Abstract
There has been little information available on how the use of dextran in vivo influences plasma viscosity and yield stress of blood. Hence, the main purpose of this study is to provide such information when level of red blood cell aggregation in rats was raised to levels seen in normal human blood with administration of Dextran 500. Our results show that plasma viscosity (P < 0.003) and yield stress (P < 0.01) of rat blood increased significantly after dextran infusion. Whole blood viscosity also significantly increased with the dextran treatment over the entire range of shear rates we measured. The viscosity changes at high shear rates after dextran infusion would be due mainly to elevation of plasma viscosity, whereas the changes at low shear rates would be attributed to red blood cell aggregation induced by the dextran treatment.
Similar content being viewed by others
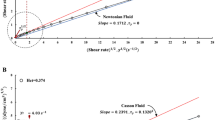
References
A. S. Popel, P. C. Johnson, M. V. Kameneva and M. A. Wild, Capacity for red blood cell aggregation is higher in athletic mammalian species than in sedentary species, J. Appl. Physiol., 77(4) (1994) 1790–1794.
S. Chien and K. Jan, Red cell aggregation by macromolecules: roles of surface adsorption and electrostatic repulsion, J. Supramol. Struct., 1 (1973) 385–409.
S. Chien and K. Jan, Ultrastructural basis of the mechanism of rouleaux formation, Microvascular Research, 5 (1973) 155–166.
W. Reinke, P. Gaehtgens and P. C. Johnson, Blood viscosity in small tubes: effect of shear rate, aggregation and sedimentation, Am. J. Physiol. Heart. Circ. Physiol., 253 (1987) 540–547.
J. J. Bishop, A. S. Popel, M. Intaglietta and P. C. Johnson, Effects of erythrocyte aggregation and venous network geometry on red blood cell axial migration, Am. J. Physiol. Heart. Circ. Physiol., 281 (2001) 939–950.
P. C. Johnson, J. J. Bishop, A. S. Popel and M. Intaglietta, Effects of red cell aggregation on the venous microcirculation, Biorheology, 36 (1999) 457–460.
M. Cabel, H. J. Meiselman, A. S. Popel and P. C. Johnson, Contribution of red blood cell aggregation to venous vascular resistance in skeletal muscle, Am. J. Physiol. Heart. Circ. Physiol., 272 (1997) 1020–1032.
G. Mchedlishvili, L. Gobejishvili and N. Beritashvili, Effect of intensified red blood cell aggregability on arterial pressure and mesenteric microcirculation, Microvascular Research, 45 (1993) 233–242.
R. Y. Z. Chen, R. D. Carlin, S. Simchon, K. Jan, and S. Chien, Effects of dextran-induced hyperviscosity on regional blood flow and hemodynamics in dogs, Am. J. Physiol. Heart. Circ. Physiol., 256 (1989) 898–905.
L. Gustafsson, L. Appelgren and H. E. Myrvold, Effects of increased plasma viscosity and red blood cell aggregation on blood viscosity in vivo, Am. J. Physiol. Heart. Circ. Physiol., 241 (1981) 513–518.
E. W. Merrill, G. C. Cokelet, A. Britten and R. E. Wells, Non-Newtonian rheology of human blood-effect of fibrinogen deduced by “subtraction”, Circ. Res., 13 (1963) 48–55.
E. W. Merrill, E. R. Gilliland, T. S. Lee and E. W. Salzman, Blood rheology: effect of fibrinogen deduced by addition, Circ. Res., 18 (1966) 437–446.
S. Kim, Y. I. Cho, W. N. Hogenauer and K. R. Kensey, A method of isolating surface tension and yield stress effects in a U-shaped scanning capillary-tube viscometer using a casson model, J. Non-Newtonian Fluid Mech., 103 (2002) 205–219.
S. Kim, Y. I. Cho, A. H. Jeon, B. Hogenauer and K. R. Kensey, A new method for blood viscosity measurement, J. Non-Newtonian Fluid Mech., 94 (2000) 47–56.
S. E. Charm and G. S. Kurland, Static method for determining blood yield stress, Nature, 216 (1967) 1121–1123.
C. Picart, J. Piau and H. Galliard, Human blood shear yield stress and its hematocrit dependence, J. Rheol., 42(1) (1998) 1–12.
B. K. Lee, T. Alexy, W. R. B. and H. J. Meiselman, Red blood cell aggregation quantified with Myrenne aggregometer and yield shear stress, Biorheology, 44 (2007) 29–35.
E. Vicaut, Opposite effects of red blood cell aggregation on resistance to blood flow, J. Cardiovasc. Surg., 36 (1995) 361–368.
S. D. House and P. C. Johnson, Diameter and blood flow of skeletal muscle venules during local flow regulation, Am. J. Physiol. Heart. Circ. Physiol., 250 (1986) 828–837.
H. Schmid-Schonbein, P. Gaehtgens and H. Hirsch, On the shear rate dependence of red cell aggregation in vitro, Journal of Clinical Investigation, 47 (1968) 1447–1454.
M. Soutani, Y. Suzuki, N Tateishi and N. Maeda, Quantitative evaluation of flow dynamics of erythrocytes in microvessels: influence of erythrocyte aggregation, Am. J. Physiol. Heart. Circ. Physiol., 268 (1995) 1959–1965.
J. J. Bishop, P. R. Nance, A. S. Popel, M. Intaglietta and P.C. Johnson, Effect of erythrocyte aggregation on velocity profiles in venules, Am. J. Physiol. Heart. Circ. Physiol., 280 (2001) 222–236.
R. Busse and I. Fleming, Regulation of endothelium-derived vasoactive autacoid production by hemodynamic forces, Trends Pharmacol. Sci., 24 (2003) 24–29.
A. Calver, J. Collier and P. Vallance, Nitric oxide and cardiovascular control, Exp. Physiol., 78 (1993) 303–326.
S. Moncada, Nitric oxide: discovery and impact on clinical medicine, J. R. Soc. Med. 92 (1999) 164–169.
G. Radegran and B. Saltin, Nitric oxide in the regulation of vasomotor tone in human skeletal muscle, Am. J. Physiol. Heart. Circ. Physiol., 276 (1999) 1951–1960.
Author information
Authors and Affiliations
Corresponding author
Additional information
This paper was recommended for publication in revised form by Associate Editor Haecheon Choi
Sangho Kim received his B.S. degree in Mechanical Engineering from Kyung-Pook National University in 1997. He then received his Ph.D. degree from Drexel University, USA, in 2002. Dr. Kim is currently an Assistant Professor in the Division of Bioengineering and the Department of Surgery at the National University of Singapore in Singapore.
Shihong Yang received his B.S. degree in Bioengineering from the National University of Singapore, Singapore, in 2007. He is currently a research engineer in the Division of Bioengineering, National University of Singapore, Singapore.
Dohyung Lim received B.S. and M.S. degrees in Biomedical Engineering from Inje University, Kimhae, Korea, in 1998 and 2000, respectively. He then went on to receive his Ph.D. degree from the School of Biomedical Engineering, Science, & Health Systems, Drexel University, Philadelphia, PA, USA, in 2004. Dr. Lim completed a postdoctoral fellowship in the Department of Physical Therapy and Human Movement Science, Feinberg School of Medicine, Northwestern University, Chicago, IL, USA and as a Research Professor of Biomedical Engineering, Yonsei University, Wonju, Gangwon, Korea. Dr. Lim is currently a Senior Researcher at the Korea Institute of Industrial Technology in Cheonan, Chungnam, Korea.
Rights and permissions
About this article
Cite this article
Kim, S., Yang, S. & Lim, D. Effect of dextran on rheological properties of rat blood. J Mech Sci Technol 23, 868–873 (2009). https://doi.org/10.1007/s12206-009-0211-0
Received:
Revised:
Accepted:
Published:
Issue Date:
DOI: https://doi.org/10.1007/s12206-009-0211-0