Abstract
By proteolytic cleavage, matrix metalloproteinases (MMPs) not only remodel the extracellular matrix (ECM) but they also modify the structure and activity of other proteinases, growth factors, signaling molecules, cell surface receptors, etc. Their vast substrate repertoire adds a complex extra dimension of biological control and turns MMPs into important regulatory nodes in the protease web. In the central nervous system (CNS), the detrimental impact of elevated MMP activities has been well-described for traumatic injuries and many neurodegenerative diseases. Nonetheless, there is ample proof corroborating MMPs as fine regulators of CNS physiology, and well-balanced MMP activity is instrumental to development, plasticity, and repair. In this manuscript, we review the emerging evidence for MMPs as beneficial modulators of axonal regeneration in the mammalian CNS. By exploring the multifactorial causes underlying the inability of mature axons to regenerate, and describing how MMPs can help to overcome these hurdles, we emphasize the benign actions of these Janus-faced proteases.

Similar content being viewed by others
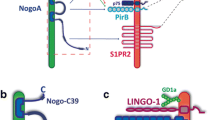
References
Ethell IM, Ethell DW (2007) Matrix metalloproteinases in brain development and remodeling: synaptic functions and targets. J Neurosci Res 85(13):2813–2823
Fujioka H, Dairyo Y, Yasunaga K, Emoto K (2012) Neural functions of matrix metalloproteinases: plasticity, neurogenesis, and disease. Biochem Res Int 2012:789083. doi:10.1155/2012/789083
Morrison CJ, Butler GS, RodrÃguez D, Overall CM (2009) Matrix metalloproteinase proteomics: substrates, targets, and therapy. Curr Opin Cell Biol 21(5):645–653. doi:10.1016/j.ceb.2009.06.006
Rodríguez D, Morrison CJ, Overall CM (2010) Matrix metalloproteinases: what do they not do? New substrates and biological roles identified by murine models and proteomics. Biochim Biophys Acta (BBA) - Mol Cell Res 1803(1):39–54. doi:10.1016/j.bbamcr.2009.09.015
Schlage P, Keller UA (2015) Proteomic approaches to uncover MMP function. Matrix Biol. doi:10.1016/j.matbio.2015.01.003
McCawley LJ, Matrisian LM (2001) Matrix metalloproteinases: they’re not just for matrix anymore! Curr Opin Cell Biol 13(5):534–540. doi:10.1016/S0955-0674(00)00248-9
Cauwe B, Opdenakker G (2010) Intracellular substrate cleavage: a novel dimension in the biochemistry, biology and pathology of matrix metalloproteinases. Crit Rev Biochem Mol Biol 45(5):351–423. doi:10.3109/10409238.2010.501783
Mannello F, Medda V (2012) Nuclear localization of matrix metalloproteinases. Prog Histochem Cytochem 47(1):27–58. doi:10.1016/j.proghi.2011.12.002
Bai G, Pfaff SL (2011) Protease regulation: the Yin and Yang of neural development and disease. Neuron 72(1):9–21. doi:10.1016/j.neuron.2011.09.012
Agrawal SM, Lau L, Yong VW (2008) MMPs in the central nervous system: where the good guys go bad. Semin Cell Dev Biol 19(1):42–51. doi:10.1016/j.semcdb.2007.06.003
Page-McCaw A, Ewald AJ, Werb Z (2007) Matrix metalloproteinases and the regulation of tissue remodelling. Nat Rev Mol Cell Biol 8(3):221–233. doi:10.1038/nrm2125
Fingleton B (2008) MMPs as therapeutic targets—still a viable option? Semin Cell Dev Biol 19(1):61–68. doi:10.1016/j.semcdb.2007.06.006
Vandenbroucke RE, Libert C (2014) Is there new hope for therapeutic matrix metalloproteinase inhibition? Nat Rev Drug Discov 13(12):904–927. doi:10.1038/nrd4390
Fields GB (2015) New strategies for targeting matrix metalloproteinases. Matrix Biol. doi:10.1016/j.matbio.2015.01.002
Miller JP, Holcomb J, Al-Ramahi I, de Haro M, Gafni J, Zhang N, Kim E, Sanhueza M et al (2010) Matrix metalloproteinases are modifiers of huntingtin proteolysis and toxicity in Huntington’s disease. Neuron 67(2):199–212. doi:10.1016/j.neuron.2010.06.021
Rivera S, Khrestchatisky M, Kaczmarek L, Rosenberg GA, Jaworski DM (2010) Metzincin proteases and their inhibitors: foes or friends in nervous system physiology? J Neurosci 30(46):15337–15357. doi:10.1523/JNEUROSCI.3467-10.2010
Rosenberg GA (2009) Matrix metalloproteinases and their multiple roles in neurodegenerative diseases. Lancet Neurol 8(2):205–216. doi:10.1016/S1474-4422(09)70016-X
Yong VW (2005) Metalloproteinases: mediators of pathology and regeneration in the CNS. Nat Rev Neurosci 6(12):931–944. doi:10.1038/nrn1807
Verslegers M, Lemmens K, Van Hove I, Moons L (2013) Matrix metalloproteinase-2 and −9 as promising benefactors in development, plasticity and repair of the nervous system. Prog Neurobiol 105:60–78. doi:10.1016/j.pneurobio.2013.03.004
Zhang H, Adwanikar H, Werb Z, Noble-Haeusslein LJ (2010) Matrix metalloproteinases and neurotrauma: evolving roles in injury and reparative processes. Neuroscientist 16(2):156–170. doi:10.1177/1073858409355830
Tonti GA, Mannello F, Cacci E, Biagioni S (2009) Neural stem cells at the crossroads: MMPs may tell the way. Int J Dev Biol 53(1):1–17. doi:10.1387/ijdb.082573gt
Milward EA, Fitzsimmons C, Szklarczyk A, Conant K (2007) The matrix metalloproteinases and CNS plasticity: an overview. J Neuroimmunol 187(1–2):9–19. doi:10.1016/j.jneuroim.2007.04.010
Van Hove I, Lemmens K, Van de Velde S, Verslegers M, Moons L (2012) Matrix metalloproteinase-3 in the central nervous system: a look on the bright side. J Neurochem 2012(2):1471–4159
Wiera G, Szczot M, Wojtowicz T, Lebida K, Koza P, Mozrzymas J (2015) Impact of matrix metalloproteinase-9 overexpression on synaptic excitatory transmission and its plasticity in rat CA3-CA1 hippocampal pathway. J Physiol Pharmacol. doi:info:pmid/25903961
Becker CG, Becker T (2007) Growth and pathfinding of regenerating axons in the optic projection of adult fish. J Neurosci Res 85(12):2793–2799. doi:10.1002/jnr.21121
Becker T, Becker CG (2014) Axonal regeneration in zebrafish. Curr Opin Neurobiol 27:186–191. doi:10.1016/j.conb.2014.03.019
Fleisch VC, Fraser B, Allison WT (2011) Investigating regeneration and functional integration of CNS neurons: lessons from zebrafish genetics and other fish species. Biochim Biophys Acta 3:364–380
Lemmens K, Bollaerts I, Bhumika S, De Groef L, Van Houcke J, Darras VM, Van Hove I, Moons L (2015) Matrix metalloproteinases as promising regulators of axonal regrowth in the injured adult zebrafish retinotectal system. J Comp Neurol 28(10):23920
McCurley AT, Callard GV (2010) Time course analysis of gene expression patterns in zebrafish eye during optic nerve regeneration. J Exp Neurosci 2010(4):17–33
Chernoff EA (1996) Spinal cord regeneration: a phenomenon unique to urodeles? Int J Dev Biol 40(4):823–831
Chernoff EA, O’Hara CM, Bauerle D, Bowling M (2000) Matrix metalloproteinase production in regenerating axolotl spinal cord. Wound Repair Regen 8(4):282–291
Yang P, Yang Z (2012) Enhancing intrinsic growth capacity promotes adult CNS regeneration. J Neurol Sci 312(1–2):1–6. doi:10.1016/j.jns.2011.08.037
Goldberg JL, Barres BA (2000) The relationship between neuronal survival and regeneration. Annu Rev Neurosci 23(1):579–612. doi:10.1146/annurev.neuro.23.1.579
Ahmed Z, Dent RG, Leadbeater WE, Smith C, Berry M, Logan A (2005) Matrix metalloproteases: degradation of the inhibitory environment of the transected optic nerve and the scar by regenerating axons. Mol Cell Neurosci 28(1):64–78. doi:10.1016/j.mcn.2004.08.013
Pizzi MA, Crowe MJ (2007) Matrix metalloproteinases and proteoglycans in axonal regeneration. Exp Neurol 204(2):496–511
David S, Kroner A (2011) Repertoire of microglial and macrophage responses after spinal cord injury. Nat Rev Neurosci 12(7):388–399
Neumann H, Kotter MR, Franklin RJM (2009) Debris clearance by microglia: an essential link between degeneration and regeneration. Brain 132(2):288–295. doi:10.1093/brain/awn109
Reeves TM, Prins ML, Zhu J, Povlishock JT, Phillips LL (2003) Matrix metalloproteinase inhibition alters functional and structural correlates of deafferentation-induced sprouting in the dentate gyrus. J Neurosci 23(32):10182–10189
Duchossoy Y, Arnaud S, Feldblum S (2001) Matrix metalloproteinases: potential therapeutic target in spinal cord injury. Clin Chem Lab Med 39(4):362–367. doi:10.1515/CCLM.2001.057
Seo JH, Guo S, Lok J, Navaratna D, Whalen MJ, Kim KW, Lo EH (2012) Neurovascular matrix metalloproteinases and the blood–brain barrier. Curr Pharm Des 18(25):3645–3648
Rosenberg GA, Estrada EY, Dencoff JE (1998) Matrix metalloproteinases and TIMPs are associated with blood–brain barrier opening after reperfusion in rat brain. Stroke 29(10):2189–2195
Wells JEA, Rice TK, Nuttall RK, Edwards DR, Zekki H, Rivest S, Yong VW (2003) An adverse role for matrix metalloproteinase 12 after spinal cord injury in mice. J Neurosci 23(31):10107–10115
Hautamaki RD, Kobayashi DK, Senior RM, Shapiro SD (1997) Requirement for macrophage elastase for cigarette smoke-induced emphysema in mice. Science 277(5334):2002–2004
Lanone S, Zheng T, Zhu Z, Liu W, Lee CG, Ma B, Chen Q, Homer RJ et al (2002) Overlapping and enzyme-specific contributions of matrix metalloproteinases-9 and −12 in IL-13-induced inflammation and remodeling. J Clin Invest 110(4):463–474
Shipley JM, Wesselschmidt RL, Kobayashi DK, Ley TJ, Shapiro SD (1996) Metalloelastase is required for macrophage-mediated proteolysis and matrix invasion in mice. Proc Natl Acad Sci U S A 93(9):3942–3946
Hsu JY, McKeon R, Goussev S, Werb Z, Lee JU, Trivedi A, Noble-Haeusslein LJ (2006) Matrix metalloproteinase-2 facilitates wound healing events that promote functional recovery after spinal cord injury. J Neurosci 26(39):9841–9850. doi:10.1523/JNEUROSCI.1993-06.2006
de Castro RJ, Burns CL, McAdoo DJ, Romanic AM (2000) Metalloproteinase increases in the injured rat spinal cord. Neuroreport 11(16):3551–3554
Kim EM, Hwang O (2011) Role of matrix metalloproteinase-3 in neurodegeneration. J Neurochem 116(1):22–32. doi:10.1111/j.1471-4159.2010.07082.x
Busch SA, Horn KP, Silver DJ, Silver J (2009) Overcoming macrophage-mediated axonal dieback following CNS injury. J Neurosci 29(32):9967–9976. doi:10.1523/jneurosci.1151-09.2009
Kawano H, Kimura-Kuroda J, Komuta Y, Yoshioka N, Li H, Kawamura K, Li Y, Raisman G (2012) Role of the lesion scar in the response to damage and repair of the central nervous system. Cell Tissue Res 349(1):169–180. doi:10.1007/s00441-012-1336-5
Hsu JY, Bourguignon LY, Adams CM, Peyrollier K, Zhang H, Fandel T, Cun CL, Werb Z et al (2008) Matrix metalloproteinase-9 facilitates glial scar formation in the injured spinal cord. J Neurosci 28(50):13467–13477. doi:10.1523/JNEUROSCI.2287-08.2008
Silver J, Miller JH (2004) Regeneration beyond the glial scar. Nat Rev Neurosci 5(2):146–156
Duchossoy Y, Horvat JC, Stettler O (2001) MMP-related gelatinase activity is strongly induced in scar tissue of injured adult spinal cord and forms pathways for ingrowing neurites. Mol Cell Neurosci 17(6):945–956
Goussev S, Hsu JY, Lin Y, Tjoa T, Maida N, Werb Z, Noble-Haeusslein LJ (2003) Differential temporal expression of matrix metalloproteinases after spinal cord injury: relationship to revascularization and wound healing. J Neurosurg 99(2 Suppl):188–197
Davies SJ, Goucher DR, Doller C, Silver J (1999) Robust regeneration of adult sensory axons in degenerating white matter of the adult rat spinal cord. J Neurosci 19(14):5810–5822
Pastrana E, Moreno-Flores MT, Gurzov EN, Avila J, Wandosell F, Diaz-Nido J (2006) Genes associated with adult axon regeneration promoted by olfactory ensheathing cells: a new role for matrix metalloproteinase 2. J Neurosci 26(20):5347–5359. doi:10.1523/JNEUROSCI.1111-06.2006
Wang X, Jung J, Asahi M, Chwang W, Russo L, Moskowitz MA, Dixon CE, Fini ME et al (2000) Effects of matrix metalloproteinase-9 gene knock-out on morphological and motor outcomes after traumatic brain injury. J Neurosci 20(18):7037–7042
Noble LJ, Donovan F, Igarashi T, Goussev S, Werb Z (2002) Matrix metalloproteinases limit functional recovery after spinal cord injury by modulation of early vascular events. J Neurosci 22(17):7526–7535
Jones LL, Yamaguchi Y, Stallcup WB, Tuszynski MH (2002) NG2 is a major chondroitin sulfate proteoglycan produced after spinal cord injury and is expressed by macrophages and oligodendrocyte progenitors. J Neurosci 22(7):2792–2803
Trivedi A, Hsu JY, Lin Y, Goussev S, Gan J, Topp KS, Noble-Haeusslein LJ (2005) The effects of acute and extended inhibition of matrix metalloproteinases on demyelination and functional recovery after spinal cord injury. Int J Neuroprotect Neuroregen 2:30–38
Yao JS, Chen Y, Zhai W, Xu K, Young WL, Yang GY (2004) Minocycline exerts multiple inhibitory effects on vascular endothelial growth factor-induced smooth muscle cell migration: the role of ERK1/2, PI3K, and matrix metalloproteinases. Circ Res 95(4):364–371. doi:10.1161/01.RES.0000138581.04174.2f
Xu J, Kim GM, Ahmed SH, Yan P, Xu XM, Hsu CY (2001) Glucocorticoid receptor-mediated suppression of activator protein-1 activation and matrix metalloproteinase expression after spinal cord injury. J Neurosci 21(1):92–97
Muir EM, Adcock KH, Morgenstern DA, Clayton R, von Stillfried N, Rhodes K, Ellis C, Fawcett JW et al (2002) Matrix metalloproteases and their inhibitors are produced by overlapping populations of activated astrocytes. Mol Brain Res 100(1–2):103–117
Cua RC, Lau LW, Keough MB, Midha R, Apte SS, Yong VW (2013) Overcoming neurite-inhibitory chondroitin sulfate proteoglycans in the astrocyte matrix. Glia:NA. doi:10.1002/glia.22489
Ferguson TA, Muir D (2000) MMP-2 and MMP-9 increase the neurite-promoting potential of schwann cell basal laminae and are upregulated in degenerated nerve. Mol Cell Neurosci 16(2):157–167. doi:10.1006/mcne.2000.0859
Zuo J, Ferguson TA, Hernandez YJ, Stetler-Stevenson WG, Muir D (1998) Neuronal matrix metalloproteinase-2 degrades and inactivates a neurite-inhibiting chondroitin sulfate proteoglycan. J Neurosci 18(14):5203–5211
Nordstrom LA, Lochner J, Yeung W, Ciment G (1995) The metalloproteinase stromelysin-1 (transin) mediates PC12 cell growth cone invasiveness through basal laminae. Mol Cell Neurosci 6(1):56–68. doi:10.1006/mcne.1995.1006
Filous AR, Miller JH, Coulson-Thomas YM, Horn KP, Alilain WJ, Silver J (2010) Immature astrocytes promote CNS axonal regeneration when combined with chondroitinase ABC. Dev Neurobiol 70(12):826–841
Shechter R, Raposo C, London A, Sagi I, Schwartz M (2011) The glial scar-monocyte interplay: a pivotal resolution phase in spinal cord repair. PLoS One 6(12), e27969. doi:10.1371/journal.pone.0027969
Ahmed Z, Bansal D, Tizzard K, Surey S, Esmaeili M, Gonzalez AM, Berry M, Logan A (2014) Decorin blocks scarring and cystic cavitation in acute and induces scar dissolution in chronic spinal cord wounds. Neurobiol Dis 64:163–176. doi:10.1016/j.nbd.2013.12.008
Durigova M, Nagase H, Mort JS, Roughley PJ (2011) MMPs are less efficient than ADAMTS5 in cleaving aggrecan core protein. Matrix Biol 30(2):145–153
Zhang Y, Klassen HJ, Tucker BA, Perez MT, Young MJ (2007) CNS progenitor cells promote a permissive environment for neurite outgrowth via a matrix metalloproteinase-2-dependent mechanism. J Neurosci 27(17):4499–4506. doi:10.1523/JNEUROSCI.0200-07.2007
Verdu E, Garcia-Alias G, Fores J, Gudino-Cabrera G, Muneton VC, Nieto-Sampedro M, Navarro X (2001) Effects of ensheathing cells transplanted into photochemically damaged spinal cord. Neuroreport 12(11):2303–2309
Lakatos A, Barnett SC, Franklin RJ (2003) Olfactory ensheathing cells induce less host astrocyte response and chondroitin sulphate proteoglycan expression than Schwann cells following transplantation into adult CNS white matter. Exp Neurol 184(1):237–246
Li Y, Sauve Y, Li D, Lund RD, Raisman G (2003) Transplanted olfactory ensheathing cells promote regeneration of cut adult rat optic nerve axons. J Neurosci 23(21):7783–7788
Moreno-Flores MT, Bradbury EJ, Martin-Bermejo MJ, Agudo M, Lim F, Pastrana E, Avila J, Diaz-Nido J et al (2006) A clonal cell line from immortalized olfactory ensheathing glia promotes functional recovery in the injured spinal cord. Mol Ther 13(3):598–608, http://www.nature.com/mt/journal/v13/n3/suppinfo/mt200672s1.html
Veeravalli KK, Dasari VR, Tsung AJ, Dinh DH, Gujrati M, Fassett D, Rao JS (2009) Human umbilical cord blood stem cells upregulate matrix metalloproteinase-2 in rats after spinal cord injury. Neurobiol Dis 36(1):200–212. doi:10.1016/j.nbd.2009.07.012
Charalambous P, Hurst LA, Thanos S (2008) Engrafted chicken neural tube-derived stem cells support the innate propensity for axonal regeneration within the rat optic nerve. Invest Ophthalmol Vis Sci 49(8):3513–3524. doi:10.1167/iovs.07-1473
Berry M, Carlile J, Hunter A (1996) Peripheral nerve explants grafted into the vitreous body of the eye promote the regeneration of retinal ganglion cell axons severed in the optic nerve. J Neurocytol 25(2):147–170
Berry M, Carlile J, Hunter A, Tsang W, Rosenstiel P, Sievers J (1999) Optic nerve regeneration after intravitreal peripheral nerve implants: trajectories of axons regrowing through the optic chiasm into the optic tracts. J Neurocytol 28(9):721–741
Ogier C, Bernard A, Chollet AM, LE Diguardher T, Hanessian S, Charton G, Khrestchatisky M, Rivera S (2006) Matrix metalloproteinase-2 (MMP-2) regulates astrocyte motility in connection with the actin cytoskeleton and integrins. Glia 54(4):272–284. doi:10.1002/glia.20349
Pizzi MA, Crowe MJ (2006) Transplantation of fibroblasts that overexpress matrix metalloproteinase-3 into the site of spinal cord injury in rats. J Neurotrauma 23(12):1750–1765. doi:10.1089/neu.2006.23.1750
Ould-Yahoui A, Sbai O, Baranger K, Bernard A, Gueye Y, Charrat E, Clement B, Gigmes D et al (2013) Role of matrix metalloproteinases in migration and neurotrophic properties of nasal olfactory stem and ensheathing cells. Cell Transplant 22(6):993–1010. doi:10.3727/096368912X657468
Filbin MT (2003) Myelin-associated inhibitors of axonal regeneration in the adult mammalian CNS. Nat Rev Neurosci 4(9):703–713
Sandvig A, Berry M, Barrett LB, Butt A, Logan A (2004) Myelin-, reactive glia-, and scar-derived CNS axon growth inhibitors: expression, receptor signaling, and correlation with axon regeneration. Glia 46(3):225–251. doi:10.1002/glia.10315
Gijbels K, Proost P, Masure S, Carton H, Billiau A, Opdenakker G (1993) Gelatinase B is present in the cerebrospinal fluid during experimental autoimmune encephalomyelitis and cleaves myelin basic protein. J Neurosci Res 36(4):432–440. doi:10.1002/jnr.490360409
Proost P, Vandamme J, Opdenakker G (1993) Leukocyte gelatinase B cleavage releases encephalitogens from human myelin basic protein. Biochem Biophys Res Commun 192(3):1175–1181. doi:10.1006/bbrc.1993.1540
Beliën ATJ, Paganetti PA, Schwab ME (1999) Membrane-type 1 matrix metalloprotease (MT1-MMP) enables invasive migration of glioma cells in central nervous system white matter. J Cell Biol 144(2):373–384
Walmsley AR, McCombie G, Neumann U, Marcellin D, Hillenbrand R, Mir AK, Frentzel S (2004) Zinc metalloproteinase-mediated cleavage of the human Nogo-66 receptor. J Cell Sci 117(Pt 19):4591–4602. doi:10.1242/jcs.01324
Walmsley AR, Mir AK, Frentzel S (2005) Ectodomain shedding of human Nogo-66 receptor homologue-1 by zinc metalloproteinases. Biochem Biophys Res Commun 327(1):112–116
Ferraro GB, Morrison CJ, Overall CM, Strittmatter SM, Fournier AE (2011) Membrane-type matrix metalloproteinase-3 regulates neuronal responsiveness to myelin through Nogo-66 receptor 1 cleavage. J Biol Chem 286(36):31418–31424. doi:10.1074/jbc.M111.249169
Ahmed Z, Suggate EL, Brown ER, Dent RG, Armstrong SJ, Barrett LB, Berry M, Logan A (2006) Schwann cell-derived factor-induced modulation of the NgR/p75NTR/EGFR axis disinhibits axon growth through CNS myelin in vivo and in vitro. Brain 129(6):1517–1533
Jones M, Tussey L, Athanasou N, Jackson DG (2000) Heparan sulfate proteoglycan isoforms of the CD44 hyaluronan receptor induced in human inflammatory macrophages can function as paracrine regulators of fibroblast growth factor action. J Biol Chem 275(11):7964–7974
Whitelock JM, Murdoch AD, Iozzo RV, Underwood PA (1996) The degradation of human endothelial cell-derived perlecan and release of bound basic fibroblast growth factor by stromelysin, collagenase, plasmin, and heparanases. J Biol Chem 271(17):10079–10086
Lee R, Kermani P, Teng KK, Hempstead BL (2001) Regulation of cell survival by secreted proneurotrophins. Science 294(5548):1945–1948
Glaser J, Gonzalez R, Sadr E, Keirstead HS (2006) Neutralization of the chemokine CXCL10 reduces apoptosis and increases axon sprouting after spinal cord injury. J Neurosci Res 84(4):724–734
Van den Steen PE, Husson SJ, Proost P, Van Damme J, Opdenakker G (2003) Carboxyterminal cleavage of the chemokines MIG and IP-10 by gelatinase B and neutrophil collagenase. Biochem Biophys Res Commun 310(3):889–896
Yamagata T, Saito H, Habuchi O, Suzuki S (1968) Purification and properties of bacterial chondroitinases and chondrosulfatases. J Biol Chem 243(7):1523–1535
Siebert JR, Conta Steencken A, Osterhout DJ (2014) Chondroitin sulfate proteoglycans in the nervous system: inhibitors to repair. BioMed Res Int 2014:15. doi:10.1155/2014/845323
Winkler S, Stahl RC, Carey DJ, Bansal R (2002) Syndecan-3 and perlecan are differentially expressed by progenitors and mature oligodendrocytes and accumulate in the extracellular matrix. J Neurosci Res 69(4):477–487
Endo K, Takino T, Miyamori H, Kinsen H, Yoshizaki T, Furukawa M, Sato H (2003) Cleavage of syndecan-1 by membrane type matrix metalloproteinase-1 stimulates cell migration. J Biol Chem 278(42):40764–40770
Muir D, Engvall E, Varon S, Manthorpe M (1989) Schwannoma cell-derived inhibitor of the neurite-promoting activity of laminin. J Cell Biol 109(5):2353–2362
Fowlkes JL, Winkler MK (2002) Exploring the interface between metallo-proteinase activity and growth factor and cytokine bioavailability. Cytokine Growth Factor Rev 13(3):277–287. doi:10.1016/S1359-6101(02)00005-9
Hayashita-Kinoh H, Kinoh H, Okada A, Komori K, Itoh Y, Chiba T, Kajita M, Yana I et al (2001) Membrane-type 5 matrix metalloproteinase is expressed in differentiated neurons and regulates axonal growth. Cell Growth Differ : Mol Biol J Am Assoc Cancer Res 12(11):573–580
Chambaut-Guerin AM, Herigault S, Rouet-Benzineb P, Rouher C, Lafuma C (2000) Induction of matrix metalloproteinase MMP-9 (92-kDa gelatinase) by retinoic acid in human neuroblastoma SKNBE cells: relevance to neuronal differentiation. J Neurochem 74(2):508–517
Sheffield JB, Krasnopolsky V, Dehlinger E (1994) Inhibition of retinal growth cone activity by specific metalloproteinase inhibitors in vitro. Dev Dyn 200(1):79–88
Schlosshauer B, Walter J, Bonhoeffer F (1990) Is guidance of chick retinal axons in vitro influenced by proteases? Neurosci Lett 113(3):333–338
Hawkins RL, Seeds NW (1989) Protease inhibitors influence the direction of neurite outgrowth. Brain Res Dev Brain Res 45(2):203–209
Muir D (1994) Metalloproteinase-dependent neurite outgrowth within a synthetic extracellular matrix is induced by nerve growth factor. Exp Cell Res 210(2):243–252
Vaillant C, Meissirel C, Mutin M, Belin MF, Lund LR, Thomasset N (2003) MMP-9 deficiency affects axonal outgrowth, migration, and apoptosis in the developing cerebellum. Mol Cell Neurosci 24(2):395–408
Gaublomme D, Buyens T, De Groef L, Stakenborg M, Janssens E, Ingvarsen S, Porse A, Behrendt N et al (2014) Matrix metalloproteinase 2 and membrane type 1 matrix metalloproteinase co-regulate axonal outgrowth of mouse retinal ganglion cells. J Neurochem 129(6):966–979
Galko MJ, Tessier-Lavigne M (2000) Function of an axonal chemoattractant modulated by metalloprotease activity. Science 289(5483):1365–1367
Hattori M, Osterfield M, Flanagan JG (2000) Regulated cleavage of a contact-mediated axon repellent. Science 289(5483):1360–1365
Schimmelpfeng K, Gogel S, Klambt C (2001) The function of leak and kuzbanian during growth cone and cell migration. Mech Dev 106(1–2):25–36
Diaz-Rodriguez E, Cabrera N, Esparis-Ogando A, Montero JC, Pandiella A (1999) Cleavage of the TrkA neurotrophin receptor by multiple metalloproteases generates signalling-competent truncated forms. Eur J Neurosci 11(4):1421–1430
Naus S, Richter M, Wildeboer D, Moss M, Schachner M, Bartsch JW (2004) Ectodomain shedding of the neural recognition molecule CHL1 by the metalloprotease-disintegrin ADAM8 promotes neurite outgrowth and suppresses neuronal cell death. J Biol Chem 279(16):16083–16090
Hehr CL, Hocking JC, McFarlane S (2005) Matrix metalloproteinases are required for retinal ganglion cell axon guidance at select decision points. Development 132(15):3371–3379. doi:10.1242/dev.01908
Janssens E, Gaublomme D, De Groef L, Darras VM, Arckens L, Delorme N, Claes F, Van Hove I et al (2013) Matrix metalloproteinase 14 in the zebrafish: an eye on retinal and retinotectal development. PLoS One 8(1), e52915. doi:10.1371/journal.pone.0052915
Webber CA, Hocking JC, Yong VW, Stange CL, McFarlane S (2002) Metalloproteases and guidance of retinal axons in the developing visual system. J Neurosci 22(18):8091–8100
Levi E, Fridman R, Miao HQ, Ma YS, Yayon A, Vlodavsky I (1996) Matrix metalloproteinase 2 releases active soluble ectodomain of fibroblast growth factor receptor 1. Proc Natl Acad Sci U S A 93(14):7069–7074
Lin K-T, Sloniowski S, Ethell DW, Ethell IM (2008) Ephrin-B2-induced cleavage of EphB2 receptor is mediated by matrix metalloproteinases to trigger cell repulsion. J Biol Chem 283(43):28969–28979. doi:10.1074/jbc.M804401200
Gonthier B, Koncina E, Satkauskas S, Perraut M, Roussel G, Aunis D, Kapfhammer JP, Bagnard D (2009) A PKC-dependent recruitment of MMP-2 controls semaphorin-3A growth-promoting effect in cortical dendrites. PLoS One 4(4):8
Gonthier B, Nasarre C, Roth L, Perraut M, Thomasset N, Roussel G, Aunis D, Bagnard D (2007) Functional interaction between matrix metalloproteinase-3 and semaphorin-3C during cortical axonal growth and guidance. Cereb Cortex 17(7):1712–1721. doi:10.1093/cercor/bhl082
Vogt K, Mellor J, Tong G, Nicoll R (2000) The actions of synaptically released zinc at hippocampal mossy fiber synapses. Neuron 26(1):187–196
Phillips LL, Chan JL, Doperalski AE, Reeves TM (2014) Time dependent integration of matrix metalloproteinases and their targeted substrates directs axonal sprouting and synaptogenesis following central nervous system injury. Neural Regener Res 9(4):362–376. doi:10.4103/1673-5374.128237
Fredrich M, Illing RB (2010) MMP-2 is involved in synaptic remodeling after cochlear lesion. Neuroreport 21(5):324–327
Fredrich M, Illing RB (2011) Deafferentation-induced redistribution of MMP-2, but not of MMP-9, depends on the emergence of GAP-43 positive axons in the adult rat cochlear nucleus. Neural Plast 2011:859359. doi:10.1155/2011/859359
Falo MC, Reeves TM, Phillips LL (2008) Agrin expression during synaptogenesis induced by traumatic brain injury. J Neurotrauma 25(7):769–783. doi:10.1089/neu.2008.0511
Monea S, Jordan BA, Srivastava S, DeSouza S, Ziff EB (2006) Membrane localization of membrane type 5 matrix metalloproteinase by AMPA receptor binding protein and cleavage of cadherins. J Neurosci 26(8):2300–2312
Warren KM, Reeves TM, Phillips LL (2012) MT5-MMP, ADAM-10, and N-cadherin act in concert to facilitate synapse reorganization after traumatic brain injury. J Neurotrauma 29(10):1922–1940. doi:10.1089/neu.2012.2383
Sternlicht MD, Werb Z (2001) How matrix metalloproteinases regulate cell behavior. Annu Rev Cell Dev Biol 17:463–516. doi:10.1146/annurev.cellbio.17.1.463
Stellwagen D, Malenka RC (2006) Synaptic scaling mediated by glial TNF-alpha. Nature 440(7087):1054–1059
Beattie EC, Stellwagen D, Morishita W, Bresnahan JC, Ha BK, Von Zastrow M, Beattie MS, Malenka RC (2002) Control of synaptic strength by glial TNFalpha. Science 295(5563):2282–2285
Huang EJ, Reichardt LF (2001) Neurotrophins: roles in neuronal development and function. Annu Rev Neurosci 24:677–736
Larsen PH, DaSilva AG, Conant K, Yong VW (2006) Myelin formation during development of the CNS is delayed in matrix metalloproteinase-9 and −12 null mice. J Neurosci 26(8):2207–2214
Larsen PH, Wells JE, Stallcup WB, Opdenakker G, Yong VW (2003) Matrix metalloproteinase-9 facilitates remyelination in part by processing the inhibitory NG2 proteoglycan. J Neurosci 23(35):11127–11135
Uhm JH, Dooley NP, Oh LY, Yong VW (1998) Oligodendrocytes utilize a matrix metalloproteinase, MMP-9, to extend processes along an astrocyte extracellular matrix. Glia 22(1):53–63
Larsen PH, Yong VW (2004) The expression of matrix metalloproteinase-12 by oligodendrocytes regulates their maturation and morphological differentiation. J Neurosci 24(35):7597–7603
Oh LY, Larsen PH, Krekoski CA, Edwards DR, Donovan F, Werb Z, Yong VW (1999) Matrix metalloproteinase-9/gelatinase B is required for process outgrowth by oligodendrocytes. J Neurosci 19(19):8464–8475
Fowlkes JL, Serra DM, Bunn RC, Thrailkill KM, Enghild JJ, Nagase H (2004) Regulation of insulin-like growth factor (IGF)-I action by matrix metalloproteinase-3 involves selective disruption of IGF-I/IGF-binding protein-3 complexes. Endocrinology 145(2):620–626
Fowlkes JL, Thrailkill KM, Serra DM, Suzuki K, Nagase H (1995) Matrix metalloproteinases as insulin-like growth factor binding protein-degrading proteinases. Prog Growth Factor Res 6(2–4):255–263
Del Bigio MR, Jacque CM (1995) Localization of proteinase expression in the developing rabbit brain. Brain Res Dev Brain Res 86(1–2):345–347
Skuljec J, Gudi V, Ulrich R, Frichert K, Yildiz O, Pul R, Voss EV, Wissel K et al (2011) Matrix metalloproteinases and their tissue inhibitors in cuprizone-induced demyelination and remyelination of brain white and gray matter. J Neuropathol Exp Neurol 70(9):758–769
Kotter MR, Li WW, Zhao C, Franklin RJ (2006) Myelin impairs CNS remyelination by inhibiting oligodendrocyte precursor cell differentiation. J Neurosci 26(1):328–332
John GR, Shankar SL, Shafit-Zagardo B, Massimi A, Lee SC, Raine CS, Brosnan CF (2002) Multiple sclerosis: re-expression of a developmental pathway that restricts oligodendrocyte maturation. Nat Med 8(10):1115–1121
Baron W, Colognato H, ffrench-Constant C (2005) Integrin-growth factor interactions as regulators of oligodendroglial development and function. Glia 49(4):467–479
Van den Steen PE, Proost P, Wuyts A, Van Damme J, Opdenakker G (2000) Neutrophil gelatinase B potentiates interleukin-8 tenfold by aminoterminal processing, whereas it degrades CTAP-III, PF-4, and GRO-alpha and leaves RANTES and MCP-2 intact. Blood 96(8):2673–2681
Tsai HH, Frost E, To V, Robinson S, Ffrench-Constant C, Geertman R, Ransohoff RM, Miller RH (2002) The chemokine receptor CXCR2 controls positioning of oligodendrocyte precursors in developing spinal cord by arresting their migration. Cell 110(3):373–383
Pluchino S, Quattrini A, Brambilla E, Gritti A, Salani G, Dina G, Galli R, Del Carro U et al (2003) Injection of adult neurospheres induces recovery in a chronic model of multiple sclerosis. Nature 422(6933):688–694, http://www.nature.com/nature/journal/v422/n6933/suppinfo/nature01552_S1.html
Acknowledgments
The authors acknowledge the Research Foundation Flanders (FWO-Vlaanderen, Belgium, G.05311.10) and the KU Leuven Research Council (KU Leuven, Belgium, BOF-OT/10/033). IVH is a research fellow of IWT-Vlaanderen.
Author information
Authors and Affiliations
Corresponding author
Ethics declarations
Conflict of Interest
The authors declare no conflict of interest. The founding sponsors had no role in the design of the study; in the collection, analyses, or interpretation of data; in the writing of the manuscript, and in the decision to publish the results.
Rights and permissions
About this article
Cite this article
Andries, L., Van Hove, I., Moons, L. et al. Matrix Metalloproteinases During Axonal Regeneration, a Multifactorial Role from Start to Finish. Mol Neurobiol 54, 2114–2125 (2017). https://doi.org/10.1007/s12035-016-9801-x
Received:
Accepted:
Published:
Issue Date:
DOI: https://doi.org/10.1007/s12035-016-9801-x