Abstract
Background
The Anopheles gambiae and Anopheles funestus mosquito species complexes are the primary vectors of Plasmodium falciparum malaria in sub-Saharan Africa. To better understand the environmental factors influencing these species, the abundance, distribution and transmission data from a south-eastern Kenyan study were retrospectively analysed, and the climate, vegetation and elevation data in key locations compared.
Methods
Thirty villages in Malindi, Kilifi and Kwale Districts with data on An. gambiae sensu strict, Anopheles arabiensis and An. funestus entomological inoculation rates (EIRs), were used as focal points for spatial and environmental analyses. Transmission patterns were examined for spatial autocorrelation using the Moran's I statistic, and for the clustering of high or low EIR values using the Getis-Ord Gi* statistic. Environmental data were derived from remote-sensed satellite sources of precipitation, temperature, specific humidity, Normalized Difference Vegetation Index (NDVI), and elevation. The relationship between transmission and environmental measures was examined using bivariate correlations, and by comparing environmental means between locations of high and low clustering using the Mann-Whitney U test.
Results
Spatial analyses indicated positive autocorrelation of An. arabiensis and An. funestus transmission, but not of An. gambiae s.s., which was found to be widespread across the study region. The spatial clustering of high EIR values for An. arabiensis was confined to the lowland areas of Malindi, and for An. funestus to the southern districts of Kilifi and Kwale. Overall, An. gambiae s.s. and An. arabiensis had similar spatial and environmental trends, with higher transmission associated with higher precipitation, but lower temperature, humidity and NDVI measures than those locations with lower transmission by these species and/or in locations where transmission by An. funestus was high. Statistical comparisons indicated that precipitation and temperatures were significantly different between the An. arabiensis and An. funestus high and low transmission locations.
Conclusion
These finding suggest that the abundance, distribution and malaria transmission of different malaria vectors are driven by different environmental factors. A better understanding of the specific ecological parameters of each malaria mosquito species will help define their current distributions, and how they may currently and prospectively be affected by climate change, interventions and other factors.
Similar content being viewed by others
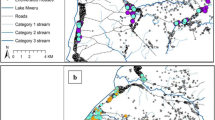
Background
In sub-Saharan Africa, Plasmodium falciparum malaria is primarily transmitted by mosquito species belonging to the Anopheles gambiae and Anopheles funestus complexes [1–4]. The intensity of malaria transmission is heterogeneous across the continent, and influenced by mosquito species' compositions, vector competence, and underlying demographic and environmental factors [5]. High levels of transmission frequently occur where both An. gambiae sensu lato and An. funestus are present, as they tend to exploit different breeding habitats and peak at different times, thereby prolonging the transmission period. Generally, Anopheles gambiae s.l. are most abundant during the rainy season, and An. funestus is predominant at the end of the rains and beginning of the dry season [1–3]. The extent to which these species are influenced by the same environmental factors is largely unknown, as very few studies have examined them simultaneously over a wide geographical range. One of the most comprehensive studies undertaken in Kenya, by Mbogo et al[6], provides an opportunity to retrospectively analyse the spatial abundance, distribution and transmission data on An. gambiae s.l. and An. funestus, and compare climate, vegetation and elevation data derived from remote-sensed satellite sources in key locations.
The study by Mbogo et al[6] provides information on the numbers and transmission intensities, i.e. entomological inoculation rates (EIRs), of An. gambiae s.l. and An. funestus at 30 villages in the Malindi, Kilifi and Kwale Districts along the south-eastern coast of Kenya. Mosquito collections between June 1997 and May 1998 indicated that An. gambiae sensu stricto, Anopheles arabiensis and An. funestus were the main malaria vectors, with differing geographical abundance and transmission patterns over the 200 km study area. Interestingly, An. gambiae s.s. was found to be widespread, whereas An. arabiensis was mostly confined to Malindi in the north and An. funestus to Kwale in the south. Preliminary climate analyses by Mbogo et al[6], found positive correlations between rainfall and the temporal distributions of An. gambiae s.l. and An. funestus, however, these varied by species and between districts, and climate data were limited to one meteorological station in each district.
The recent advances in space technology and increased public access to remote-sensed satellite data provide a cost-effective and efficient alternative to examine relationships between climate, the environment and mosquito vectors of human disease [7, 8]. This is important in poorly resourced regions of the world where the collection of reliable data over large geographical areas is not possible. As a follow-up to the Mbogo et al[6] study, comparisons of satellite-derived precipitation, temperature, humidity, vegetation and elevation measures at each study site in Malindi, Kilifi and Kwale Districts, and in An. gambiae s.s., An. arabiensis and An. funestus clustered locations were carried out.
Methods
First, the average number and daily EIRs of An. gambiae s.l and An. funestus (data from Table 1 in Mbogo et al[6]), and climate, vegetation and elevation data for each district were summarized. Second, the relationship between the relative contribution (%) of the three main species, i.e. An. gambiae s.s, An. arabiensis and An. funestus, to annual EIR (data from Table 2 in Mbogo et al[6]), and each environmental variable was examined using bivariate correlations, and Pearson's correlation coefficient (2-tailed P value ≤ 0.05 significance). Third, the spatial patterns of An. gambiae s.s, An. arabiensis and An. funestus transmission were examined in ArcGIS using Spatial Analyst tools (ESRI, Redland, CA). The Moran's I statistic was used to determine spatial autocorrelation patterns i.e. clustered, dispersed, random, and the Getis-Ord Gi* statistic was to identify the locations with high and low clustering (Z scores, 95% confidence levels (CI) -1.96 and +1.96 standard deviations). The distributions of clustering across the study area were highlighted in relation to elevation, using a 3D wireframe map created in the surface mapping programme Surfer 7.0 (Golden Software Inc., Golden, CO). Mean environmental measures between high and low clustering trends were compared using the Mann-Whitney U test with Bonferroni correction for multiple comparisons. All statistical analyses were performed in Microsoft Excel and SPSS 16.0 (SPSS Inc., Chicago, IL).
Climate and vegetation data corresponding to the 30 mosquito collection sites (i.e. latitude and longitude), and original time period (i.e 1997-1998) were obtained from the best available sources, accessed via the IRI/LDEO Climate Data Library of the International Research Institute for Climate and Society [9]. Average daily precipitation (mm), monthly temperature (C°) and daily specific humidity (qa) measures for each month were extracted from satellite data from the National Oceanic and Atmospheric Administration (NOAA) [10–12]. Vegetation cover was based on Normalized Difference Vegetation Index (NDVI) satellite data extracted from monthly maximum NDVI data available from U.S Geological Survey's (USGS), Africa Data Dissemination Service [13]. Elevation data were derived from the USGS ETOPO2 Digital Elevation Model available from ESRI (Redlands, CA).
Results
District summaries
The findings of these analyses suggest that the different mosquito species compositions found in Malindi, Kilifi and Kwale Districts during 1997 and 1998 may be related to their different climate and topographical profiles. Figure 1 shows that the 10 sites from the Malindi District in the north, comprised predominately of An. gambiae s.l., had significantly (95% CI) higher precipitation, but lower temperature, specific humidity, NDVI and elevation measures than the 10 sites from Kwale District in the south, where An. funestus was most prevalent. Overall, these trends are supported by the correlations between the three main species, and each environmental variable (Table 1). Anopheles gambiae s.s. and An. arabiensis are positively correlated with precipitation, and negatively correlated with temperature and humidity measures. This contrasts to An. funestus, which was significantly negatively correlated with precipitation, but positively with temperature, humidity and NDVI. Interestingly, correlation analysis between each of these three Anopheles species, indicated that An. gambiae s.s (r = -0.454) and An. arabiensis (r = -0.385) were negatively correlated with An. funestus, which is in accordance with observations by Mbogo et al[6].
Spatial analyses
Spatial analyses indicated positive spatial autocorrelation or clustering for An. arabiensis (Moran's I value = 0.18, Z score = 3.8, P ≤ 0.01) and An. funestus (MI = 0.24, Z score = 4.41, P ≤ 0.01), but not for An. gambiae s.s. (MI = 0.03, Z score = 1, P ≥ 0.05). The resultant Z scores of the Getis-Ord Gi* hot spot analyses (using inverse-distance weighting), indicated similar trends with significant spatial clusters of high and low EIR values found for An. arabiensis and An. funestus but not for An. gambiae s.s. The clustering trends are shown in Figure 2, and highlight the distinct patterns of each species across the study region. For An. gambiae s.s, 17 locations had positive Z scores (ranging 0.38 to 1.73) predominantly in the north, while the remaining 13 locations had negative Z scores (ranging -0.16 to -1.70) predominately in the south. For An. arabiensis, six locations with high EIR values were significantly clustered (Z scores ≥ 1.96) in Malindi District, and two with low EIR values (Z score ≤ -1.96) in Kwale District. This contrasts to An. funestus, which had five high EIR values significantly clustered in Kwale District, and five with low EIR values in Malindi District.
Environmental comparisons
For each species, comparisons of environmental measures between locations with high and low transmission trends are shown in Table 2. Due to the small numbers in the study, and few locations with significant spatial clustering, these analyses were limited to mean comparisons between locations with high and low EIR clustering trends defined by positive Z scores (> 0) and negative Z scores (< 0), respectively. Overall, An. gambiae s.s and An. arabiensis showed similar environmental trends, with locations with higher transmission having higher precipitation, but lower temperature, humidity and NDVI measures than those locations with lower transmission by these species and/or where transmission by An. funestus was higher. Notably, locations with higher An. arabiensis transmission trends had markedly low elevations, also illustrated in Figure 2. Statistical comparisons indicated that for An. gambiae s.s there were no significant differences (P value <0.0033 Bonferroni corrected), while for An. arabiensis precipitation and temperatures were found to be significantly different, and for An. funestus precipitation, temperatures and humidity were found to be significantly different between the higher and lower transmission locations.
Conclusion
These simple comparative analyses of 30 sites across three districts in Kenya indicate that An. gambiae s.l and An. funestus can have distinct ecological niches and requirements within a relatively small geographical area. This is supported by other entomological studies carried out in the region, which highlight the heterogeneous nature of these species' seasonality, host feeding preferences [14–16], body size [17] and the distribution and type of breeding sites [18–20]. For example, An. gambiae s.s larvae mostly occur in open shallow sunlit puddles and pools close to homesteads, whereas An. funestus larvae prevail in permanent vegetated aquatic habitats such as stream pools of rivers. In general, malaria transmission by An. funestus predominantly occurs in rural areas of sub-Saharan Africa, and the fact that Kwale District was less urbanized than the other districts [21, 22], may also explain why An. funestus prevailed in this region. Furthermore, the presence of both An. gambiae s.s. and An. funestus, whose ecological requirements may be complementary to each other [23], may also account for the overall higher EIRs found in Kwale District [6].
Changes in the local environment are important to understand because they can create, or reduce the number of, suitable breeding sites for local vectors, thereby affecting their abundance and transmission patterns. In central Kenya, the introduction of irrigated rice cultivation appeared to reduce the risk of malaria transmission by An. funestus but not by An. arabiensis[24], and in Lake Victoria, a recent reduction in water level has created newly emerged land and habitats more suitable for An. funestus than for An. gambiae[25]. Along the Kenyan coast, information on the impact of urbanisation [22], agricultural activities and changes in climate on malaria transmission is limited, but becoming increasingly important. Currently, the prolonged drought affecting Kwale District and other Kenyan communities, has resulted in changes to human food security, population movement, cattle density, grazing and water storage practices [26, 27], which will almost certainly alter vector abundance distributions and the risk of malaria.
Similarly, the impact of interventions such as insecticide-treated bed nets (ITNs), long-lasting insecticide-treated nets (LLINs) and indoor residual spraying (IRS) should be considered as they may affect species differently, especially if distributed widely over large geographical areas. The introduction of ITNs in Kilifi and Kwale District during the 1990s significantly reduced the number of indoor-resting Anopheles species, and a change in mosquito composition and biting times of An. gambiae s.l. [28], and in feeding preference of An. funestus with a shift among the outdoor resting females from endophagy on humans to exophagy on animals [15]. However, these ITNs were restricted to selected areas and are unlikely to have affected the overall relative abundance of the difference species in the study region. Other studies in East Africa [29, 30] and elsewhere [31–33] have shown that An. funestus can be readily eliminated from an entire area by IRS programmes. However, this vector can reappear and become widespread again, sometimes with resistance to the insecticides used in the spray campaign [34–36]. This poses a further complication for vector control programmes. It also emphasizes the need for on-going mosquito and insecticide resistance surveillance [37], especially given the mass distribution of LLINs and IRS programmes currently taking place across sub-Saharan Africa, which could alter mosquito compositions and transmission dynamics over time [38].
Although there was considerable overlap between An. gambiae s.s. and An. arabiensis, An. gambiae s.s had no significant clustering or environmental differences between high and low transmission locations. The reasons for this may be related to its wide distribution and ability to exploit a range of habitats [1–3, 18], but may also be because this species may comprise different molecular or chromosomal forms which are not well defined in this region compared with other regions of sub-Saharan Africa [1]. In West Africa, the chromosomal forms of An. gambiae s.s have shown to have differing spatial distributions and environmental parameters [39–41], and distinct differences between the M and S molecular forms have been described in Mali [42]. In this coastal region of Kenya, only the An. gambiae S form has been detected in two locations [43], therefore, a better understanding of the speciation and transmission patterns of the An. gambiae s.s forms is crucial, especially as An. gambiae s.l appears to be the main vector of both malaria and lymphatic filariasis in Kilifi and Kwale Districts [15, 44, 45].
The study by Mbogo et al[6] collected mosquitoes using pyrethroid spray catches (PSC) inside houses, which could potentially underestimate the abundance of exophilic mosquito species such as An. arabiensis, as shown in other East African countries [46]. In general, measuring the population dynamics of An. gambiae s.l and An. funestus is difficult, and studies have shown great variability depending on the sampling technique used, and whether interventions such as ITNs are present and acting as a deterrent [16, 28, 47–49]. The presence of cattle for An. arabiensis is also an important consideration as they prefer to feed on these animals over humans and other livestock [46, 50, 51]. Although there are limitations to using the PSC method to estimate abundance and transmission patterns, the study by Mbogo et al[6] is one of largest datasets available for East Africa, which compares the abundance and transmission potential of An. gambiae s.l and An. funestus across a diverse ecological range using a standard sampling technique.
Malaria transmission is complex, and more knowledge on the relationship between the environment, mosquito vectors, human disease and demography in sub-Saharan Africa will help implement appropriate control measures in a rapidly changing landscape. This is particularly important in areas already reporting changes in transmission intensity [52, 53], and may be additional factors to include in future malaria models. This small follow-up study to Mbogo et al[6] aimed to elucidate environmental factors associated with the An. gambiae and An. funestus complexes in one region of Kenya. It exemplifies what can be done with existing entomological data contained in the literature and elsewhere, and how modern satellite and GIS technologies in public health research may be exploited, especially for climate sensitive diseases in developing countries, such as malaria [54, 55].
References
Coetzee M, Craig M, le Sueur D: Distribution of African malaria mosquitoes belonging to the Anopheles gambiae complex. Parasitol Today. 2000, 16: 74-77. 10.1016/S0169-4758(99)01563-X.
Coetzee M, Fontenille D: Advances in the study of Anopheles funestus, a major vector of malaria in Africa. Insect Biochem Mol Biol. 2004, 34: 599-605. 10.1016/j.ibmb.2004.03.012.
Gillies MT, De Meillon B: The Anophelinae of Africa South of the Sahara. South African Institute for Medical Research. 1968, 54: 127-150.
Hay SI, Guerra CA, Tatem AJ, Atkinson PM, Snow RW: Urbanization, malaria transmission and disease burden in Africa. Nat Rev Microbiol. 2005, 3: 81-90. 10.1038/nrmicro1069.
Kelly-Hope LA, McKenzie FE: The multiplicity of malaria transmission: a review of entomological inoculation rate measurements and methods across sub-Saharan Africa. Malar J. 2009, 8: 19-10.1186/1475-2875-8-19.
Mbogo CM, Mwangangi JM, Nzovu J, Gu W, Yan G, Gunter JT, Swalm C, Keating J, Regens JL, Shililu JI, Githure JI, Beier JC: Spatial and temporal heterogeneity of Anopheles mosquitoes and Plasmodium falciparum transmission along the Kenyan coast. Am J Trop Med Hyg. 2003, 68: 734-742.
Hay SI, Omumbo JA, Craig MH, Snow RW: Earth observation, geographic information systems and Plasmodium falciparum malaria in sub-Saharan Africa. Adv Parasitol. 2000, 47: 173-215. full_text.
Hay SI, Tatem AJ, Graham AJ, Goetz SJ, Rogers DJ: Global environmental data for mapping infectious disease distribution. Adv Parasitol. 2006, 62: 37-77. 10.1016/S0065-308X(05)62002-7.
International Research Institute for Climate and Society (IRI): IRI/LDEO Climate Data Library. Columbia University, New York, [http://iridl.ldeo.columbia.edu/index.html]
National Oceanic and Atmospheric Administration (NOAA): Precipitation data: NOAA NCEP CPC FEWS Africa DAILY ARC daily est_prcp. Washington, DC, USA. [http://iridl.ldeo.columbia.edu/SOURCES/.NOAA/.NCEP/.CPC/.FEWS/.Africa/.DAILY/.ARC/.daily/.est_prcp/]
National Oceanic and Atmospheric Administration (NOAA): Temperature data: NOAA NCEP-NCAR CDAS-1 MONTHLY Diagnostic above ground temp. Washington, DC, USA. [http://iridl.ldeo.columbia.edu/SOURCES/.NOAA/.NCEP-NCAR/.CDAS-1/.MONTHLY/.Diagnostic/.above_ground/.temp/]
National Oceanic and Atmospheric Administration (NOAA): Specific humidity data: NOAA NCEP-NCAR CDAS-1 DAILY Diagnostic above_ground qa. Washington, DC, USA. [http://iridl.ldeo.columbia.edu/SOURCES/.NOAA/.NCEP-NCAR/.CDAS-1/.DAILY/.Diagnostic/.above_ground/.qa/]
U.S Geological Survey: Normalized Difference Vegetation Index: USGS ADDS NDVI NDVIg monthly c8204 avgNDVImonmax: 1982-2004 Avg. of Monthly Maximum NDVI data. [http://iridl.ldeo.columbia.edu/SOURCES/.USGS/.ADDS/.NDVI/.NDVIg/.monthly/.c8204/.avgNDVImonmax/]
Mwangangi JM, Mbogo CM, Nzovu JG, Githure JI, Yan G, Beier JC: Blood meal analysis for anopheline mosquitoes sampled along the Kenyan coast. J Am Mosq Contr Assoc. 2003, 19: 371-375.
Bøgh C, Pedersen EM, Mukoko DA, Ouma JH: Permethrin-impregnated bednet effects on resting and feeding behaviour of lymphatic filariasis vector mosquitoes in Kenya. Med Vet Entomol. 1998, 12: 52-59. 10.1046/j.1365-2915.1998.00091.x.
Mbogo CNM, Kabiru EW, Muiruri SK, Nzovu JM, Ouma JH, Githure I, Beier JC: Blood feeding behavior of Anopheles gambiae s.l. and Anopheles funestus in Kilifi district. Kenya. J Am Mosq Contr Assoc. 1993, 9: 225-227.
Mwangangi JM, Mbogo CM, Nzovu JG, Kabiru EW, Mwambi H, Githure JI, Beier JC: Relationships between body size of Anopheles mosquitoes and Plasmodium falciparum sporozoite rates along the Kenya Coast. J Am Mosq Contr Assoc. 2004, 20: 390-394.
Mwangangi JM, Mbogo CM, Muturi EJ, Nzovu JG, Githure JI, Yan G, Minakawa N, Novak R, Beier JC: Spatial distribution and habitat characterisation of Anopheles larvae along the Kenyan coast. J Vect Borne Dis. 2007, 44: 44-51.
Mbogo CNM, Snow RW, Khamala CPM, Kabiru EW, Ouma JH, Githure JI, Marsh K, Beier JC: Relationships between Plasmodium falciparum transmission by vector populations and the incidence of severe disease at nine sites on the Kenyan coast. Am J Trop Med Hyg. 1995, 52: 201-206.
Mosha FW, Petrarca V: Ecological studies on Anopheles gambiae complex sibling species on the Kenyan coast. Trans R Soc Trop Med Hyg. 1983, 77: 344-345. 10.1016/0035-9203(83)90161-X.
CIESIN/CIAT: Gridded Population of the World (GPW), version 3. Palisades, New York CIESIN, Columbia University, Centre for International Earth Science Information Network (CIESIN), Colombia University; Centro Internacional de Agricultura Tropical (CIAT). 2004, [http://sedac.ciesin.columbia.edu/gpw/]
Macintyre K, Keating J, Sosler S, Kibe L, Mbogo CM, Githeko AK, Beier JC: Examining the determinants of mosquito-avoidance practices in two Kenyan cities. Malar J. 2002, 15 (1): 14-10.1186/1475-2875-1-14.
Gillies MT: The density of adult Anopheles in the neighbourhood of an East African village. Am J Trop Med Hyg. 1955, 4: 1103-1113.
Muturi EJ, Muriu S, Shililu J, Mwangangi J, Jacob BG, Mbogo C, Githure J, Novak RJ: Effect of rice cultivation on malaria transmission in central Kenya. Am J Trop Med Hyg. 2008, 78: 270-5.
Minakawa N, Sonye G, Dida GO, Futami K, Kaneko S: Recent reduction in the water level of Lake Victoria has created more habitats for Anopheles funestus. Malar J. 2008, 7: 119-10.1186/1475-2875-7-119.
Kenya Red Cross Society: Kenya Drought Appeal 2009. Nairobi, Kenya. [http://www.kenyaredcross.org/index.php?option=com_content&task=view&id=167&Itemid=117]
ReliefWeb: FEWS Kenya Food Security Update Jun 2009 - Food security continues to deteriorate. Geneva, Switzerland. [http://www.reliefweb.int/rw/rwb.nsf/db900sid/MYAI-7TJ3NL?OpenDocument]
Mbogo CNM, Baya NM, Ofulla AVO, Githure JI, Snow RW: The impact of permethrin-impregnated bednets on malaria vectors on the Kenyan coast. Med Vet Entomol. 1996, 10: 251-259. 10.1111/j.1365-2915.1996.tb00739.x.
Pringle G: Malaria in the Pare area of Tanzania. 3. The course of malaria transmission since the suspension of an experimental programme of residual insecticide spraying. Trans R Soc Trop Med Hyg. 1967, 61: 69-79. 10.1016/0035-9203(67)90055-7.
Pringle G: Experimental malaria control and demography in a rural East African community: a retrospect. Trans R Soc Trop Med Hyg. 1969, 63: s2-s18. 10.1016/0035-9203(69)90201-6.
Molineaux L, Gramiccia G: The Garki project: research on the epidemiology and control of malaria in the Sudan savanna of West Africa. 1980, World Health Organization (WHO), Geneva
Sharp BL, Ridl FC, Govender D, Kuklinski J, Kleinschmidt I: Malaria vector control by indoor residual insecticide spraying on the tropical island of Bioko, Equatorial Guinea. Malar J. 2007, 6: 52-10.1186/1475-2875-6-52.
Sharp BL, Kleinschmidt I, Streat E, Maharaj R, Barnes KI, Durrheim DN, Ridl FC, Morris N, Seocharan I, Kunene S, LA Grange JJ, Mthembu JD, Maartens F, Martin CL, Barreto A: Seven years of regional malaria control collaboration--Mozambique, South Africa, and Swaziland. Am J Trop Med Hyg. 2007, 76: 42-47.
Hargreaves K, Koekemoer LL, Brooke BD, Hunt RH, Mthembu J, Coetzee M: Anopheles funestus is resistant to pyrethroid insecticides in South Africa. Med Vet Entomol. 2000, 14: 181-189. 10.1046/j.1365-2915.2000.00234.x.
Brooke BD, Kloke G, Hunt RH, Koekemoer LL, Temu EA, Taylor ME, Small G, Hemingway J, Coetzee M: Bioassay and biochemical analyses of insecticide resistance in southern African Anopheles funestus (Diptera: Culicidae). Bull Entomol Res. 2001, 91: 265-272.
Casimiro S, Coleman M, Mohloai P, Hemingway J, Sharp B: Insecticide resistance in Anopheles funestus (Diptera: Culicidae) from Mozambique. J Med Entomol. 2006, 43: 267-75. 10.1603/0022-2585(2006)043[0267:IRIAFD]2.0.CO;2.
Kelly-Hope L, Ranson H, Hemingway J: Lessons from the past: managing insecticide resistance in malaria control and eradication programmes. Lancet Infect Dis. 2008, 8: 387-9. 10.1016/S1473-3099(08)70045-8.
Enayati A, Hemingway J: Malaria Management: Past, Present, and Future. Annu Rev Entomol. Epub 2009 Sep 15
Coluzzi M, Sabatini A, Petrarca V, Di Deco MA: Chromosomal differentiation and adaptation to human environments in the Anopheles gambiae complex. Trans R Soc Trop Med Hyg. 1979, 73: 483-497. 10.1016/0035-9203(79)90036-1.
della Torre A, Fanello C, Akogbeto M, Dossou-yovo J, Favia G, Petrarca V, Coluzzi M: Molecular evidence of incipient speciation within Anopheles gambiae s.s. in West Africa. Insect Mol Biol. 2001, 10: 9-18. 10.1046/j.1365-2583.2001.00235.x.
Bayoh MN, Thomas CJ, Lindsay SW: Mapping distributions of chromosomal forms of Anopheles gambiae in West Africa using climate data. Med Vet Entomol. 2001, 15: 267-274. 10.1046/j.0269-283x.2001.00298.x.
Sogoba N, Vounatsou P, Bagayoko MM, Doumbia S, Dolo G, Gosoniu L, Traoré SF, Smith TA, Touré YT: Spatial distribution of the chromosomal forms of anopheles gambiae in Mali. Malar J. 2008, 7: 205-10.1186/1475-2875-7-205.
Lehmann T, Licht M, Elissa N, Maega BT, Chimumbwa JM, Watsenga FT, Wondji CS, Simard F, Hawley WA: Population Structure of Anopheles gambiae in Africa. J Hered. 2003, 94: 133-47. 10.1093/jhered/esg024.
Muturi EJ, Mbogo CM, Mwangangi JM, Ng'ang'a ZW, Kabiru EW, Mwandawiro C, Beier JC: Concomitant infections of Plasmodium falciparum and Wuchereria bancrofti on the Kenyan coast. Filaria J. 2006, 5: 8-10.1186/1475-2883-5-8.
Muturi EJ, Mbogo CM, Ng'ang'a ZW, Kabiru EW, Mwandawiro C, Novak RJ, Beier JC: Relationship between malaria and filariasis transmission indices in an endemic area along the Kenyan Coast. J Vector Borne Dis. 2006, 43: 77-83.
Tirados I, Costantini C, Gibson G, Torr SJ: Blood-feeding behaviour of the malarial mosquito Anopheles arabiensis : implications for vector control. Med Vet Entomol. 2006, 20: 425-37. 10.1111/j.1365-2915.2006.652.x.
Gimnig JE, Kolczak MS, Hightower AW, Vulule JM, Schoute E, Kamau L, Phillips-Howard PA, ter Kuile FO, Nahlen BL, Hawley WA: Effect of permethrin-treated bed nets on the spatial distribution of malaria vectors in western Kenya. Am J Trop Med Hyg. 2003, 68 (4 Suppl): 115-20.
Mathenge EM, Gimnig JE, Kolczak M, Ombok M, Irungu LW, Hawley WA: Effect of permethrin-impregnated nets on exiting behavior, blood feeding success, and time of feeding of malaria mosquitoes (Diptera: Culicidae) in western Kenya. J Med Entomol. 2001, 38: 531-6. 10.1603/0022-2585-38.4.531.
Mathenge EM, Misiani GO, Oulo DO, Irungu LW, Ndegwa PN, Smith TA, Killeen GF, Knols BG: Comparative performance of the Mbita trap, CDC light trap and the human landing catch in the sampling of Anopheles arabiensis, An. funestus and culicine species in a rice irrigation in western Kenya. Malar J. 2005, 4: 7-10.1186/1475-2875-4-7.
Kweka EJ, Mwang'onde BJ, Kimaro E, Msangi S, Massenga CP, Mahande AM: A resting box for outdoor sampling of adult Anopheles arabiensis in rice irrigation schemes of lower Moshi, northern Tanzania. Malar J. 2009, 8: 82-10.1186/1475-2875-8-82.
Mahande AM, Mosha FW, Mahande JM, Kweka EJ: Feeding and resting behaviour of malaria vector, Anopheles arabiensis with reference to zooprophylaxis. Malar J. 2007, 6: 100-10.1186/1475-2875-6-100.
Okiro EA, Hay SI, Gikandi PW, Sharif SK, Noor AM, Peshu N, Marsh K, Snow RW: The decline in paediatric malaria admissions on the coast of Kenya. Malar J. 2007, 6: 151-10.1186/1475-2875-6-151.
O'Meara WP, Bejon P, Mwangi TW, Okiro EA, Peshu N, Snow RW, Newton CR, Marsh K: Effect of a fall in malaria transmission on morbidity and mortality in Kilifi, Kenya. Lancet. 2008, 372: 1555-1562. 10.1016/S0140-6736(08)61655-4.
Cano J, Descalzo MA, Moreno M, Chen Z, Nzambo S, Bobuakasi L, Buatiche JN, Ondo M, Micha F, Benito A: Spatial variability in the density, distribution and vectorial capacity of anopheline species in a high transmission village (Equatorial Guinea). Malar J. 2006, 5: 21-10.1186/1475-2875-5-21.
Bøgh C, Lindsay SW, Clarke SE, Dean A, Jawara M, Pinder M, Thomas CJ: High spatial resolution mapping of malaria transmission risk in the Gambia, west Africa, using LANDSAT TM satellite imagery. Am J Trop Med Hyg. 2007, 76: 875-881.
Acknowledgements
We are grateful to Dr Michael Bell, from The International Research Institute (IRI) for Climate and Society, The Earth Institute, Columbia University, USA for his advice on the best available environmental satellite data sources for this study.
Author information
Authors and Affiliations
Corresponding author
Additional information
Competing interests
The authors declare that they have no competing interests.
Authors' contributions
LKH identified data sources, designed the study, carried out the data analysis and wrote the first draft of the manuscript. JH participated in the interpretation of the results and editing of the manuscript. EM conceived the idea for the study, and contributed to the writing and editing of the manuscript. All authors have read and approved the final manuscript.
Authors’ original submitted files for images
Below are the links to the authors’ original submitted files for images.
Rights and permissions
This article is published under license to BioMed Central Ltd. This is an Open Access article distributed under the terms of the Creative Commons Attribution License (http://creativecommons.org/licenses/by/2.0), which permits unrestricted use, distribution, and reproduction in any medium, provided the original work is properly cited.
About this article
Cite this article
Kelly-Hope, L.A., Hemingway, J. & McKenzie, F.E. Environmental factors associated with the malaria vectors Anopheles gambiae and Anopheles funestus in Kenya. Malar J 8, 268 (2009). https://doi.org/10.1186/1475-2875-8-268
Received:
Accepted:
Published:
DOI: https://doi.org/10.1186/1475-2875-8-268