Abstract
Background
The dysregulation of gene expression in the TNF-TNFR superfamily has been involved in various human cancers including non-small cell lung cancer (NSCLC). Furthermore, functional polymorphisms in TNF-α and TNFRSF1B genes that alter gene expression are likely to be associated with risk and clinical outcomes of cancers. However, few reported studies have investigated the association between potentially functional SNPs in both TNF-α and TNFRSF1B and prognosis of NSCLC patients treated with chemoradiotherapy.
Methods
We genotyped five potentially functional polymorphisms of TNF-α and TNFRSF1B genes [TNF-α -308 G>A (rs1800629) and -1031 T>C (rs1799964); TNFRSF1B +676 T>G (rs1061622), -1709A>T(rs652625) and +1663A>G (rs1061624)] in 225 NSCLC patients treated with chemoradiotherapy or radiotherapy alone. Kaplan-Meier survival analysis, log-rank tests and Cox proportional hazard models were used to evaluate associations between these variants and NSCLC overall survival (OS).
Results
We found that the TNFRSF1B +676 GG genotype was associated with a significantly better OS of NSCLC (GG vs. TT: adjusted HR = 0.38, 95% CI = 0.15-0.94; GG vs. GT/TT: adjusted HR = 0.35, 95% CI = 0.14-0.88). Further stepwise multivariate Cox regression analysis showed that the TNFRSF1B +676 GG was an independent prognosis predictor in this NSCLC cohort (GG vs. GT/TT: HR = 0.35, 95% CI = 0.14-0.85), in the presence of node status (N2-3 vs. N0-1: HR = 1.60, 95% CI = 1.09-2.35) and tumor stage (T3-4 vs. T0-2: HR = 1.48, 95% CI = 1.08-2.03).
Conclusions
Although the exact biological function for this SNP remains to be explored, our findings suggest a possible role of TNFRSF1B +676 T>G (rs1061622) in the prognosis of NSCLC. Further large and functional studies are needed to confirm our findings.
Similar content being viewed by others
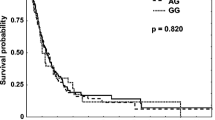
Background
Lung cancer is the most common tobacco-induced cancer and the leading cause of cancer-related deaths worldwide, with an estimated 1.61 million new cases and 1.38 million deaths in 2008 [1]. About 80% of primary lung cancer patients are non-small cell lung cancer (NSCLC), and one third of the patients were diagnosed at a locally advanced stage [2]. Despite significant advances in early detection and combination treatment including radiotherapy and chemotherapy in the last few decades, the prognosis of lung cancer remains poor, with a five-year overall survival rate of about 15% in the United States [3]. The tumor, lymph node, metastasis (TNM) staging system of lung cancer has been used as a guide for predicting prognosis [4]; however, dramatically different survival outcomes in NSCLC patients with the same pathological or clinical stage and the same treatments suggest that other factors may play an important role in the prognosis of NSCLC. Therefore, the discovery and application of novel prognostic biomarkers could help predict clinical outcomes and administer the optimal therapy in the management of NSCLC patients.
Tumor necrosis factor alpha (TNF-α) is a pro-inflammatory cytokine produced by activated macrophages and exerts its action through binding to its two cognate cell surface receptors, TNFRSF1A/TNFR1 (p55/60) and TNFRSF1B/TNFR2 (p75/80). It is well known that TNF and its superfamily members have both beneficial and harmful activities, playing a role as a "double-edged sword" [5]. Although TNF was discovered as a cytokine that could kill tumor cells, it is now clear that TNF can also contribute to tumorigenesis by mediating the proliferation, invasion and metastasis of tumor cells [5]. The dysregulation of gene expression in the TNF-TNFR superfamily has been reported to be involved in the development and prognosis of various human cancers including NSCLC [6–12]. For example, studies indicated that high serum concentrations of TNF were associated with a significantly longer survival in NSCLC patients after chemotherapy [12] and that TNFRSF1B had a significantly different expression profile in 5-FU-non-responding and responding liver cancer patients [11]. Additionally, recent reports found that TNF-α was involved in the pathogenesis of radiation-induced lung injury [13] and that inhibiting the TNF-α pathway was a novel radioprotection strategy [14]. These observations suggest that TNF and TNFRSF1B may play a role in patients' treatment response, toxicity, and survival. Thus, genetic variations in TNF and TNFRSF1B that alter gene expression and/or protein production may be potential candidates for prognosis predictors of NSCLC patients.
TNF-α and TNFRSF1B genes are highly polymorphic, and several functional single nucleotide polymorphisms (SNPs) in these two genes have been identified, which may contribute to differences in expression levels of the genes or protein products [15–20]. Of a particular significance are two TNF-α SNPs (SNP -308 G>A and -1031 T>C in the promoter region) and one TNFRSF1B SNP (+676 T>G in exon 6), which have been widely investigated for their associations with susceptibility to and progression and prognosis of various cancers [21–37]. However, to the best of our knowledge, no published study has investigated associations between potentially functional SNPs of these two genes and prognosis of NSCLC patients treated with chemoradiotherapy. Therefore, we performed a case-only study with 225 NSCLC patients treated with chemoradiotherapy or radiotherapy alone to investigate whether these three SNPs (SNP -308 G>A and -1031 T>C in TNF-α, and +676 T>G) as well as the other two potentially functional SNPs (-1709A>T and +1663A>G in TNFRSF1B) are associated with overall survival of NSCLC.
Methods
Study population
Epidemiological and clinical data were available from a larger dataset of 576 NSCLC patients who were treated with definitive radiation at The University of Texas M.D. Anderson Cancer Center (Houston, TX) between 1998 and 2006. The detailed information about these subjects has been described elsewhere [38]. After the exclusion of those patients who had surgical resection or had been treated elsewhere before coming to M. D. Anderson, a total of 225 NSCLC patients treated with chemoradiotherapy or radiotherapy alone were included in this analysis; The study was approved by the M. D. Anderson Cancer Center institutional review board in compliance with Health Insurance Portability and Accountability Act (HIPAA) regulations.
SNP selection
We screened the NCBI dbSNP database (http://www.ncbi.nlm.nih.gov/, build 131) and Hapmap database (http://www.hapmap.org/, Rel 27) for common, potentially functional SNPs with a minor allele frequency (MAF) ≥ 0.05 in CEU populations, i.e., those SNPs located in the 5' near gene, 5'- and 3'-untranslated regions and coding region of TNF-α and TNFRSF1B. Meanwhile, linkage disequilibrium (LD) analysis and bioinformatics prediction by SNPinfo (http://snpinfo.niehs.nih.gov/) [39] were also performed to optimize SNP selection. Finally, a total of five SNPs (TNF-α -308 G>A, TNF-α -1031 T>C, and TNFRSF1B +676 T>G, TNFRSF1B -1709A>T and TNFRSF1B+1663A>G) were selected for genotyping. Among these, three SNPs are located in the promoter regions of the genes (TNF-α -308 G>A, TNF-α -1031 T>C, and TNFRSF1B -1709A>T), which are predicted to affect the binding of some transcription factors. One SNP (TNFRSF1B +676 T>G) causes a missense change and the other (TNFRSF1B +1663A>G) is located in the 3'-untranslated region, which may affect miRNA binding.
Genotyping
Genomic DNA was isolated from the buffy coat fraction of each blood sample with a Blood Mini Kit (Qiagen, Valencia, CA) according to the manufacturer's instructions. Genotypes of the three selected SNPs (SNP -308 G>A and -1031 C>T in TNF-α, and -1709A>T in TNFRSF1B ) were determined by the primer-introduced restriction analysis (PIRA)-PCR assay. The primers used for TNF-α -308 G >A, TNF-α -1031 T>C and TNFRSF1B -1709A>T were 5'-GCAATAGGTTTTGAGGGCCATG-3' (sense) and 5'-TTTGGAAAGTTGGGGACACACA-3'(anti-sense); 5'-GGGAAGCAAAGGAGAAGCTGAGAACA-3' (sense) and 5'-GGGGGGTCCCCATACTCGACTT-3' (anti-sense); and 5'-CCACACACCTCAAGACCAATGAG-3' (sense) and 5'-CTTGAATTCGTTCCCAGGATGG-3' (anti-sense), respectively. The mismatched G, C and A were respectively introduced into the sense primers at 6 bp, 2 bp and 2 bp from these three polymorphic sites to create NcoI, NlaIII and MlyI restriction sites. Additionally, TNFRSF1B +676T>G and +1663A>G were genotyped by the polymerase chain reaction-restriction fragment length polymorphism (PCR-RFLP) method with the primers 5'-CTCCTCCTCCAGCTGTAACG-3' (sense) and 5'-CCAACTGGAAGAGCGAAGTC-3' (anti-sense), and 5'-AGGCCCCCACCACTAGGACTCT-3' (sense) and 5'-GTTGTGGAAAGCCTCTGCTGC-3' (anti-sense), respectively. The following PCR conditions were performed: 5 min of initial denaturation at 95°C followed by 35 cycles of 30 s at 95°C; 45 s at 64°C (TNF-α -308 G >A) or 59°C (TNF-α -1031 C>T) or 64°C (TNFRSF1B +676 T>G) or 59°C (TNFRSF1B -1709A>T) or 62°C (TNFRSF1B +1663A>G), respectively; 45 s at 72°C; and a final 10-min step at 72°C for final extension.
All the PCR products were digested with the restriction enzymes overnight (NcoI for TNF-α -308 G >A, NlaIII for TNF-α -1031 C>T and TNFRSF1B +676 T>G, and MlyI for TNFRSF1B -1709A>T) and MspA1I for TNFRSF1B (+1663A>G), and the products were separated in 3% MetaPhor agarose gel. After completion of gel electrophoresis, the TNF-α -308 GG genotype produced two bands (127- and 18-bp), AA produced a single band (145-bp), and AG displayed all three bands (145-, 127-, and 18-bp) (Figure 1A); the TNF-α -1031 CC genotype resulted in two bands of 108- and 86-bp, TT produced three bands of 108-, 62- and 24-bp, and CT produced four bands (108-, 86-, 62- and 24-bp) (Figure 1B); the TNFRSF1B +676 TT genotype produced two bands (200- and 48-bp), GG produced a single band (248-bp), and TG displayed all three bands (248-, 200- and 48-bp) (Figure 1C); TNFRSF1B -1709 TT genotype produced two bands of 136- and 24-bp, AA resulted in a single 160-bp band, and TA displayed all three bands (160-, 136- and 24-bp) (Figure 1D); and TNFRSF1B +1663AA resulted in a single 128-bp band, GG genotype produced another single band of 104-bp, and GA displayed all two bands (128-, and 104-bp) (Figure 1E)
Genotyping was performed without knowing the subjects' disease status. Two research assistants independently read the gel pictures and performed the repeated assays, if they did not reach a consensus on the tested genotype. About 10% of the samples were randomly selected to perform the repeated assays, and the results were 100% concordant.
Statistical analysis
The two-sided χ2 tests were performed to determine any statistically significant differences in the distributions of the TNF-α and TNFRSF1B genotypes by demographic variables and clinical features. Median survival time (MST) was calculated, and mean survival time was presented when the MST could not be calculated. Kaplan-Meier estimates were used to estimate overall survival (OS) among four genotype groups, and the log-rank test was used to test for equality of the survival distributions. Univariate analysis and multivariate Cox proportional hazards models were conducted to estimate the effect of each genotype on survival with or without the presence of known prognostic factors. Cox stepwise hazards regression model was also conducted to determine independently predictors of NSCLC prognosis, with a significance level of 0.050 for entering and 0.051 for removing the respective explanatory variables. Haplotype frequencies and individual haplotypes based on the observed genotypes were generated by using SAS PROC HAPLOTYPE. Analyses were performed by using SAS statistical package version 9.1 (SAS Institute Inc., Cary, NC).
Results
Patient characteristics and clinical features
As shown in Table 1, 225 patients were included in the final analyses, of which 155 deaths were observed during the follow-up period, and the overall MST was 23 months. Among the cases, 79 (35.1%) were adenocarcinoma, 71 (31.6) squamous cell carcinoma, and 75 (33.3%) other type of NSCLC. According to the AJCC 6th edition stage grouping criteria [40], 33 cases (14.7%) were stage I-II, and 192 (85.3%) were stage III-IV. In this study, all cases received radiotherapy with a median radiation dose of 63.0 (range 50.4-84.0) Gy at 1.8 to 2 Gy per fraction once a day. Platinum and taxane-based chemotherapy was also given to 163 (72.4%) patients. None of the patients received surgery. Log-rank test showed that, in this study population, there were no significant differences in NSCLC-specific survival by age (log-rank P = 0.117), ethnicity (P = 0.827), smoking status (P = 0.457), histology (P = 0.092), Karnofsky's performance scores (KPS, P = 0.405), chemotherapy status (P = 0.407) and radiotherapy dose (P = 0.706). However, sex, tumor stage, and node status were significantly associated with MST of NSCLC (log-rank P = 0.036, 0.010 and 0.023, respectively). Because these variables may be confounding factors for the effect of the genotypes on OS, they were further adjusted in the multivariable analysis.
TNF-α and TNFRSF1Bgenotypes and NSCLC survival
The genotype distributions of the five SNPs in TNF-α and TNFRSF1B and their associations with OS are summarized in Table 2. In all patients, only genotypes of TNFRSF1B +676 G>T (rs1061622) were statistically significantly associated with OS (log-rank P = 0.040 in an additive model, Figure 2A; log-rank P = 0.014 in a recessive model, Figure 2B). After adjustment for age, sex, ethnicity, smoking status, tumor histology, Karnofsky's performance scores, tumor stage, application of chemotherapy and radiotherapy dose, the TNFRSF1B +676 GG variant homozygous genotype remained to be associated with a significantly decreased risk of death from NSCLC (adjusted HR = 0.38, 95% CI = 0.15-0.94 for GG vs. TT; adjusted HR = 0.35, 95% CI = 0.14-0.88 for GG vs. GT/TT), but this association was not observed for the other four SNPs investigated in this study.
In order to confirm an independent role of SNPs in NSCLC survival, we further performed a multivariate stepwise analysis with selected demographic characteristics and clinical features in different genetic models of TNFRSF1B +676 T>G (rs1061622) on NSCLC survival. Three variables (tumor stage, node status and rs1061622 GG vs. GT/TT) were identified in the regression model with a significance level of 0.050 for entering and 0.051 for removing a variable (Table 3), suggesting the independent effect of these three factors on death risk of NSCLC. When age and sex were forced into the final model, the rs1061622 GG genotype remained a significantly favorable predictor for NSCLC survival (GG vs. GT/TT: HR = 0.39, 95% CI = 0.16-0.95) (Table 3).
Stratification analysis and haplotype analysis
As shown in Figure 3A-E, we further evaluated cumulative survival in subgroups by TNFRSF1B +676 T>G (rs1061622) genotypes and selected factors including age, ethnicity, tumor stage and node status. The longest survival was observed in subgroups with both rs1061622 GG and age≤62 years (MST = 44.0 months), or white ethnicity (MST = 48.3 months), or T0-2 tumors (MST = 48.3 months), or N2-3 tumors (MST = 44.0 months), compared with the poorest survival in subgroups with both rs1061622 GT/TT and age>62 years (MST = 20.7 months), or non-white (MST = 21.0 months), or T3-4 tumors (MST = 18.2 months), or N0-1 tumors (MST = 29.2 months); however, small sample size in these subgroups might have led to a suboptimal statistical power to detect a statistical difference in the survival time. We also conducted the stratification analysis by histology and different treatments, but the numbers of patients in subgroups were too small, and no significant results were found in every stratum (data not shown).
In addition, we also evaluated the combined effect of these polymorphisms on NSCLC survival by using the haplotype analysis. The results showed that TNF-α -308G/-1031T (GT) and TNFRSF1B +676T/+1663A/-1709A (TAA) were the most common haplotypes in the patients with the frequencies of 63.3% and 40.2%, respectively. However, we did not found significant associations between other haplotypes and OS of NSCLC patients, compared to the most common haplotypes (data not shown), which is likely due to limited study power.
Discussion
In the present study, we examined the effect of five selected polymorphisms in TNF-α and TNFRSF1B on survival of NSCLC patients treated with chemoradiotherapy or radiotherapy alone. We found that the TNFRSF1B +676 GG (rs1061622) variant homozygous genotype was associated with a significantly improved survival of NSCLC in this non-Hispanic patient population. Such an effect was not observed for other SPNs under investigation.
Tumor necrosis factor-alpha (TNF-α) executes multiple functions in immunity, inflammation, differentiation, control of cell proliferation, and apoptosis through distinct receptors known as TNF receptor type I (TNFR1) and type II (TNFR2) [5]. Expression levels of TNF-α and its receptors have been linked to development and treatment outcomes of solid tumors including NSCLC [6, 7, 10]. The expression of TNF-α is mostly regulated at the transcriptional level, and polymorphisms within the TNF-α promoter have been related to TNF-α levels [15, 23]. For example, TNF-α-308 G/A in the promoter of TNF-α has been reported to be associated with higher expression levels of TNF-α [41] and thus susceptibility to numerous cancers, including cancers of the stomach [25], breasts [34], oral cavity [31, 32], bladder [35] and lung [25]. Additionally, several studies also found that TNF-α-308 G/A and another SNP in the promoter of TNF-α (-1031C>T, rs1799964) were significantly associated with prognosis of bladder cancer and breast cancer [28, 29, 36], clinical characteristics of colorectal cancer, and severity of lung cancer [25]. However, no significant associations between these two SNPs and NSCLC survival were found in our study, which was consistent with recent data that suggested the lack of associations between TNF-α-308 G/A or TNF-α-1031 T>C and the prognosis of several other cancers, including gastric cancer [42], colorectal cancer [43], Hodgkin's lymphoma [44], and breast cancer [45]. Such a discrepancy of reported studies might be explained by different tumor sites and tumor progression features, ethnic difference with diverse genetic background, different therapeutic strategies, and either false negative or false positive results because of the small sample sizes in previously published studies.
Interestingly, logistic and stepwise regression analysis in our study indicated that TNFRSF1B rs1061622 GG might be an independent predictor for survival of NSCLC patients treated with chemoradiotherapy or radiotherapy alone. The TNFRSF1B is the principal TNF receptor found on circulating T lymphocytes, which functions both as a TNF antagonist by neutralizing it and as an agonist by facilitating interaction between TNF and TNFR1 at the cell surface [46]. rs1061622 is located in the exon 6 of TNFRSF1B (M196R) that causes a functional amino acid change from methionine (M) to arginine (R) [20], which is postulated to affect the proteolytic cleavage of the membrane bound TNFRSF1B to a soluble form as well as TNF binding and/or TNF induced apoptosis by impaired NF-κB signaling [47, 48].
However, the results from published studies on associations between rs1061622 and prognosis of cancers were controversial rather than conclusive. For example, a Tunisian study showed significant associations of TNFRSF1B +676 T>G (rs1061622) with susceptibility and survival of breast cancer patients [26], but another Japanese study suggested no association between this SNP and prognosis of esophageal squamous cell carcinoma [49]. Up to now, no published study investigated the association between TNFRSF1B +676 T>G (rs1061622) and lung cancer survival, and the exact biological mechanism underlying this association in our study remains to be investigated. It is possible that this SNP affects the function of TNFRSF1B and enhances radiotherapy or chemotherapy efficacy through inhibiting tumor therapy resistance. Another possibility is that this SNP may change the expression level of TNFRSF1B and influence the character of immunological response to infection in lung cancer patients, finally resulting in the difference of survival in NSCLC [50]. Furthermore, we also found that cumulative survival was different in subgroups by TNFRSF1B +676 T>G (rs1061622) genotypes and selected factors including age, ethnicity, tumor stage and node status. Although our sample size was relatively small in these subgroups, it provided a clue that effect of this SNP on prognosis of NSCLC may be dependent on patients' characteristics and clinical features as well. However, the exact functional relevance of this SNP in the prognosis of NSCLC patients treated with chemoradiotherapy remains to be explored.
Although our study reported some new findings about polymorphisms of TNF and TNFRSF1B genes in NSCLC patients, several potential limitations should be taken into consideration. Firstly, our sample size is relatively small with a limited statistical power to evaluate interactions between the studied polymorphisms and clinical factors including tumor stage and node status. For the same reason, the results from our subgroups by TNFRSF1B +676 T>G (rs1061622) genotypes and selected factors need validation by larger studies. Secondly, only five potentially functional SNPs in TNF and TNFRSF1B were included in our study, which is far from comprehensive. Indeed, both genes are highly polymorphic, and it is possible that some important SNPs may have been missed or the observed association may have been due to other polymorphisms in LD with the studied ones. Again, much larger studies would have the statistical power to confirm the associations of more SNPs with OS in such a patient population.
Conclusion
In summary, in this cohort of NSCLC patients, we identified a possible role of TNFRSF1B +676 T>G in the prognosis of NSCLC. However, replication studies with diverse ethnic groups, larger sample sizes and functional characterizations are warranted. Currently, we have ongoing projects that help continue recruiting similar patients for additional analysis in the future.
Abbreviations
- TNF-α :
-
Tumor necrosis factor-alpha
- TNFR2 :
-
tumor necrosis factor receptor super family 2
- PCR-RFLP :
-
polymerase chain reaction-restriction fragment length polymorphism
- HR :
-
hazard ratio
- CI :
-
confidence interval
- SNP :
-
single nucleotide polymorphism
- NSCLC :
-
non-small cell lung cancer
- OS :
-
overall survival.
References
Ferlay J, Shin HR, Bray F, Forman D, Mathers C, Parkin DM: Estimates of worldwide burden of cancer in 2008: GLOBOCAN 2008. Int J Cancer. 2010, 127: 2893-917. 10.1002/ijc.25516.
Gandara D, Narayan S, Lara PN, Goldberg Z, Davies A, Lau DH, Mack P, Gumerlock P, Vijayakumar S: Integration of novel therapeutics into combined modality therapy of locally advanced non-small cell lung cancer. Clin Cancer Res. 2005, 11: 5057s-5062s. 10.1158/1078-0432.CCR-05-9012.
Parkin DM, Bray F, Ferlay J, Pisani P: Global cancer statistics, 2002. CA Cancer J Clin. 2005, 55: 74-108. 10.3322/canjclin.55.2.74.
Naruke T, Tsuchiya R, Kondo H, Asamura H: Prognosis and survival after resection for bronchogenic carcinoma based on the 1997 TNM-staging classification: the Japanese experience. Ann Thorac Surg. 2001, 71: 1759-1764. 10.1016/S0003-4975(00)02609-6.
Aggarwal BB: Signalling pathways of the TNF superfamily: a double-edged sword. Nat Rev Immunol. 2003, 3: 745-756. 10.1038/nri1184.
Boldrini L, Calcinai A, Samaritani E, Pistolesi F, Mussi A, Lucchi M, Angeletti CA, Basolo F, Fontanini G: Tumour necrosis factor-alpha and transforming growth factor-beta are significantly associated with better prognosis in non-small cell lung carcinoma: putative relation with BCL-2-mediated neovascularization. Br J Cancer. 2000, 83: 480-486. 10.1054/bjoc.2000.1345.
Boldrini L, Gisfredi S, Ursino S, Lucchi M, Melfi F, Mussi A, Basolo F, Fontanini G: Tumour necrosis factor-alpha: prognostic role and relationship with interleukin-8 and endothelin-1 in non-small cell lung cancer. Int J Mol Med. 2006, 17: 887-892.
Miles DW, Happerfield LC, Naylor MS, Bobrow LG, Rubens RD, Balkwill FR: Expression of tumour necrosis factor (TNF alpha) and its receptors in benign and malignant breast tissue. Int J Cancer. 1994, 56: 777-782. 10.1002/ijc.2910560603.
Naylor MS, Stamp GW, Foulkes WD, Eccles D, Balkwill FR: Tumor necrosis factor and its receptors in human ovarian cancer. Potential role in disease progression. J Clin Invest. 1993, 91: 2194-2206. 10.1172/JCI116446.
Tran TA, Kallakury BV, Ambros RA, Ross JS: Prognostic significance of tumor necrosis factors and their receptors in nonsmall cell lung carcinoma. Cancer. 1998, 83: 276-282. 10.1002/(SICI)1097-0142(19980715)83:2<276::AID-CNCR11>3.0.CO;2-Q.
Matsuyama R, Togo S, Shimizu D, Momiyama N, Ishikawa T, Ichikawa Y, Endo I, Kunisaki C, Suzuki H, Hayasizaki Y, et al: Predicting 5-fluorouracil chemosensitivity of liver metastases from colorectal cancer using primary tumor specimens: three-gene expression model predicts clinical response. Int J Cancer. 2006, 119: 406-413. 10.1002/ijc.21843.
Su C, Zhou C, Zhou S, Xu J: Serum cytokine levels in patients with advanced non-small cell lung cancer: correlation with treatment response and survival. Med Oncol. 2010
Rube CE, Wilfert F, Uthe D, Schmid KW, Knoop R, Willich N, Schuck A, Rube C: Modulation of radiation-induced tumour necrosis factor alpha (TNF-alpha) expression in the lung tissue by pentoxifylline. Radiother Oncol. 2002, 64: 177-187. 10.1016/S0167-8140(02)00077-4.
Zhang M, Qian J, Xing X, Kong FM, Zhao L, Chen M, Lawrence TS: Inhibition of the tumor necrosis factor-alpha pathway is radioprotective for the lung. Clin Cancer Res. 2008, 14: 1868-1876. 10.1158/1078-0432.CCR-07-1894.
Hajeer AH, Hutchinson IV: Influence of TNFalpha gene polymorphisms on TNFalpha production and disease. Hum Immunol. 2001, 62: 1191-1199. 10.1016/S0198-8859(01)00322-6.
Negoro K, Kinouchi Y, Hiwatashi N, Takahashi S, Takagi S, Satoh J, Shimosegawa T, Toyota T: Crohn's disease is associated with novel polymorphisms in the 5'-flanking region of the tumor necrosis factor gene. Gastroenterology. 1999, 117: 1062-1068. 10.1016/S0016-5085(99)70390-2.
Westendorp RG, Langermans JA, Huizinga TW, Verweij CL, Sturk A: Genetic influence on cytokine production in meningococcal disease. Lancet. 1997, 349: 1912-1913. 10.1016/S0140-6736(05)63910-4.
Wilson AG, Symons JA, McDowell TL, McDevitt HO, Duff GW: Effects of a polymorphism in the human tumor necrosis factor alpha promoter on transcriptional activation. Proc Natl Acad Sci USA. 1997, 94: 3195-3199. 10.1073/pnas.94.7.3195.
Beltinger CP, White PS, Maris JM, Sulman EP, Jensen SJ, LePaslier D, Stallard BJ, Goeddel DV, de Sauvage FJ, Brodeur GM: Physical mapping and genomic structure of the human TNFR2 gene. Genomics. 1996, 35: 94-100. 10.1006/geno.1996.0327.
Morita C, Horiuchi T, Tsukamoto H, Hatta N, Kikuchi Y, Arinobu Y, Otsuka T, Sawabe T, Harashima S, Nagasawa K, et al: Association of tumor necrosis factor receptor type II polymorphism 196R with Systemic lupus erythematosus in the Japanese: molecular and functional analysis. Arthritis Rheum. 2001, 44: 2819-2827. 10.1002/1529-0131(200112)44:12<2819::AID-ART469>3.0.CO;2-2.
Bayley JP, Ottenhoff TH, Verweij CL: Is there a future for TNF promoter polymorphisms?. Genes Immun. 2004, 5: 315-329. 10.1038/sj.gene.6364055.
Gaudet MM, Egan KM, Lissowska J, Newcomb PA, Brinton LA, Titus-Ernstoff L, Yeager M, Chanock S, Welch R, Peplonska B, et al: Genetic variation in tumor necrosis factor and lymphotoxin-alpha (TNF-LTA) and breast cancer risk. Hum Genet. 2007, 121: 483-490. 10.1007/s00439-006-0315-x.
Hajeer AH, Hutchinson IV: TNF-alpha gene polymorphism: clinical and biological implications. Microsc Res Tech. 2000, 50: 216-228. 10.1002/1097-0029(20000801)50:3<216::AID-JEMT5>3.0.CO;2-Q.
Leek RD, Landers R, Fox SB, Ng F, Harris AL, Lewis CE: Association of tumour necrosis factor alpha and its receptors with thymidine phosphorylase expression in invasive breast carcinoma. Br J Cancer. 1998, 77: 2246-2251. 10.1038/bjc.1998.373.
Shih CM, Lee YL, Chiou HL, Chen W, Chang GC, Chou MC, Lin LY: Association of TNF-alpha polymorphism with susceptibility to and severity of non-small cell lung cancer. Lung Cancer. 2006, 52: 15-20. 10.1016/j.lungcan.2005.11.011.
Mestiri S, Bouaouina N, Ben Ahmed S, Chouchane L: A functional polymorphism of the tumor necrosis factor receptor-II gene associated with the survival and relapse prediction of breast carcinoma. Cytokine. 2005, 30: 182-187. 10.1016/j.cyto.2005.01.007.
Stankovic MM, Nestorovic AR, Tomovic AM, Petrovic-Stanojevic ND, Andjelic-Jelic MS, Dopudja-Pantic VB, Nagorni-Obradovic Lj M, Mitic-Milikic MM, Radojkovic DP: TNF-alpha-308 promotor polymorphism in patients with chronic obstructive pulmonary disease and lung cancer. Neoplasma. 2009, 56: 348-352. 10.4149/neo_2009_04_348.
Ahirwar DK, Mandhani A, Dharaskar A, Kesarwani P, Mittal RD: Association of tumour necrosis factor-alpha gene (T-1031C, C-863A, and C-857T) polymorphisms with bladder cancer susceptibility and outcome after bacille Calmette-Guerin immunotherapy. BJU Int. 2009, 104: 867-873. 10.1111/j.1464-410X.2009.08549.x.
Kim EJ, Jeong P, Quan C, Kim J, Bae SC, Yoon SJ, Kang JW, Lee SC, Jun Wee J, Kim WJ: Genotypes of TNF-alpha, VEGF, hOGG1, GSTM1, and GSTT1: useful determinants for clinical outcome of bladder cancer. Urology. 2005, 65: 70-75. 10.1016/j.urology.2004.08.005.
Jang WH, Yang YI, Yea SS, Lee YJ, Chun JH, Kim HI, Kim MS, Paik KH: The -238 tumor necrosis factor-alpha promoter polymorphism is associated with decreased susceptibility to cancers. Cancer Lett. 2001, 166: 41-46. 10.1016/S0304-3835(01)00438-4.
Yapijakis C, Serefoglou Z, Vylliotis A, Nkenke E, Derka S, Vassiliou S, Avgoustidis D, Neukam FW, Patsouris E, Vairaktaris E: Association of polymorphisms in Tumor Necrosis Factor Alpha and Beta genes with increased risk for oral cancer. Anticancer Res. 2009, 29: 2379-2386.
Gupta R, Sharma SC, Das SN: Association of TNF-alpha and TNFR1 promoters and 3' UTR region of TNFR2 gene polymorphisms with genetic susceptibility to tobacco-related oral carcinoma in Asian Indians. Oral Oncol. 2008, 44: 455-463. 10.1016/j.oraloncology.2007.06.003.
Machado JC, Figueiredo C, Canedo P, Pharoah P, Carvalho R, Nabais S, Castro Alves C, Campos ML, Van Doorn LJ, Caldas C, et al: A proinflammatory genetic profile increases the risk for chronic atrophic gastritis and gastric carcinoma. Gastroenterology. 2003, 125: 364-371. 10.1016/S0016-5085(03)00899-0.
Fang F, Yao L, Yu XJ, Yu L, Wu Q: TNFalpha -308 G/A polymorphism is associated with breast cancer risk: a meta-analysis involving 10,184 cases and 12,911 controls. Breast Cancer Res Treat. 122: 267-271.
Jeong P, Kim EJ, Kim EG, Byun SS, Kim CS, Kim WJ: Association of bladder tumors and GA genotype of -308 nucleotide in tumor necrosis factor-alpha promoter with greater tumor necrosis factor-alpha expression. Urology. 2004, 64: 1052-1056. 10.1016/j.urology.2004.06.018.
Mestiri S, Bouaouina N, Ahmed SB, Khedhaier A, Jrad BB, Remadi S, Chouchane L: Genetic variation in the tumor necrosis factor-alpha promoter region and in the stress protein hsp70-2: susceptibility and prognostic implications in breast carcinoma. Cancer. 2001, 91: 672-678. 10.1002/1097-0142(20010215)91:4<672::AID-CNCR1050>3.0.CO;2-J.
Kladny J, Suchy J, Klujszo-Grabowska E, Kacperski T, Scott RJ, Kurzawski G, Lubinski J: Clinical characteristics of tumors derived from colorectal cancer patients who harbor the tumor necrosis factor alpha-1031T/T and NOD2 3020insC polymorphism. Cancer Epidemiol. 2009, 33: 161-163. 10.1016/j.canep.2009.06.004.
Yuan X, Liao Z, Liu Z, Wang LE, Tucker SL, Mao L, Wang XS, Martel M, Komaki R, Cox JD, et al: Single nucleotide polymorphism at rs1982073:T869C of the TGFbeta 1 gene is associated with the risk of radiation pneumonitis in patients with non-small-cell lung cancer treated with definitive radiotherapy. J Clin Oncol. 2009, 27: 3370-3378. 10.1200/JCO.2008.20.6763.
Xu Z, Taylor JA: SNPinfo: integrating GWAS and candidate gene information into functional SNP selection for genetic association studies. Nucleic Acids Res. 2009, 37: W600-605. 10.1093/nar/gkp290.
Greene FL: The American Joint Committee on Cancer: updating the strategies in cancer staging. Bull Am Coll Surg. 2002, 87: 13-15.
Das SN, Baniasadi V, Kapuria V: Association of -308 TNF-alpha promoter polymorphism with type 1 diabetes in North Indians. Int J Immunogenet. 2006, 33: 411-416. 10.1111/j.1744-313X.2006.00632.x.
Glas J, Torok HP, Schneider A, Brunnler G, Kopp R, Albert ED, Stolte M, Folwaczny C: Allele 2 of the interleukin-1 receptor antagonist gene is associated with early gastric cancer. J Clin Oncol. 2004, 22: 4746-4752. 10.1200/JCO.2004.03.034.
Sharma R, Zucknick M, London R, Kacevska M, Liddle C, Clarke SJ: Systemic inflammatory response predicts prognosis in patients with advanced-stage colorectal cancer. Clin Colorectal Cancer. 2008, 7: 331-337. 10.3816/CCC.2008.n.044.
Hohaus S, Giachelia M, Di Febo A, Martini M, Massini G, Vannata B, D'Alo F, Guidi F, Greco M, Pierconti F, et al: Polymorphism in cytokine genes as prognostic markers in Hodgkin's lymphoma. Ann Oncol. 2007, 18: 1376-1381. 10.1093/annonc/mdm132.
Smith KC, Bateman AC, Fussell HM, Howell WM: Cytokine gene polymorphisms and breast cancer susceptibility and prognosis. Eur J Immunogenet. 2004, 31: 167-173. 10.1111/j.1365-2370.2004.00462.x.
Tartaglia LA, Pennica D, Goeddel DV: Ligand passing: the 75-kDa tumor necrosis factor (TNF) receptor recruits TNF for signaling by the 55-kDa TNF receptor. J Biol Chem. 1993, 268: 18542-18548.
Stark GL, Dickinson AM, Jackson GH, Taylor PR, Proctor SJ, Middleton PG: Tumour necrosis factor receptor type II 196M/R genotype correlates with circulating soluble receptor levels in normal subjects and with graft-versus-host disease after sibling allogeneic bone marrow transplantation. Transplantation. 2003, 76: 1742-1749. 10.1097/01.TP.0000092496.05951.D5.
Till A, Rosenstiel P, Krippner-Heidenreich A, Mascheretti-Croucher S, Croucher PJ, Schafer H, Scheurich P, Seegert D, Schreiber S: The Met-196 -> Arg variation of human tumor necrosis factor receptor 2 (TNFR2) affects TNF-alpha-induced apoptosis by impaired NF-kappaB signaling and target gene expression. J Biol Chem. 2005, 280: 5994-6004.
Kuwahara A, Yamamori M, Fujita M, Okuno T, Tamura T, Kadoyama K, Okamura N, Nakamura T, Sakaeda T: TNFRSF1B A1466G genotype is predictive of clinical efficacy after treatment with a definitive 5-fluorouracil/cisplatin-based chemoradiotherapy in Japanese patients with esophageal squamous cell carcinoma. J Exp Clin Cancer Res. 2010, 29: 100-10.1186/1756-9966-29-100.
Sainz J, Pérez E, Hassan L, Moratalla A, Romero A, Collado MD, Jurado M: Variable number of tandem repeats of TNF receptor type 2 promoter as genetic biomarker of susceptibility to develop invasive pulmonary aspergillosis. Hum Immunol. 2007, 68: 41-50. 10.1016/j.humimm.2006.10.011.
Pre-publication history
The pre-publication history for this paper can be accessed here:http://www.biomedcentral.com/1471-2407/11/447/prepub
Acknowledgements and Funding
This study was in part supported by National Institutes of Health grants R01 ES11740 and CA131274 (to Q. W.) and CA16672 (to M. D. Anderson Cancer Center). We thank Margaret Lung for assistance in data management of the subjects and Hui Zhao for the data analysis assistance.
Author information
Authors and Affiliations
Corresponding authors
Additional information
Competing interests
The authors declare that they have no competing interests.
Authors' contributions
XXG, QYW and ZXL designed the study and prepared the manuscript. XXG performed the experiments with the assistance from ZSL and JQ. XXG and HXM conducted the analysis with assistance from LEW. XLY, DG and RK helped to collect and organize the clinical data. HXM, QYW and LEW helped to finalize the manuscript. All authors read and approved the final manuscript.
Authors’ original submitted files for images
Below are the links to the authors’ original submitted files for images.
Rights and permissions
Open Access This article is published under license to BioMed Central Ltd. This is an Open Access article is distributed under the terms of the Creative Commons Attribution License ( https://creativecommons.org/licenses/by/2.0 ), which permits unrestricted use, distribution, and reproduction in any medium, provided the original work is properly cited.
About this article
Cite this article
Guan, X., Liao, Z., Ma, H. et al. TNFRSF1B +676 T>G polymorphism predicts survival of non-Small cell lung cancer patients treated with chemoradiotherapy. BMC Cancer 11, 447 (2011). https://doi.org/10.1186/1471-2407-11-447
Received:
Accepted:
Published:
DOI: https://doi.org/10.1186/1471-2407-11-447