3.0.CO;2-2.
Paluszczak J, Misiak P, Wierzbicka M, Wozniak A, Baer-Dubowska W: Frequent hypermethylation of DAPK, RARbeta, MGMT, RASSF1A and FHIT in laryngeal squamous cell carcinomas and adjacent normal mucosa. Oral Oncol. 2011, 47: 104-107. 10.1016/j.oraloncology.2010.11.006.
Huang S, Lee H, Mar K, Ji D, Huang M, Hsia K: Loss expression of O6-methylguanine DNA methyltransferase by promoter hypermethylation and its relationship to betel quid chewing in oral squamous cell carcinoma. Oral Surg Oral Med Oral Pathol Oral Radiol Endod. 2010, 109: 883-889. 10.1016/j.tripleo.2009.12.019.
Esteller M, Hamilton SR, Burger PC, Baylin SB, Herman JG: Inactivation of the DNA Repair Gene O6-Methylguanine-DNA Methyltransferase by Promoter Hypermethylation is a Common Event in Primary Human Neoplasia. Cancer Res. 1999, 59: 793-797.
Zuo C, Ai L, Ratliff P, Suen JY, Hanna E, Brent TP, Fan CY: O6-methylguanine-DNA methyltransferase gene: epigenetic silencing and prognostic value in head and neck squamous cell carcinoma. Cancer Epidemiol Biomarkers Prev. 2004, 13: 967-975.
Czerninski R, Krichevsky S, Ashhab Y, Gazit D, Patel V, Ben-Yehuda D: Promoter hypermethylation of mismatch repair genes, hMLH1 and hMSH2 in oral squamous cell carcinoma. Oral Dis. 2009, 15: 206-213. 10.1111/j.1601-0825.2008.01510.x.
Wang Y, Irish J, MacMillan C, Brown D, Xuan Y, Boyington C, Gullane P, Kamel-Reid S: High frequency of microsatellite instability in young patients with head-and-neck squamous-cell carcinoma: lack of involvement of the mismatch repair genes hMLH1 AND hMSH2. Int J Cancer. 2001, 93: 353-360. 10.1002/ijc.1337.
Demokan S, Suoglu Y, Demir D, Gozeler M, Dalay N: Microsatellite instability and methylation of the DNA mismatch repair genes in head and neck cancer. Ann Oncol. 2006, 17: 995-999. 10.1093/annonc/mdl048.
Sengupta S, Chakrabarti S, Roy A, Panda CK, Roychoudhury S: Inactivation of human mutL homolog 1 and mutS homolog 2 genes in head and neck squamous cell carcinoma tumors and leukoplakia samples by promoter hypermethylation and its relation with microsatellite instability phenotype. Cancer. 2007, 109: 703-712. 10.1002/cncr.22430.
Liu K, Zuo C, Luo QK, Suen JY, Hanna E, Fan CY: Promoter hypermethylation and inactivation of hMLH1, a DNA mismatch repair gene, in head and neck squamous cell carcinoma. Diagn Mol Pathol. 2003, 12: 50-56. 10.1097/00019606-200303000-00007.
Gonzalez-Ramirez I, Ramirez-Amador V, Irigoyen-Camacho ME, Sanchez-Perez Y, Anaya-Saavedra G, Granados-Garcia M, Garcia-Vazquez F, Garcia-Cuellar CM: hMLH1 promoter methylation is an early event in oral cancer. Oral Oncol. 2011, 47: 22-26. 10.1016/j.oraloncology.2010.10.002.
Hogg RP, Honorio S, Martinez A, Agathanggelou A, Dallol A, Fullwood P, Weichselbaum R, Kuo MJ, Maher ER, Latif F: Frequent 3p allele loss and epigenetic inactivation of the RASSF1A tumour suppressor gene from region 3p21.3 in head and neck squamous cell carcinoma. Eur J Cancer. 2002, 38: 1585-1592. 10.1016/S0959-8049(01)00422-1.
Gao F, Huang C, Lin M, Wang Z, Shen J, Zhang H, Jiang L, Chen Q: Frequent inactivation of RUNX3 by promoter hypermethylation and protein mislocalization in oral squamous cell carcinomas. J Cancer Res Clin Oncol. 2009, 135: 739-747. 10.1007/s00432-008-0508-x.
Lee HW, Hwang YH, Han JH, Choi JH, Kang SY, Jeong SH, Ann MS, Oh YT, Kim JH, Kim CH, Sheen SS: High expression of excision repair cross-complementation group 1 protein predicts poor outcome in patients with nasopharyngeal cancer. Oral Oncol. 2010, 46: 209-213. 10.1016/j.oraloncology.2009.12.007.
Handra-Luca A, Hernandez J, Mountzios G, Taranchon E, Lacau-St-Guily J, Soria JC, Fouret P: Excision repair cross complementation group 1 immunohistochemical expression predicts objective response and cancer-specific survival in patients treated by Cisplatin-based induction chemotherapy for locally advanced head and neck squamous cell carcinoma. Clin Cancer Res. 2007, 13: 3855-3859. 10.1158/1078-0432.CCR-07-0252.
Jun HJ, Ahn MJ, Kim HS, Yi SY, Han J, Lee SK, Ahn YC, Jeong HS, Son YI, Baek JH, Park K: ERCC1 expression as a predictive marker of squamous cell carcinoma of the head and neck treated with cisplatin-based concurrent chemoradiation. Br J Cancer. 2008, 99: 167-172. 10.1038/sj.bjc.6604464.
Olaussen KA, Dunant A, Fouret P, Brambilla E, Andre F, Haddad V, Taranchon E, Filipits M, Pirker R, Popper HH, Stahel R, Sabatier L, Pignon JP, Tursz T, Le Chevalier T, Soria JC, Ialt Bio Investigators: DNA repair by ERCC1 in non-small-cell lung cancer and cisplatin-based adjuvant chemotherapy. N Engl J Med. 2006, 355: 983-991. 10.1056/NEJMoa060570.
Do H, Wong NC, Murone C, John T, Solomon B, Mitchell PL, Dobrovic A: A critical re-assessment of DNA repair gene promoter methylation in non-small cell lung carcinoma. Sci Rep. 2014, 4: 4186-
Cervigne NK, Machado J, Goswami RS, Sadikovic B, Bradley G, Perez-Ordonez B, Galloni NN, Gilbert R, Gullane P, Irish JC, Jurisica I, Reis PP, Kamel-Reid S: Recurrent genomic alterations in sequential progressive leukoplakia and oral cancer: drivers of oral tumorigenesis?. Hum Mol Genet. 2014, 23: 2618-2628. 10.1093/hmg/ddt657.
Wolff E, Girod S, Liehr T, Vorderwülbecke U, Ries J, Steininger H, Gebhart E: Oral squamous cell carcinomas are characterized by a rather uniform pattern of genomic imbalances detected by comparative genomic hybridisation. Oral Oncol. 1998, 34: 186-190. 10.1016/S1368-8375(97)00079-1.
Hecht F, Hecht BK: Cancer in ataxia-telangiectasia patients. Cancer Genet Cytogenet. 1990, 46: 9-19. 10.1016/0165-4608(90)90003-S.
Lazar AD, Winter MR, Nogueira CP, Larson PS, Finnemore EM, Dolan RW, Fuleihan N, Chakravarti A, Zietman A, Rosenberg CL: Loss of heterozygosity at 11q23 in squamous cell carcinoma of the head and neck is associated with recurrent disease. Clin Cancer Res. 1998, 4: 2787-2793.
Lavin MF: The Mre11 complex and ATM: a two-way functional interaction in recognising and signaling DNA double strand breaks. DNA Repair (Amst). 2004, 3: 1515-1520. 10.1016/j.dnarep.2004.07.001.
Lim AM, Young RJ, Collins M, Fox SB, McArthur GA, Corry J, Peters L, Rischin D, Solomon B: Correlation of Ataxia-Telangiectasia-Mutated (ATM) gene loss with outcome in head and neck squamous cell carcinoma. Oral Oncol. 2012, 48: 698-702. 10.1016/j.oraloncology.2012.02.014.
Joenje H, Arwert F: Connecting Fanconi anemia to BRCA1. Nat Med. 2001, 7: 406-407. 10.1038/86458.
Timmers C, Taniguchi T, Hejna J, Reifsteck C, Lucas L, Bruun D, Thayer M, Cox B, Olson S, D’Andrea AD, Moses R, Grompe M: Positional cloning of a novel Fanconi anemia gene, FANCD2. Mol Cell. 2001, 7: 241-248. 10.1016/S1097-2765(01)00172-1.
Oksuzoglu B, Yalcin S: Squamous cell carcinoma of the tongue in a patient with Fanconi’s anemia: a case report and review of the literature. Ann Hematol. 2002, 81: 294-298. 10.1007/s00277-002-0455-6.
Sun D, Zhang Z, Van do N, Huang G, Ernberg I, Hu L: Aberrant methylation of CDH13 gene in nasopharyngeal carcinoma could serve as a potential diagnostic biomarker. Oral Oncol. 2007, 43: 82-87. 10.1016/j.oraloncology.2006.01.007.
Brock MV, Hooker CM, Ota-Machida E, Han Y, Guo M, Ames S, Glockner S, Piantadosi S, Gabrielson E, Pridham G, Pelosky K, Belinsky SA, Yang SC, Baylin SB, Herman JG: DNA methylation markers and early recurrence in stage I lung cancer. N Engl J Med. 2008, 358: 1118-1128. 10.1056/NEJMoa0706550.
Lim AM, Do H, Young RJ, Wong SQ, Angel C, Collins M, Takano EA, Corry J, Wiesenfeld D, Kleid S, Sigston E, Lyons B, Fox SB, Rischin D, Dobrovic A, Solomon B: Differential mechanisms of CDKN2A (p16) alteration in oral tongue squamous cell carcinomas and correlation with patient outcome. Int J Cancer. 2014, 135: 887-895. 10.1002/ijc.28727.
Kristensen LS, Mikeska T, Krypuy M, Dobrovic A: Sensitive Melting Analysis after Real Time- Methylation Specific PCR (SMART-MSP): high-throughput and probe-free quantitative DNA methylation detection. Nucleic Acids Res. 2008, 36: e42-10.1093/nar/gkn113.
Claus R, Wilop S, Hielscher T, Sonnet M, Dahl E, Galm O, Jost E, Plass C: A systematic comparison of quantitative high-resolution DNA methylation analysis and methylation-specific PCR. Epigenetics. 2012, 7: 772-780. 10.4161/epi.20299.
Wojdacz TK, Dobrovic A, Hansen LL: Methylation-sensitive high-resolution melting. Nat Protoc. 2008, 3: 1903-1908. 10.1038/nprot.2008.191.
Candiloro IL, Mikeska T, Dobrovic A: Assessing combined methylation-sensitive high resolution melting and pyrosequencing for the analysis of heterogeneous DNA methylation. Epigenetics. 2011, 6: 500-507. 10.4161/epi.6.4.14853.
Liu Y, Ji Y, Qiu P: Identification of thresholds for dichotomizing DNA methylation data. EURASIP J Bioinform Syst Biol. 2013, 2013: 8-10.1186/1687-4153-2013-8.
Cameron EE, Baylin SB, Herman JG: p15INK4B CpG Island Methylation in Primary Acute Leukemia Is Heterogeneous and Suggests Density as a Critical Factor for Transcriptional Silencing. Blood. 1999, 94: 2445-2451.
Wee EJ, Rauf S, Shiddiky MJ, Dobrovic A, Trau M: DNA ligase-based strategy for quantifying heterogeneous DNA methylation without sequencing. Clin Chem. 2014
Claus R, Lucas DM, Stilgenbauer S, Ruppert AS, Yu L, Zucknick M, Mertens D, Buhler A, Oakes CC, Larson RA, Kay NE, Jelinek DF, Kipps TJ, Rassenti LZ, Gribben JG, Dohner H, Heerema NA, Marcucci G, Plass C, Byrd JC: Quantitative DNA methylation analysis identifies a single CpG dinucleotide important for ZAP-70 expression and predictive of prognosis in chronic lymphocytic leukemia. J Clin Oncol. 2012, 30: 2483-2491. 10.1200/JCO.2011.39.3090.
Pulling LC, Grimes MJ, Damiani LA, Juri DE, Do K, Tellez CS, Belinsky SA: Dual promoter regulation of death-associated protein kinase gene leads to differentially silenced transcripts by methylation in cancer. Carcinogenesis. 2009, 30: 2023-2030. 10.1093/carcin/bgp276.
Bibikova M, Barnes B, Tsan C, Ho V, Klotzle B, Le JM, Delano D, Zhang L, Schroth GP, Gunderson KL, Fan JB, Shen R: High density DNA methylation array with single CpG site resolution. Genomics. 2011, 98: 288-295. 10.1016/j.ygeno.2011.07.007.
van Dongen JJ, Langerak AW, Bruggemann M, Evans PA, Hummel M, Lavender FL, Delabesse E, Davi F, Schuuring E, Garcia-Sanz R, van Krieken JH, Droese J, Gonzalez D, Bastard C, White HE, Spaargaren M, Gonzalez M, Parreira A, Smith JL, Morgan GJ, Kneba M, Macintyre EA: Design and standardization of PCR primers and protocols for detection of clonal immunoglobulin and T-cell receptor gene recombinations in suspect lymphoproliferations: report of the BIOMED-2 Concerted Action BMH4-CT98-3936. Leukemia. 2003, 17: 2257-2317. 10.1038/sj.leu.2403202.
Wojdacz TK, Dobrovic A: Methylation-sensitive high resolution melting (MS-HRM): a new approach for sensitive and high-throughput assessment of methylation. Nucleic Acids Res. 2007, 35: e41-10.1093/nar/gkm013.
Worm J, Aggerholm A, Guldberg P: In-tube DNA methylation profiling by fluorescence melting curve analysis. Clin Chem. 2001, 47: 1183-1189.
Ruijter JM, Ramakers C, Hoogaars WM, Karlen Y, Bakker O, van den Hoff MJ, Moorman AF: Amplification efficiency: linking baseline and bias in the analysis of quantitative PCR data. Nucleic Acids Res. 2009, 37: e45-10.1093/nar/gkp045.
Zapparoli GV, Jorissen RN, Hewitt CA, McBean M, Westerman DA, Dobrovic A: Quantitative threefold allele-specific PCR (QuanTAS-PCR) for highly sensitive JAK2 V617F mutant allele detection. BMC Cancer. 2013, 13: 206-10.1186/1471-2407-13-206.
Triche TJ, Weisenberger DJ, Van Den Berg D, Laird PW, Siegmund KD: Low-level processing of Illumina Infinium DNA Methylation BeadArrays. Nucleic Acids Res. 2013, 41: e90-10.1093/nar/gkt090.
Maksimovic J, Gordon L, Oshlack A: SWAN: Subset-quantile within array normalization for illumina infinium HumanMethylation450 BeadChips. Genome Biol. 2012, 13: R44-10.1186/gb-2012-13-6-r44.
Teschendorff AE, Marabita F, Lechner M, Bartlett T, Tegner J, Gomez-Cabrero D, Beck S: A beta-mixture quantile normalization method for correcting probe design bias in Illumina Infinium 450 k DNA methylation data. Bioinformatics. 2013, 29: 189-196. 10.1093/bioinformatics/bts680.
Naeem H, Wong NC, Chatterton Z, Hong MK, Pedersen JS, Corcoran NM, Hovens CM, Macintyre G: Reducing the risk of false discovery enabling identification of biologically significant genome-wide methylation status using the HumanMethylation450 array. BMC Genomics. 2014, 15: 51-10.1186/1471-2164-15-51.
Bibikova M, Le J, Barnes B, Saedinia-Melnyk S, Zhou L, Shen R, Gunderson KL: Genome-wide DNA methylation profiling using Infinium(R) assay. Epigenomics. 2009, 1: 177-200. 10.2217/epi.09.14.
Acknowledgements
A.M. Lim was supported by a Cancer Council of Victoria Postgraduate Cancer Research Scholarship.
A. Dobrovic received funding from the National Breast Cancer Foundation, Cancer Australia and the Cancer Council of Victoria.
Author information
Authors and Affiliations
Corresponding authors
Additional information
Competing interests
The authors declare that they have no competing interests.
Authors’ contributions
AML co-designed and coordinated the project, collected patient samples and clinicopathological data, performed all methylation analyses, assisted with the HM450K analyses, interpreted the data and drafted the manuscript. ILMC co-designed the project, conducted methylation analyses and revised the manuscript. NW performed the bioinformatic analysis of the HM450K data, assisted interpretation of the data and revised the manuscript. MC performed all the statistical analyses and revised the manuscript. HD assisted with the MS-HRM analyses and revised the manuscript. EAT assisted with DNA extraction, MS-HRM analyses and revised the manuscript. CA performed the pathology review of all specimens and revised the manuscript. RJY assisted with sample preparation, sample collection and revised the manuscript. JC provided the patient database, assisted with sample collection, provided clinicopathological data, and revised the manuscript. DW, SK, ES, and BL provided patient clinicopathological data, assisted with sample collection and revised the manuscript. DR and BS co-designed the project, secured funding for the project, provided patient clinicopathological data, assisted with interpretation of the data, and revised the manuscript. AD co-designed the project, assisted with methylation analyses, interpretation of data and revised the manuscript. All authors read and approved the final manuscript.
Electronic supplementary material
13148_2014_93_MOESM1_ESM.docx
Additional file 1: Figure S1: Representative heat maps for the HM450K probes that overlap MS-HRM amplicons for the TCGA HNSCC cohort. These heat maps represent methylation of A) APC and B) CDH1, which both demonstrate low levels or no methylation detected for the examined probes. The key in the top left of the figure indicates the β-value, of which the scale of increasing methylation values is indicated from 0% (0) methylation (red) to 100% (0.1) methylated (bright yellow). A β-value >0.2 is considered a significant quantity of methylation. Figure S2. Representative heat maps for the HM450K probes that flank MS-HRM amplicons for the TCGA HNSCC cohort. These heat maps represent methylation of A) DAPK1 and B) RASSF1A, which demonstrate low levels or no methylation (red) detected for the examined probes. (DOCX 597 KB)
13148_2014_93_MOESM2_ESM.docx
Additional file 2: Table S1: MS-HRM primers, reaction mixtures, and conditions for selected loci. Table S2. SMART-MSP primers, reaction mixtures and conditions for DAPK1 and RASSF1A. Table S3. Pyrosequencing primers, interrogated sequence and dispensation order for ABO and RUNX3. Table S4. Methylation levels detected in the unmethylated control DNA (WGA), assessed by pyrosequencing. (DOCX 20 KB)
Authors’ original submitted files for images
Below are the links to the authors’ original submitted files for images.
Rights and permissions
This article is published under an open access license. Please check the 'Copyright Information' section either on this page or in the PDF for details of this license and what re-use is permitted. If your intended use exceeds what is permitted by the license or if you are unable to locate the licence and re-use information, please contact the Rights and Permissions team.
About this article
Cite this article
Lim, A.M., Candiloro, I.L., Wong, N. et al. Quantitative methodology is critical for assessing DNA methylation and impacts on correlation with patient outcome. Clin Epigenet 6, 22 (2014). https://doi.org/10.1186/1868-7083-6-22
Received:
Accepted:
Published:
DOI: https://doi.org/10.1186/1868-7083-6-22