Abstract
Background
The majority of the mosquito and parasite life-history traits that combine to determine malaria transmission intensity are temperature sensitive. In most cases, the process-based models used to estimate malaria risk and inform control and prevention strategies utilize measures of mean outdoor temperature. Evidence suggests, however, that certain malaria vectors can spend large parts of their adult life resting indoors.
Presentation of hypothesis
If significant proportions of mosquitoes are resting indoors and indoor conditions differ markedly from ambient conditions, simple use of outdoor temperatures will not provide reliable estimates of malaria transmission intensity. To date, few studies have quantified the differential effects of indoor vs outdoor temperatures explicitly, reflecting a lack of proper understanding of mosquito resting behaviour and associated microclimate.
Testing the hypothesis
Published records from 8 village sites in East Africa revealed temperatures to be warmer indoors than outdoors and to generally show less daily variation. Exploring the effects of these temperatures on malaria parasite development rate suggested indoor-resting mosquitoes could transmit malaria between 0.3 and 22.5 days earlier than outdoor-resting mosquitoes. These differences translate to increases in transmission risk ranging from 5 to approaching 3,000%, relative to predictions based on outdoor temperatures. The pattern appears robust for low- and highland areas, with differences increasing with altitude.
Implications of the hypothesis
Differences in indoor vs outdoor environments lead to large differences in the limits and the intensity of malaria transmission. This finding highlights a need to better understand mosquito resting behaviour and the associated microclimate, and to broaden assessments of transmission ecology and risk to consider the potentially important role of endophily.
Similar content being viewed by others
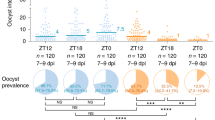
Background
The transmission intensity of malaria is strongly influenced by environmental temperature [1–5]. This effect derives from the fact that malaria mosquitoes are small, coldblooded insects, with body temperatures that will tend to closely track that of the direct surrounding environment. As temperature changes, so will mosquito (and parasite) physiology and associated ecology.
Most biological process-based models that approximate malaria risk use mean (often monthly) outdoor air temperature to estimate the various mosquito and parasite life history parameters that combine to determine transmission intensity [1, 6–11]. This approach has been challenged recently with studies demonstrating, for example, the additional influence of daily temperature variation [5, 12] and the importance of microclimatic differences between adult and larval mosquito habitats [13]. The aim of the current study is to highlight a further factor shaping the effects of temperature on malaria risk, namely the influence of indoor vs outdoor temperatures.
The mosquito gonotrophic cycle (blood-feeding, egg maturation and oviposition, which are repeated several times throughout adult life) can be as short as two days, but could take over a week, depending on temperature [14–16]. If a mosquito lays eggs and searches for a new blood meal during a single night (as appears appropriate for Anopheles gambiae, which has been observed to deposit the majority of eggs in the first hours after sunset [17, 18] and to bite throughout the night [19–21]), then a large portion of the gonotrophic cycle is spent resting. But where do mosquitoes rest?
Mosquito resting behaviour can be divided into two categories. An endophilic mosquito is defined as a mosquito that rests indoors, inside a human dwelling, during the period between the end of blood-feeding and the onset of searching for an oviposition site [22]. An exophilic mosquito spends this period somewhere outside the human dwelling (Figure 1). Of the key malaria vectors in sub-Saharan Africa, Anopheles gambiae s.s. is thought to be largely endophilic and spend considerable time indoors [23–27], although exophily has been reported [28, 29]. Anopheles funestus is also usually classified as a more endophilic species [25, 26, 30–33] but again, exophilic behaviour is observed [34]. Anopheles arabiensis, in contrast, is classified as a more exophilic species [23, 25, 29, 33–36], although the reverse behaviour (endophily) is also reported [24, 27, 37, 38].
Schematic overview of the gonotrophic cycle of mosquitoes that both feed (exophagic) and rest (exophilic) outdoors (left hand side of figure), or both feed (endophagic) and rest (endophilic) indoors (right hand side of figure). At these extremes, mosquitoes will spend the majority of their adult lives under dramatically different environmental conditions.
Overall, while there appear some generalizations, resting behaviour appears to be relatively plastic with considerable potential for variation between and within species. Indeed, a couple of studies have reported no significant tendency to repeated endophily or exophily for even the same individual mosquito [26, 39].
Presentation of the hypothesis
If mosquitoes are at least partially endophilic, then estimating transmission intensity requires measures of indoor as well as outdoor temperatures. Unfortunately, there are only a few studies that actually measure the mean indoor and outdoor temperature simultaneously, and even fewer studies that keep track of the daily temperature variability. From the limited studies that exist, it appears that indoor temperatures in traditional houses are a few degrees Celsius higher than the outdoor temperature (Table 1, [14, 40–44]). Additionally, the indoor daily temperature range (or DTR, the difference between daily minimum and maximum temperature) in traditional houses tends to be smaller than the outdoor DTR (Table 1, [45, 46]). That said, and the relatively modest DTRs shown in Table 1 notwithstanding, large DTRs of 10-15°C are commonly observed indoors [40, 41, 47, 48].
The difference between indoor and outdoor temperatures is expected to alter temperature-related estimates of transmission intensity. Some studies have attempted to account for this effect by applying a simple temperature correction [49–51], and one study included actual indoor temperature [41]. However, few (if any) studies have quantified the differential effects of indoor vs outdoor temperatures explicitly.
Testing of the hypothesis
The parasite development time, or extrinsic incubation period (EIP) is one of the most influential parameters determining transmission intensity, as defined by the basic reproductive number, R 0 [52]. EIP is known to be highly temperature sensitive [5, 52, 53]. Based on the data presented in Table 1 the effects of mean temperature and DTR inside and outside human dwellings on EIP was assessed using two different malaria development models: the widely used day-degree Detinova model [53], and the non-linear thermodynamic model proposed by Paaijmans and colleagues [12]. Using the reported minimum and maximum temperatures (Table 1, [50, 54]), air temperature [55] and EIP [for methods see 12] were modelled at 30min intervals.
Table 2a shows that small differences in temperature between indoor and outdoor environments can have a large impact on the estimated length of the EIP. With warmer indoor temperatures, parasite development is faster than predicted from ambient conditions, with differences tending to becoming larger at higher altitudes as indoor and outdoor temperatures become more divergent. At the extreme, indoor environmental temperatures can enable parasites to complete incubation at altitudes where outdoor temperatures fall below the threshold for development. Thus, the environmental limits for malaria transmission depend not just on ambient conditions, but also on indoor conditions and the extent of endophily. These patterns are qualitatively similar for both temperature-development models. The patterns also hold up when the influence of diurnal temperature fluctuations is included, although temperature variation makes the differences in growth between indoor and outdoor environments slightly less marked.
The EIP is just one of a range of parameters used to determine R 0 [52]. Assessing the absolute effects of indoor vs outdoor temperatures on R 0 requires estimates of all parameters. However, by taking the simplifying (and conservative) assumption that other parameters remain constant, the relative consequences of changes in parasite development rate on R 0 can be assessed using the relationship between EIP and median daily mosquito survival rate, p, derived from the R 0 equation: pEIP/-lnp [see 12 for more details]. Importantly, adult mosquito survival is largely insensitive to temperature across much of the transmission range, with mortality only increasing markedly as mean temperature exceeds 35-36 °C [1]. Keeping p fixed (in this case a median daily survivorship of p = 0.86, as used elsewhere [56]) enables the relative change in R 0 due to effects of indoor vs. outdoor temperature on EIP to be estimated.
As expected, the shorter EIPs resulting from the warmer conditions indoors than outdoors generate relative increases in R 0 (Table 2b). As differences between indoor and outdoor temperatures increase (e.g. with altitude), so does the relative change in transmission intensity. With the current data set, relative increases in R 0 range from around 5 to approaching 3,000%, depending on the model and exact temperatures used.
Implications of the hypothesis
Use of relative R 0 does not in itself quantify absolute disease risk and as pointed out by Rogers & Randolph [4], even a large increase from a very small initial R 0 will still be a small R 0 . Nonetheless, this analysis clearly reveals that current risk models based on mean outdoor temperatures could be substantially underestimating transmission intensity if mosquitoes spend significant periods of the gonotrophic cycle resting indoors. At higher altitudes, where the differences are greatest, indoor resting may be common as mosquitoes attempt to alleviate the burden of hostile outdoor microclimates [57]. Variation between individual villages indicates that application of a simple temperature correction factor is unlikely to capture the effects across different house structures and local environments.
The effect of the indoor microclimate will also extend to other temperature-dependent life-history characteristics, such as blood-meal digestion and egg production, potentially increasing malaria risk by endophilic mosquitoes even further. Understanding malaria transmission ecology and malaria risk, therefore, requires better awareness of mosquito resting behaviour and the associated microclimate. In this regard, the current study reveals a number of important knowledge gaps:
Where do exophilic mosquitoes rest and what are the exact microclimates?
Searches for outdoor resting mosquitoes have frequently proved time consuming and unrewarding [58]. It seems likely that indoor collections will result in a relatively larger proportion of mosquitoes being caught, even when the outdoor resting population is of the same size or larger. A human dwelling is extremely small compared with its outdoor surroundings and so the size and importance of the exophilic fraction of a population is probably often overlooked [58, 59]. The consequences of a prevailing sampling bias was highlighted recently with the discovery of a previously unknown subgroup of exophilic An. gambiae in Burkina Faso [60].
Outdoor resting mosquitoes seek shelter in a variety of environments, such as under the eaves of huts, in dry pots, canal water pipes, undersides of bridges, at bases of trees, in tree holes, piles of fallen leaves, cracks and crevices of brick pits, cracks and holes in the ground, small ridges under rocks, granaries, etc. [26, 58, 59, 61, 62]. All these sites are likely to be heavily shaded [61], and have their own specific microclimate. Outdoor temperature data, however, are most commonly collected from met-stations, such as Stevenson screens. Whether these met-station data are representative of the outdoor microclimates experienced by mosquitoes is unclear. Studies similar to the one by Meyer et al[63], in which microhabitat temperatures of resting places of Culex tarsalis were monitored in detail in California, USA, are urgently needed for tropical malaria mosquitoes.
Where do endophilic mosquitoes rest and what are the exact microclimates?
Indoor temperature will strongly depend on factors such as season [14, 41, 42], location/altitude [14, 40–42, 50, 54], the nature of the building structure [47, 48, 64], its surroundings [14, 40–42], the number of occupants [44], and whether people burn wood indoors [65]. Additionally, even within a single house there is likely to exist a gradient of temperature microhabitats [66].
There appear few records of exactly where African malaria mosquitoes rest within a house. In Burkina Faso, 95% of the An. gambiae and An. funestus mosquitoes were resting on the ceiling [67]. In South-America, the preferred resting site within houses appears to differ between species and locations. In Columbia it was observed that Anopheles darlingi and Anopheles marajoara tended to rest close to the ground, whereas Anopheles oswaldoi and Anopheles rangeli rested higher up [68]. In Brazil, on the other hand, An. darlingi mosquitoes were mostly collected from the ceiling (59%), with 37% resting on the walls and only 4% on the floor [69]. In Guatemala, the greatest numbers (53%) of Anopheles albimanus were found at the undersurface of shed roofs, the remainder mostly on walls (28%) and furniture (13%) [70].
Similar variation has been observed in Asia for Anopheles culicifacies. In Maharashtra, India, about 70% were found resting on the underside of roofs of village houses while only 30% rested on the vertical walls and surface of furniture, vessels, grain bins, etc. In villages around Delhi, 72% of An. culicifacies were found resting on the ceiling and walls above 1.8 m from the floor [71]. In Sri Lanka, however, it was found that the species preferred to rest on walls below 1.8 m [72]. Whether such behavioural variation is important with respect to temperature, and is possibly even driven by microclimate selection, is uncertain.
Do mosquitoes moderate body temperature via behavioural thermoregulation?
Short-term selection of thermally favoured microclimates, especially of sunny or shaded substrates, is probably the most common mechanism for control of body temperatures in insects [73]. The extent to which adult mosquitoes select habitats based on micro-environment and can behaviourally thermoregulate, however, is unclear. One study examined the escape or avoidance behaviour of An. gambiae and An. arabiensis in response to increasing temperatures and showed An. arabiensis to be slightly more thermally tolerant than An. gambiae[74]. Another study showed that newly-emerged An. gambiae mosquitoes can avoid desiccation by using their thermohygroreceptor cells to guide them to cooler and more humid locations that facilitate survival [75]. These observations are consistent with thermal gradient studies on Anopheles stephensi, which indicated some ability to avoid temperature extremes, although no clear capacity to maintain steady body temperatures by behavioural thermoregulation was observed [76]. Beyond these examples, research investigating whether adult mosquitoes make behavioural choices in response to temperature is limited. Given the potential importance of the behaviour, further studies, including whether malaria parasites have the potential to manipulate mosquito microhabitat selection (cf. [77]), would appear warranted.
What are the implications of changes in resting behaviour due to vector control tools?
The use of insecticides on bed nets (ITNs) [78–81], eaves curtains [82], durable linings [83], or by indoor residual sprays (IRS) [84], all have the potential to keep/drive malaria vectors outdoors. Evolution of such behavioural resistance is a major threat to malaria control as all these front-line tools rely on mosquitoes feeding and/or resting indoors [85, 86]. However, in some settings a switch to actual outdoor resting could result in increases in EIP and a reduction in underlying transmission intensity (Table 2). The extent to which such changes could offset any proximate reductions in control will depend on the specifics of the system. Based on the relative changes in R 0 presented in Table 2, a shift to outdoor resting could reduce transmission intensity many fold and in theory, in areas where the outdoor environment it is too cold for parasites to complete their development within the lifespan of the mosquito, could even lead to local elimination.
The list of questions above highlights many unknowns and sets out numerous research challenges. Nonetheless, given that certain mosquitoes clearly spend part of the gonotrophic cycle indoors and that indoor microclimates do generally differ from outdoor microclimates, it seems important that malaria risk assessments move away from use of mean outdoor temperatures alone. Based on current knowledge it seems unlikely that processed-based models can fully capture the complexities of variable mosquito behaviours across variable environments. However, a useful starting point could be to take the extremes of complete exophily vs complete endophily and use these assumptions to explore the range of transmission intensity possible based on the full extent of microclimates available within a location.
References
Craig MH, Snow RW, Le Sueur D: A climate-based distribution model of malaria transmission in sub-Saharan Africa. Parasitol Today. 1999, 15: 105-111. 10.1016/S0169-4758(99)01396-4.
Harvell CD, Mitchell CE, Ward JR, Altizer S, Dobson AP, Ostfeld RS, Samuel MD: Climate warming and disease risks for terrestrial and marine biota. Science. 2002, 296: 2158-2162. 10.1126/science.1063699.
Patz JA, Olson SH: Malaria risk and temperature: Influences from global climate change and local land use practices. Proc Natl Acad Sci USA. 2006, 103: 5635-5636. 10.1073/pnas.0601493103.
Rogers DJ, Randolph SE: Climate change and vector-borne diseases. Global Mapping of Infectious Diseases: Methods, Examples and Emerging Applications (Advances in Parasitology). Edited by: Hay SI, Graham A, Rogers DJ. 2006, San Diego: Elsevier, 62: 345-381.
Paaijmans KP, Blanford S, Bell AS, Blanford JI, Read AF, Thomas MB: Influence of climate on malaria transmission depends on daily temperature variation. Proc Natl Acad Sci USA. 2010, 107: 15135-15139. 10.1073/pnas.1006422107.
Martens P, Kovats RS, Nijhof S, de Vries P, Livermore MTJ, Bradley DJ, Cox J, McMichael AJ: Climate change and future populations at risk of malaria. Global Environ Change. 1999, 9: S89-S107.
Killeen GF, McKenzie FE, Foy BD, Schieffelin C, Billingsley PF, Beier JC: A simplified model for predicting malaria entomologic inoculation rates based on entomologic and parasitologic parameters relevant to control. Am J Trop Med Hyg. 2000, 62: 535-544.
Rogers DJ, Randolph SE: The global spread of malaria in a future, warmer world. Science. 2000, 289: 1763-1766.
Ebi KL, Hartman J, Chan N, McConnell J, Schlesinger M, Weyant J: Climate suitability for stable malaria transmission in Zimbabwe under different climate change scenarios. Clim Change. 2005, 73: 375-393. 10.1007/s10584-005-6875-2.
Hay SI, Snow RW: The malaria atlas project: Developing global maps of malaria risk. PLoS Med. 2006, 3: 2204-2208.
Guerra CA, Gikandi PW, Tatem AJ, Noor AM, Smith DL, Hay SI, Snow RW: The limits and intensity of Plasmodium falciparum transmission: Implications for malaria control and elimination worldwide. PLoS Med. 2008, 5: 300-311.
Paaijmans KP, Read AF, Thomas MB: Understanding the link between malaria risk and climate. Proc Natl Acad Sci USA. 2009, 106: 13844-13849. 10.1073/pnas.0903423106.
Paaijmans K, Imbahale S, Thomas M, Takken W: Relevant microclimate for determining the development rate of malaria mosquitoes and possible implications of climate change. Malar J. 2010, 9: 196-10.1186/1475-2875-9-196.
Afrane YA, Lawson BW, Githeko AK, Yan G: Effects of microclimatic changes caused by land use and land cover on duration of gonotrophic cycles of Anopheles gambiae (Diptera: Culicidae) in western Kenya highlands. J Med Entomol. 2005, 42: 974-980. 10.1603/0022-2585(2005)042[0974:EOMCCB]2.0.CO;2.
Lardeux FJ, Tejerina RH, Quispe V, Chavez TK: A physiological time analysis of the duration of the gonotrophic cycle of Anopheles pseudopunctipennis and its implications for malaria transmission in Bolivia. Malar J. 2008, 7: 17-10.1186/1475-2875-7-17.
Rúa GL, Quiñones ML, Vélez ID, Zuluaga JS, Rojas W, Poveda G, Ruiz D: Laboratory estimation of the effects of increasing temperatures on the duration of gonotrophic cycle of Anopheles albimanus (Diptera: Culicidae). Mem Inst Oswaldo Cruz. 2005, 100: 515-520. 10.1590/S0074-02762005000500011.
Sumba LA, Okoth K, Deng AL, Githure J, Knols BGJ, Beier JC, Hassanali A: Daily oviposition patterns of the African malaria mosquito Anopheles gambiae Giles (Diptera: Culicidae) on different types of aqueous substrates. Journal of Circadian Rhythms. 2004, 2: 6-10.1186/1740-3391-2-6.
Fritz ML, Huang J, Walker ED, Bayoh MN, Vulule J, Miller JR: Ovipositional periodicity of caged Anopheles gambiae individuals. Journal of Circadian Rhythms. 2008, 6: 2-10.1186/1740-3391-6-2.
Geissbühler Y, Chaki P, Emidi B, Govella NJ, Shirima R, Mayagaya V, Mtasiwa D, Mshinda H, Fillinger U, Lindsay SW, Kannady K, de Castro MC, Tanner M, F Killeen G: Interdependence of domestic malaria prevention measures and mosquito-human interactions in urban Dar es Salaam, Tanzania. Malar J. 2007, 6: 126-10.1186/1475-2875-6-126.
Oyewole IO, Awolola TS, Ibidapo CA, Oduola AO, Okwa OO, Obansa JA: Behaviour and population dynamics of the major anopheline vectors in a malaria endemic area in southern Nigeria. J Vector Borne Dis. 2007, 44: 56-64.
Shililu J, Ghebremeskel T, Seulu F, Mengistu S, Fekadu H, Zerom M, Asmelash GE, Sintasath D, Mbogo C, Githure J, Brantly E, Beier JC, Novak RJ: Seasonal abundance, vector behavior, and malaria parasite transmission in Eritrea. J Am Mosq Control Assoc. 2004, 20: 155-164.
Pates H, Curtis C: Mosquito behavior and vector control. Annu Rev Entomol. 2005, 50: 53-70. 10.1146/annurev.ento.50.071803.130439.
Service MW: Ecological notes on species A and B of the Anopheles gambiae complex in the Kisumu area of Kenya. Bull Entomol Res. 1970, 60: 105-108. 10.1017/S0007485300034192.
Highton RB, Bryan JH, Boreham PFL, Chandler JA: Studies on the sibling species Anopheles gambiae Giles and Anopheles arabiensis Patton (Diptera: Culicidae) in the Kisumu area, Kenya. Bull Entomol Res. 1979, 69: 43-53. 10.1017/S0007485300017879.
Mnzava AEP, Rwegoshora RT, Wilkes TJ, Tanner M, Curtis CF: Anopheles arabiensis and Anopheles gambiae chromosomal inversion polymorphism, feeding and resting behavior in relation to insecticide house spraying in Tanzania. Med Vet Entomol. 1995, 9: 316-324. 10.1111/j.1365-2915.1995.tb00140.x.
Githeko AK, Service MW, Mbogo CM, Atieli FK: Resting behaviour, ecology and genetics of malaria vectors in large scale agricultural areas of western Kenya. Parassitologia. 1996, 38: 481-489.
Faye O, Konate L, Mouchet J, Fontenille D, Sy N, Hebrard G, Herve JP: Indoor resting by outdoor biting females of Anopheles gambiae complex (Diptera: Culicidae) in the sahel of northern Senegal. J Med Entomol. 1997, 34: 285-289.
Bockarie MJ, Service MW, Barnish G, Maude GH, Greenwood BM: Malaria in a rural area of Sierra Leone. III. Vector ecology and disease transmission. Ann Trop Med Parasitol. 1994, 88: 251-262.
Mahande A, Mosha F, Mahande J, Kweka E: Feeding and resting behaviour of malaria vector, Anopheles arabiensis with reference to zooprophylaxis. Malar J. 2007, 6: 100-10.1186/1475-2875-6-100.
Gillies MT: Studies in house leaving and outside resting of Anopheles gambiae Giles and Anopheles funestus Giles in East Africa. II The exodus from houses and the house resting population. Bull Entomol Res. 1954, 45: 375-387. 10.1017/S000748530002719X.
Clarke JL, Pradhan GD, Joshi GP, Fontaine RE: Assessment of the grain store as an unbaited outdoor shelter for mosquitoes of the Anopheles gambiae complex and Anopheles funestus (Diptera: Culicidae) at Kisumu, Kenya. J Med Entomol. 1980, 17: 100-102.
Aniedu I: Biting activity and resting habits of malaria vectors in Baringo district, Kenya. Anz Schädlingskunde. 1993, 66: 72-76.
Rajaonarivelo V, Le Goff G, Cot M, Brutus L: Anophelines and malaria transmission in Ambohimena, a village of the occidental fringe of Madagascar Highlands. Parasite. 2004, 11: 75-82.
Fontenille D, Lepers JP, Campbell GH, Coluzzi M, Rakotoarivony I, Coulanges P: Malaria transmission and vector biology in Manarintsoa, high plateaux of Madagascar. Am J Trop Med Hyg. 1990, 43: 107-115.
Randrianasolo BOR, Coluzzi M: Genetical investigations on zoophilic and exophilic Anopheles arabiensis from Antananarivo area (Madagascar). Parassitologia. 1987, 29: 93-97.
Tirados I, Costantini C, Gibson G, Torr SJ: Blood-feeding behaviour of the malarial mosquito Anopheles arabiensis: implications for vector control. Med Vet Entomol. 2006, 20: 425-437. 10.1111/j.1365-2915.2006.652.x.
Dukeen MYH, Omer SM: Ecology of the malaria vector Anopheles arabiensis Patton (Diptera: Culicidae) by the Nile in northern Sudan. Bull Entomol Res. 1986, 76: 451-467. 10.1017/S0007485300014942.
Ameneshewa B, Service MW: Resting habits of Anopheles arabiensis in the Awash River Valley of Ethiopia. Ann Trop Med Parasitol. 1996, 90: 515-521.
Lines JD, Lyimo EO, Curtis CF: Mixing of indoor- and outdoor-resting adults of Anopheles gambiae Giles s.l. and Anopheles funestus Giles (Diptera: Culicidae) in Coastal Tanzania. Bull Entomol Res. 1986, 76: 171-178. 10.1017/S0007485300015388.
Afrane YA, Little TJ, Lawson BW, Githeko AK, Yan GY: Deforestation and vectorial capacity of Anopheles gambiae giles mosquitoes in malaria transmission, Kenya. Emerg Infect Dis. 2008, 14: 1533-1538. 10.3201/eid1410.070781.
Afrane YA, Zhou G, Lawson BW, Githeko AK, Yan G: Effects of microclimatic changes caused by deforestation on the survivorship and reproductive fitness of Anopheles gambiae in western Kenya highlands. Am J Trop Med Hyg. 2006, 74: 772-778.
Afrane YA, Zhou G, Lawson BW, Githeko AK, Yan G: Life-table analysis of Anopheles arabiensis in western Kenya highlands: Effects of land covers on larval and adult survivorship. Am J Trop Med Hyg. 2007, 77: 660-666.
Garnham P: Malaria epidemics at exceptionally high altitudes in Kenya. Br Med Bull. 1945, 2: 456-457.
Garnham P: The incidence of malaria at high altitudes. J Nat Mal Soc. 1948, 7: 275-284.
Haddow AJ: The mosquito fauna and climate of native huts at Kisumu, Kenya. Bull Entomol Res. 1942, 33: 91-142. 10.1017/S0007485300026389.
Pascual M, Dobson AP, Bouma MJ: Underestimating malaria risk under variable temperatures. Proc Natl Acad Sci USA. 2009, 106: 13645-13646. 10.1073/pnas.0906909106.
Okech BA, Gouagna LC, Knols BGJ, Kabiru EW, Killeen GF, Beier JC, Yan G, Githure JI: Influence of indoor microclimate and diet on survival of Anopheles gambiae s.s. (Diptera: Culicidae) in village house conditions in western Kenya. Int J Trop Insect Sci. 2004, 24: 207-212.
Okech BA, Gouagna LC, Walczak E, Kabiru EW, Beier JC, Yan G, Githure JI: The development of Plasmodium falciparum in experimentally infected Anopheles gambiae (Diptera: Culicidae) under ambient microhabitat temperature in western Kenya. Acta Trop. 2004, 92: 99-108. 10.1016/j.actatropica.2004.06.003.
Shanks GD, Hay SI, Stern DI, Biomndo K, Snow RW: Meteorologic influences on Plasmodium falciparum malaria in the highland tea estates of Kericho, western Kenya. Emerg Infect Dis. 2002, 8: 1404-1408.
Bødker R, Akida J, Shayo D, Kisinza W, Msangeni HA, Pedersen EM, Lindsay SW: Relationship between altitude and intensity of malaria transmission in the Usambara Mountains, Tanzania. J Med Entomol. 2003, 40: 706-717. 10.1603/0022-2585-40.5.706.
Worrall E, Connor SJ, Thomson MC: A model to simulate the impact of timing, coverage and transmission intensity on the effectiveness of indoor residual spraying (IRS) for malaria control. Trop Med Int Health. 2007, 12: 75-88.
MacDonald G: The epidemiology and control of malaria. 1957, London: Oxford University Press
Detinova TS: Age-grouping Methods in Diptera of Medical Importance. 1962, Geneva: World Health Organization
Minakawa N, Omukunda E, Zhou G, Githeko A, Yan G: Malaria vector productivity in relation to the highland environment in Kenya. Am J Trop Med Hyg. 2006, 75: 448-453.
Parton WJ, Logan JA: A model for diurnal variation in soil and air temperature. Agr Met. 1981, 23: 205-216.
Kiszewski A, Mellinger A, Spielman A, Malaney P, Sachs SE, Sachs J: A global index representing the stability of malaria transmission. Am J Trop Med Hyg. 2004, 70: 486-498.
Tchuinkam T, Simard F, Lele-Defo E, Tene-Fossog B, Tateng-Ngouateu A, Antonio-Nkondjio C, Mpoame M, Toto JC, Njine T, Fontenille D, Awono-Ambene HP: Bionomics of Anopheline species and malaria transmission dynamics along an altitudinal transect in Western Cameroon. BMC Infect Dis. 2010, 10: 12-10.1186/1471-2334-10-12.
Service MW: Mosquito ecology Field sampling methods. 1993, London: Elsevier Applied Science, 2
Muirhead Thomson RC: Mosquito behaviour in relation to malaria transmission and control in the tropics. 1951, London: Arnold
Riehle MM, Guelbeogo WM, Gneme A, Eiglmeier K, Holm I, Bischoff E, Garnier T, Snyder GM, Li X, Markianos K, Sagnon N, Vernick KD: A cryptic subgroup of Anopheles gambiae is highly susceptible to human malaria parasites. Science. 2011, 331: 596-598. 10.1126/science.1196759.
Haridi AM: Partial exophily of Anopheles gambiae species B in the Khashm Elgirba area in eastern Sudan. Bull World Health Organ. 1972, 46: 39-42.
Service MW: The ecology of the mosquitos of the Northern Guinea Savannah of Nigeria. Bull Entomol Res. 1963, 54: 601-632. 10.1017/S000748530004904X.
Meyer RP, Hardy JL, Reisen WK: Diel changes in adult mosquito microhabitat temperatures and their relationship to the extrinsic incubation of arboviruses in mosquitoes in Kern county, California. J Med Entomol. 1990, 27: 607-614.
Atieli H, Menya D, Githeko A, Scott T: House design modifications reduce indoor resting malaria vector densities in rice irrigation scheme area in western Kenya. Malar J. 2009, 8: 9-10.1186/1475-2875-8-9.
Bockarie MJ, Service MW, Barnish G, Momoh W, Salia F: The effect of woodsmoke on the feeding and resting behaviour of Anopheles gambiae s.s. Acta Trop. 1994, 57: 337-340. 10.1016/0001-706X(94)90080-9.
Okech BA, Gouagna LC, Killeen GF, Knols BGJ, Kabiru EW, Beier JC, Yan G, Githure JI: Influence of sugar availability and indoor microclimate on survival of Anopheles gambiae (Diptera: Culicidae) under semifield conditions in western Kenya. J Med Entomol. 2003, 40: 657-663. 10.1603/0022-2585-40.5.657.
Mathis W, Hamon J, Stcloud A, Eyraud M, Miller S: Initial field studies in Upper Volta with dichlorvos residual fumigant as a malaria eradication technique. 2. Entomological evaluation. Bull World Health Organ. 1963, 29: 237-240.
Quinõnes M, Suarez M: Indoor resting heights of some anophelines in Columbia. J Am Mosq Control Assoc. 1990, 6: 602-605.
Roberts D, Alecrim W, Tavares A, Radke M: The house-frequenting, host-seeking and resting behavior of Anopheles darlingi in southeastern Amazonas, Brazil. J Am Mosq Control Assoc. 1987, 3: 433-441.
Ogata K, Ikeda T, Umino T, Bocanegra R: Observations of biting and resting behavior of Anopheles albimanus in Guatemala. Jpn J Sanit Zool. 1992, 43: 47-57.
Bhatia M, Wattal B, Mammen M: Preferential indoor resting habits of Anopheles culicifacies Giles, near Delhi. Indian J Malariol. 1957, 11: 61-71.
Rao T: The Anophelines of India. 1984, New Delhi, India: Malaria Research Centre (Indian Council of Medical Research)
May ML: Insect thermoregulation. Annu Rev Entomol. 1979, 24: 313-349. 10.1146/annurev.en.24.010179.001525.
Kirby MJ, Lindsay SW: Responses of adult mosquitoes of two sibling species, Anopheles arabiensis and A. gambiae s.s. (Diptera: Culicidae), to high temperatures. Bull Entomol Res. 2004, 94: 441-448.
Kessler S, Guerin PM: Responses of Anopheles gambiae, Anopheles stephensi, Aedes aegypti, and Culex pipiens mosquitoes (Diptera: Culicidae) to cool and humid refugium conditions. J Vector Ecol. 2008, 33: 145-149. 10.3376/1081-1710(2008)33[145:ROAGAS]2.0.CO;2.
Blanford S, Read A, Thomas M: Thermal behaviour of Anopheles stephensi in response to infection with malaria and fungal entomopathogens. Malar J. 2009, 8: 72-10.1186/1475-2875-8-72.
Fialho RF, Schall JJ: Thermal ecology of a malarial parasite and its insect vector: Consequences for the parasite's transmission success. J Anim Ecol. 1995, 64: 553-562. 10.2307/5799.
Lindsay SW, Adiamah JH, Miller JE, Armstrong JRM: Pyrethroid-treated bednet effects on mosquitoes of the Anopheles gambiae complex in The Gambia. Med Vet Entomol. 1991, 5: 477-483. 10.1111/j.1365-2915.1991.tb00576.x.
Miller JE, Lindsay SW, Armstrong JRM: Experimental hut trials of bednets impregnated with synthetic pyrethroid or organophosphate insecticide for mosquito control in The Gambia. Med Vet Entomol. 1991, 5: 465-476. 10.1111/j.1365-2915.1991.tb00575.x.
Mbogo CNM, Baya NM, Ofulla AVO, Githure JI, Snow RW: The impact of permethrin-impregnated bednets on malaria vectors of the Kenyan coast. Med Vet Entomol. 1996, 10: 251-259. 10.1111/j.1365-2915.1996.tb00739.x.
Lines JD, Myamba J, Curtis CF: Experimental hut trials of permethrin-impregnated mosquito nets and eave curtains against malaria vectors in Tanzania. Med Vet Entomol. 1987, 1: 37-51. 10.1111/j.1365-2915.1987.tb00321.x.
Githeko AK, Adungo NI, Karanja DM, Hawley WA, Vulule JM, Seroney IK, Ofulla AVO, Atieli FK, Ondijo SO, Genge IO, Odada PK, Situbi PA, Oloo JA: Some observations on the biting behavior of Anopheles gambiae s.s., Anopheles arabiensis, and Anopheles funestus and their implications for malaria control. Exp Parasitol. 1996, 82: 306-315. 10.1006/expr.1996.0038.
Chandre F, Dabire R, Hougard JM, Djogbenou L, Irish S, Rowland M, N'Guessan R: Field efficacy of pyrethroid treated plastic sheeting (durable lining) in combination with long lasting insecticidal nets against malaria vectors. Parasites & Vectors. 2010, 3: 65-10.1186/1756-3305-3-65.
Service MW, Joshi GP, Pradhan GD: Survey of Anopheles gambiae (species A) and An. arabiensis (species B) of An. gambiae Giles complex in the Kisumu area of Kenya following insecticidal spraying with OMS-43 (Fenitrothion). Ann Trop Med Parasitol. 1978, 72: 377-386.
Ranson H, N'Guessan R, Lines J, Moiroux N, Nkuni Z, Corbel V: Pyrethroid resistance in African anopheline mosquitoes: what are the implications for malaria control?. Trends Parasitol. 2011, 27: 91-98. 10.1016/j.pt.2010.08.004.
The malERA Consultative Group on Vector Control: A research agenda for malaria eradication: vector control. PLoS Med. 2011, 8: e1000401-
Acknowledgements and funding
This study was supported by a NSF-EID program grant (#EF-0914384) and NIH-NIAID ICEMR award (#U19AI089676-01).
Author information
Authors and Affiliations
Corresponding author
Additional information
Competing interests
The authors declare that they have no competing interests.
Authors' contributions
KPP and MBT both contributed to developing and writing the manuscript. Both authors read and approved the final manuscript.
Authors’ original submitted files for images
Below are the links to the authors’ original submitted files for images.
Rights and permissions
This article is published under license to BioMed Central Ltd. This is an Open Access article distributed under the terms of the Creative Commons Attribution License (http://creativecommons.org/licenses/by/2.0), which permits unrestricted use, distribution, and reproduction in any medium, provided the original work is properly cited.
About this article
Cite this article
Paaijmans, K.P., Thomas, M.B. The influence of mosquito resting behaviour and associated microclimate for malaria risk. Malar J 10, 183 (2011). https://doi.org/10.1186/1475-2875-10-183
Received:
Accepted:
Published:
DOI: https://doi.org/10.1186/1475-2875-10-183