Abstract
Insights into the process of mathematical problem posing is a central concern in mathematics education research. However, little is known about regulative or metacognitive behaviors that are essential to understanding this process. In this study, we investigate metacognitive behavior in problem posing. We aim at (1) identifying problem-posing-specific metacognitive behaviors and (2) applying these identified metacognitive behaviors to illustrate differences in problem-posing processes. For these aims, we identified problem-posing-specific metacognitive behaviors of planning, monitoring & control, and evaluating in task-based interviews with primary and secondary pre-service teachers. As a proof of concept, the identified behaviors are applied on two selected transcript fragments to illustrate how a problem-posing-specific framework of metacognitive behavior reveals differences in problem-posing processes.
Similar content being viewed by others
Avoid common mistakes on your manuscript.
Introduction
In research on problem posing, analyzing the products, that is the posed problems, plays an important role. One reason for this is that the ability to pose problems is often assessed by the posed problems (Bonotto, 2013; Singer et al., 2017; Van Harpen & Sriraman, 2013). However, in our analyses of problem-posing processes, we found that the observable quality of processes can differ, even though the same problems are posed, as will be illustrated with two exemplary processes. One construct that has made this difference within processes tangible for us is metacognitive behavior.
At least since Flavell’s (1979) seminal work, metacognition has been a central construct of research in psychology and is an established topic of mathematics education research (Schneider & Artelt, 2010). In particular, research on problem solving has benefited from the consideration of metacognitive behavior in the past decades. There are numerous characterizations of metacognitive behavior in problem solving (Artzt & Armour-Thomas, 1992; Garofalo & Lester, 1985; Schoenfeld, 1987; Yimer & Ellerton, 2010) as well as studies on the connection between metacognitive behavior and successful problem solving (Desoete et al., 2001; Kuzle, 2013; Özsoy & Ataman, 2009; Rott, 2013).
Although problem-solving research has benefited greatly from this perspective, it is noteworthy that far less conceptual or empirical research has been conducted in the related field of problem posing. A systematic literature review in high-ranked mathematics education journals has revealed that there are only a few studies that explicitly investigate problem-posing-specific aspects of metacognitive behavior (Baumanns & Rott, 2021a). For example, activities of reflection are observed where problem posers consider solvability or the appropriateness of the posed problem for a specific target group (Kontorovich et al., 2012; Pelczer & Gamboa, 2009). Furthermore, there is a lack of a framework that explicitly addresses the analysis of metacognitive behavior in problem-posing processes. That is in particular striking as researchers often note that the field of problem posing lacks conceptual insights into the activity. Such insights would enable a better analysis and interpretation of the activity itself (Ellerton et al., 2015; Ruthven, 2020; Van Harpen & Sriraman, 2013). Similar to research on problem solving, it can be assumed that the consideration and analysis of problem-posing-specific aspects of metacognitive behavior may be a central enrichment to the field. This can contribute to a better understanding of problem-posing processes.
Based on this research desideratum, this article aims at (1) identifying problem-posing-specific aspects of metacognitive behaviors in problem-posing processes and (2) applying these identified metacognitive behaviors to illustrate differences in problem-posing processes.
Theoretical Background
Problem Posing
Similar to problem solving, problem posing is considered to be a central activity of mathematicians’ (Hadamard, 1945; Halmos, 1980; Lang, 1989). Already (Pólya, 1945) mentioned problem posing as a partial activity in the context of problem solving. Despite this recognition by mathematicians, for a long time, problem posing has received noticeably less attention in mathematics education research than problem solving. At the latest since the 1980s (Brown & Walter, 1983; Butts, 1980; Kilpatrick, 1987), problem posing has been increasingly investigated. In recent years, researchers from mathematics education have shown an increasing interest in investigating and understanding problem posing (Cai et al., 2015; Cai & Hwang, 2020; Cai & Leikin, 2020; Lee, 2020; Silver, 2013). For example, problem posing has been widely used to identify or assess mathematical creativity (Joklitschke et al., 2019; Silver, 1997; Singer & Voica, 2015; Van Harpen & Sriraman, 2013; Yuan & Sriraman, 2011).
There are numerous definitions of problem posing that conceptualize more or less equivalent activities. Silver (1994) defines problem posing as the generation of new problems and reformulation of given problems which can occur before, during, or after a problem-solving process. Stoyanova and Ellerton, (1996, p. 218) refer to problem posing as the “process by which, on the basis of mathematical experience, students construct personal interpretations of concrete situations and formulate them as meaningful mathematical problems.” Cai and Hwang (2020, p. 2) subsume under problem posing “several related types of activity that entail or support teachers and students formulating (or reformulating) and expressing a problem or task based on a particular context.”
Based on the categories by Stoyanova and Ellerton (1996), we distinguish between unstructured and structured problem-posing situations depending on the degree of given information (Baumanns & Rott, 2021b). Unstructured situations are characterized by a given naturalistic or constructed situation in which tasks can be posed without or with less restrictions, for example “Consider the following infinite sequence of digits: 123456789101112131415…999100010011002… Note that it is made by writing the base ten counting numbers in order. Ask some meaningful questions. Put them in a suitable order” (Stoyanova, 1999, p. 32). To pose meaningful questions, the structure of the situation has to be explored using mathematical knowledge and concepts. In structured situations, people are asked to pose further problems based on a specific problem, for example by varying its conditions. As structured situations are used in this study, examples can be seen in Table 2.
Metacognition
Going back all the way to Flavell (1979), who significantly influenced early research on this topic, metacognition is described as “knowledge and cognition about cognitive phenomena,” which roughly means thinking about thinking. Based on this understanding, two facets of metacognition are distinguished: (1) knowledge about cognition (Cross & Paris, 1988; Kuhn & Dean, 2004; Pintrich, 2002) and (2) regulation of cognition (Schraw & Moshman, 1995; Whitebread et al., 2009).
-
(1)
Knowledge about cognition includes declarative knowledge of strategy, task, and person (Pintrich, 2002). Strategic knowledge refers to knowledge about strategies (e.g. when solving problems) and when to apply them. Knowledge of tasks refers to knowing about different degrees of difficulty of tasks and different strategies required to solve them, for example. Person’s knowledge includes knowledge about one’s own strengths and weaknesses (e.g. in problem solving)
-
(2)
Regulation of cognition refers to procedural knowledge with regard to processes that coordinate cognition. This facet includes the activities of planning, monitoring, control, and evaluating (Pintrich, 2000). Planning refers to setting of a target goal concerning the current endeavor, the activation of prior content knowledge, and activation of metacognitive knowledge, for example knowledge about specific tasks and how to solve them. Monitoring refers to metacognitive awareness and monitoring of cognition, for example when verifying that one has understood the current task. Control refers to the selection and adaption of strategies, for example with the goal to solve a problem. Evaluating refers to activities of reflecting and judging on one’s own performance and results in the form of an outcome. These activities are not completely distinct from each other and there are different perspectives on their overlap. We follow the perspective of Pintrich (2000), who states that control is mostly conceptualized as dependent or at least highly similar to monitoring (Pintrich, 2000). By that, we also follow the approach of Cohors-Fresenborg and Kaune (2007) who summarize monitoring and control under one category (see also Schraw & Moshman, 1995). This is also consistent with past work by Pólya (1945), who indicated roughly these three activities in problem solving even before the construct metacognition was established (Cohors-Fresenborg et al., 2010)
There are attempts to identify the behavior of planning, monitoring & control, and evaluating in learning contexts (Kaune, 2006; Van der Stel et al., 2010). For example, Cohors-Fresenborg and Kaune (2007) provide a category system for classifying teachers’ and students’ metacognitive activities in class discussions. The main categories of planning, monitoring & control (which they refer to as monitoring), and evaluating (which they refer to as reflecting) are divided into several subcategories with different aspects. Table 1 summarizes the codes for the main categories of planning, monitoring, and evaluating.Footnote 1 These codes are used to identify metacognitive behavior by teachers and students through the analysis of verbal protocols of classroom interactions. In addition to metacognitive behavior, Cohors-Fresenborg and Kaune (2007) also consider (negative) discursivity in their coding scheme. Discursivity is understood as a culture in which the teacher and the students always refer to each other’s expressions, work out differences in approaches, and regulate their own understanding. Because it is an additional aspect besides metacognition, and it is specific for classroom interactions which is not analyzed in the study at hand, the aspect of discursivity will not be considered further. In the study at hand, the focus is on the process of problem posing and, therefore, investigates regulation of cognition. In particular, we want to differentiate the activities of planning, monitoring & control, and evaluating for problem posing by adapting the approach by Cohors-Fresenborg and Kaune (2007).
Metacognition is often considered in conjunction with motivation and beliefs. Zimmerman and Moylan (2009) state that proactive self-regulation depends on the presence of motivational beliefs. For example, the metacognitive activity of planning depends highly on aspects like the intrinsic interest into the current endeavor, self-efficacy perceptions, or learning goal orientation. These aspects are deeply interwoven with motivation. It follows that for a holistic view of learners’ effort, for example in problem solving, metacognitive processes should be considered in addition to motivational beliefs (Zimmerman & Moylan, 2009).
Research on Metacognition in Mathematics Education
In mathematics education research, metacognition is of immense importance (for an overview, see Schneider & Artelt, 2010). Assessing metacognitive behavior is often used to investigate the mathematical skills of participants (Mevarech & Fridkin, 2006; Van der Stel et al., 2010). For example, Van der Stel et al. (2010) found out that the quality of metacognitive behavior seems to be a predictor of the mathematical performance in the future by analyzing the regulation of cognition in thinking-aloud protocols of second- and third-year students. Most prominently, metacognition is considered in problem-solving research. Considerations on this are mainly based on the ideas of Pólya (1945). Especially Schoenfeld (1985b, 1987, 1992) emphasizes the importance of control or self-regulation in problem solving. He observed that even when students have the content knowledge necessary to solve a problem, a lack of ability to keep track of what they are doing, that is metacognitive behavior, might lead to failure in solving a problem. His theoretical and empirical analyses initiated numerous studies on metacognition in mathematics education — including the present study.
Extensive discussion has been done on characterizing metacognitive behavior in problem solving (Artzt & Armour-Thomas, 1992; Garofalo & Lester, 1985; Schoenfeld, 1985b; Yimer & Ellerton, 2010). For example, Artzt and Armour-Thomas (1992) and Schoenfeld (1985b) both identify the analysis of the problem and planning the solution as predominantly metacognitive behavior. In the analysis, selecting an appropriate way to reformulate the given problem, for example in order to make it simpler, is referred to as metacognitive behavior. The phase of planning is also metacognitive by nature as it involves controlling and self-regulating the solving process (Garofalo & Lester, 1985). Building on that, several studies investigate the relationship between metacognitive activities and successful problem solving and come to the result that successful problem solving is mostly associated with the presence of metacognitive activities (Desoete et al., 2001; Kim et al., 2013; Kuzle, 2013; Özsoy & Ataman, 2009).
Research on Metacognition in Problem Posing
Due to the relevance of considering metacognitive behavior in problem solving, it can be assumed that the analysis of metacognitive behavior could be similarly relevant in problem posing. However, the potential in this area has not been sufficiently exploited to date. As some researchers interpret problem posing as a problem-solving activity (Kontorovich et al., 2012; Silver, 1995) and since there are several established frameworks of metacognitive behavior in problem solving as described above, it is a reasonable question whether there is even a need for a separate framework for metacognitive behavior in problem posing. We follow the perspective of previous studies (Baumanns & Rott, 2022; Cruz, 2006; Pelczer & Gamboa, 2009) that argue, based on observations of problem-posing processes, that there are characteristic differences between problem-posing processes and problem-solving processes in general. Therefore, also metacognitive processes involved in problem posing differ from those in problem solving. Cognitive and metacognitive processes involved in problem posing seem to be of their own nature for which metacognitive frameworks for problem solving can only serve as a stimulus. From this derives the interest addressed in this study to investigate these problem-posing-specific aspects of metacognitive behavior.
Problem posing includes different cognitive and metacognitive processes (Baumanns & Rott, 2022; Christou et al., 2005; Koichu & Kontorovich, 2013; Pelczer & Gamboa, 2009) which are indicated in the following informally: Problem posers analyze the given situation, examine which mathematical knowledge could be relevant for this, and possibly look for structures in the situation that may lead to an interesting problem. Then, problems are posed and suitable representations of them are sought. The task may then be solved and while solving, the posers may reflect on the difficulty of the task, its appropriateness for an intended target group, or the general interest in the solution. These activities are not limited to cognitive processes, but may also contain metacognitive behavior. Cognitive behavior in problem posing is, for example, applying knowledge of previous mathematical experiences to a given problem-posing situation to pose a problem. Metacognitive behavior would be, for example, to attack a problem in order to assess whether the problem is well-defined or solvable at all.
Theoretical considerations on problem posing implicitly contain some aspects of metacognition and metacognitive regulation in particular (Carrillo & Cruz, 2016; Ghasempour et al., 2013; Kontorovich et al., 2012; Pelczer & Gamboa, 2009; Singer & Voica, 2015), yet metacognition is rarely explicitly addressed as the systematic literature review on problem-posing studies by Baumanns and Rott (2022) has revealed. The following sections address the few studies that review has identified that implicitly or explicitly address metacognitive behavior.
Voica et al. (2020) mention that they found metacognitive behavior in their study with students as they were able to analyze and reflect on their own posed problems and thinking processes which helped them to become aware of their strengths. Other studies have also pointed to the lack of metacognitive activities from the participants (Crespo, 2003; Tichá & Hošpesová, 2013). For example, Crespo (2003) writes that four of her thirteen participants “posed [problems] without solving beforehand or deeply understanding the mathematics” and they “indicate unawareness of the mathematical potential and scope of [their] problem[s]” (p. 251). Crespo (2003) identifies unawareness in her participants in the sense of a lack of reflection. This can also be interpreted as a lack of metacognitive behavior. Erkan and Kar (2022) were able to observe metacognitive factors in pre-service mathematics teachers, but these factors varied depending on the problem-posing situation. Their participants considered the strengths and weaknesses of their mathematical knowledge in order to write mathematically valid problems.
Pelczer and Gamboa (2009) as well as Kontorovich et al. (2012) specify thoughts on reflection on the posed problems. In their descriptive process model, Pelczer and Gamboa (2009) state that in the phase of evaluation, expert problem posers assess their posed problems in terms of various aspects, for example, whether further modifications are needed. In the phase of final assessment, the process of posing a problem is reflected upon and the problem itself is evaluated, for example in terms of difficulty and one’s own interest in solving it. Similarly, the framework for handling the complexity of problem-posing processes in small groups by Kontorovich et al. (2012) integrates the facet of individual considerations of aptness. Consideration of aptness includes, for example, to what extent the problem poser is satisfied with the quality of the posed problem or whether the posed problem is appropriate for a specific group of solvers.
Research Objectives
As the state of research has shown, metacognition has not been an important factor in problem-posing research. Therefore, the aim of this study is to offer a focused perspective on metacognitive behavior in problem posing. This lack of conceptual and empirical insight constitutes a desideratum which leads us to the following research objective:
-
(1)
Development of a framework for identifying problem-posing-specific aspects of metacognitive behavior (i.e. planning, monitoring & control, and evaluating) in pre-service teachers’ problem-posing processes
As a second research objective, we pursue a proof of concept to identify differences in problem-posing processes based on metacognition. The problem-posing-specific metacognitive behaviors developed in research objective (1) will be applied to selected transcript excerpts of two problem-posing processes. This proof of concept is intended to show to what extent the analysis of metacognitive behaviors in problem-posing processes with the framework developed in (1) makes differences regarding metacognitive behavior in problem-posing processes visible.
-
(2)
Application of the framework developed in (1) as a proof of concept to make differences regarding metacognitive behavior in problem-posing processes visible
Methods
Research Design for Data Collection
There are several ways to assess metacognitive behavior, for example through interviews, stimulated recall, or eye-movement registration (for an overview, see Veenman et al., 2006). A clear distinction exists between off-line methods which are carried out before or after the current endeavor (often via self-report questionnaires), and on-line methods which are carried out during the current endeavor. As on-line methods seem to be more predictive with regard to the learning performance (Veenman et al., 2006), we chose the approach of video-based content analysis on problem-posing processes of pairs of pre-service teacher students. This method is based on the commonly used assumption that we can make interpretive conclusions about participants’ cognition and metacognition from their verbal expressions working in small groups (Artzt & Armour-Thomas, 1997; Goos et al., 2002).
The interviews were conducted in pairs in the same room to create a natural communication situation and eliminate the constructed pressure to produce something mathematical for the researcher (Schoenfeld, 1985a, p. 178). Johnson and Johnson (1999) underline that cooperative learning groups such as pairs are “windows into students’ minds” (p. 213). For this reason, the interviewer avoided intervening in the interaction process. The participants were asked to speak aloud at any time during interview while posing new problems. The interviews were conducted with 64 pre-service primary and secondary mathematics teachers. Sixteen students were in the first bachelor semester, 22 in the fifth bachelor semester, and 26 in the third master semester. The students worked in pairs on one of two structured problem-posing situations, either (A) Nim game or (B) Number pyramid (see Table 2). In total, 15 processes of situation (A) and 17 processes of situation (B) that range from 9 to 25 min with an average length of 14.5 min have been recorded. The processes ended when no ideas for further problems emerged from the participants. In total, 7h 46min of video material was recorded. Four pairs of students each were in the same room under authentic university seminar conditions. A camera was positioned opposite the pairs in order to capture all of the participants’ actions. In order to accustom them to a natural communication in front of the camera, short puzzles were performed before solving the initial task and the consecutive problem posing.
Data Analysis — Assessment of Metacognitive Behavior
To answer research question (1), we conducted a qualitative content analysis (Mayring, 2000). The main categories of the metacognitive behavior of planning (P), monitoring & control (MC), and evaluating (E) stem from theoretical considerations on regulation of cognition presented above (Schraw & Moshman, 1995; Whitebread et al., 2009), and more specifically from Cohors-Fresenborg’s and Kaune’s (2007; see Table 1) considerations. Although this framework is developed for analyzing classroom interaction, it has been used successfully in paired problem-solving processes (Rott, 2014). However, because problem posing is a mathematical activity of its own kind in our observations (Baumanns & Rott, 2022), a problem-posing-specific approach was chosen for the present proof of concept. The individual problem-posing-specific characteristics were obtained data-driven through an inductive category development (Mayring, 2000). The category development had four steps: (1) As the unit of analysis, the statements of the participants on the videotaped problem-posing processes were used for the category development. To identify the statements of planning, monitoring & control, and evaluating, the 32 recorded problem-posing processes were analyzed as follows: For the category of planning, we identified participants’ statements of setting a target goal for the current problem-posing situation, activating prior mathematical knowledge that helped posing new problems, or activating metacognitive knowledge in the form of knowledge on how to pose new problems in general. For the category of monitoring & control, we identified participants’ statements of awareness and monitoring of cognition as well as selecting and adapting problem-posing strategies. For the category of evaluating, we identified participants’ statements of assessing their problem-posing process or their products, that is their posed problems (e.g. “This is a good problem because it is not too difficult and it is novel compared to the initial problem.”). (2) For each identified statement in step 1, a short description was obtained (e.g. evaluation of the posed problem based on specific characteristics). Similar descriptions within the main categories planning, monitoring & control, and evaluating were then clustered into subcategories. (3) Afterwards, the developed subcategories were revised by reanalyzing seven problem-posing processes in which particularly many and different metacognitive behaviors were observed and in which participants expressed particularly many verbalizations regarding the interpretation of metacognitive behavior. This reanalysis was used to further refine the descriptions of the categories. (4) After the categories were specified, all the video material was reviewed again in order to draw attention to possible additional categories. No new categories were found in this last step. The quality of the coding was ensured through consensual validation in team discussions which is a common method of ensuring scientific quality in qualitative research (Flick, 2007).
Only for answering research question (2), selected sections of the videotaped problem-posing processes were transcribed. The framework developed in (1) is applied as a proof on concept in the second part of the paper onto the transcripts of these selected sections of two problem-posing processes. This proof of concept is intended to make differences regarding metacognitive behavior in problem-posing processes visible. In the transcripts, the participants’ statements are reproduced verbatim. Important actions of the participants are noted in parentheses for understanding the scene. Filler words that do not affect the content have been removed for readability. For the analysis, the transcripts were first read iteratively in order to obtain a rough understanding of the text and to be able to better integrate finer sections of the text into the overall context of the text. The codes developed in research question (1) are then applied to the transcript. The coding of metacognitive behavior of
,
, and
is color-coded in
,
, and
in the style of Kaune (2006; Cohors-Fresenborg & Kaune, 2007) to illustrate the distribution of the main categories. We want to emphasize that the analyses of metacognitive behavior were not linked to the correctness of the (mathematical) content. With a wrong argumentation, metacognitive behavior can be just as visible and evaluated as with a correct argumentation.
Results
Development of a Framework for Identifying Problem-Posing-Specific Aspects of Metacognitive Behavior
We first want to establish the identified problem-posing-specific aspects of metacognitive behavior of planning, monitoring & control, and evaluating. To do that, the developed types of metacognitive behavior are described and anchor examples of observed processes with regard to the Nim game and Number pyramid (see Table 2) are presented.
Planning
In Table 3, the categories of regulation of cognition in terms of planning are presented. T1 and T2 each represent any participants in the study. In Table 3,
refers to focussing on a starting point of a given situation from which a new problem can be posed. This can be, for example, a certain condition, context, or solution structure of the given initial problem. Behavior
is reminiscent of the well-known “What-if-not”-strategy (Brown & Walter, 2005), in which a similar activity is suggested before the actual problem posing. Behavior (
) refers to activities in which participants have partly considered what knowledge they or the potential solvers of a posed problem need to have in order to be able to solve it. This behavior could have a greater significance when confronted with unstructured situations in which the necessary knowledge may not be obvious because no concrete initial problem is given. In fact, the phase of setup in the mentioned framework by Pelczer and Gamboa (2009) refers to the reflection on the knowledge needed to understand the situation. Their framework is based on unstructured situations. Finally, participants named a general procedure for the upcoming problem-posing process, e.g., first vary the initial task in multiple ways, then solving the varied tasks (
).
Monitoring
In Table 4, the categories of regulation of cognition in terms of monitoring are presented.
follows on from
and characterizes that metacognitive behavior in which participants control the problem-posing process. This occurs, for example, by suggesting to solve a posed problem first before focussing on another one. Controlling the notation or representation of the posed problems (
) refers to, for example, figures drawn to illustrate a problem or to the formulation of the specific question so that it becomes understandable and precise. We observed that participants made a modification to the initial problem and analyzed the consequences that this modification had on the newly created problem (
), for example for the solution structure or its difficulty. The code
was identified when participants analyzed the mathematical structure of the given situation in order to get to a new problem or analyzed the structure of a posed problem in order to be able the characterize it, for example with regard to its solvability or appropriateness.
Evaluating
In Table 5, the categories of regulation of cognition in terms of evaluating are presented. Assessing and reflecting on the characteristics of a posed problem (
) was seen when participants posed a problem and got an idea about how to solve it. Then, they often evaluated whether their posed problem is interesting, solvable, or appropriate for a specific target group. This behavior was already mentioned in previous studies on the problem-posing process (Baumanns & Rott, 2022; Kontorovich et al., 2012; Pelczer & Gamboa, 2009). A reflection on modifications of the posed problems (
) was observed when the posed problem lacks a specific characteristic, for example it is too easy or too difficult, it is not very interesting, or it is too similar to the initial problem.
Proof of Concept: the Cases of Tino & Ulrich and Valerie & Wenke
In this section, we will analyze two cases — one by Tino & Ulrich and the other by Valerie & Wenke — with regard to the aspects of metacognitive behavior that have been developed in the previous section. The aim of this section is to illustrate different degrees of metacognitive behavior in two problem-posing processes that have a similar product. The two selected fragments are identical in numerous features of the outer structure: All four participants are pre-service high-school teachers in their third semester of their master degree. In the analyzed fragments, they work on posing new problems for the Nim game (see Table 2). In the shown fragments, both pairs of participants pose the same problem, namely the variation of the Nim game in which the players are allowed to remove 2 or 3 stones from the table. The transcript analysis is conducted to illustrate the different degree of metacognitive behavior between those two processes in which a largely identical product is produced.
Tino & Ulrich — Analysis of the Metacognitive Behavior
The following excerpt from the process of Tino and Ulrich takes place in the first half of their process. Beforehand, they already posed, solved, and analyzed several new variations of the Nim game such as what if there are 21 stones on the table in the beginning? What if you could remove 1, 2, or 3 stones from the table? What if you also get a winning point if you have removed more stones from the table than your opponent? Then, they pose the problem that you are only allowed to remove 2 or 3 stones from the table. The development of this problem is shown in the following transcribed excerpt that takes 3m 12s. For a better visual assessment of the density of metacognitive behavior, in this transcript, the codes for the metacognitive behavior are already set and marked in color.
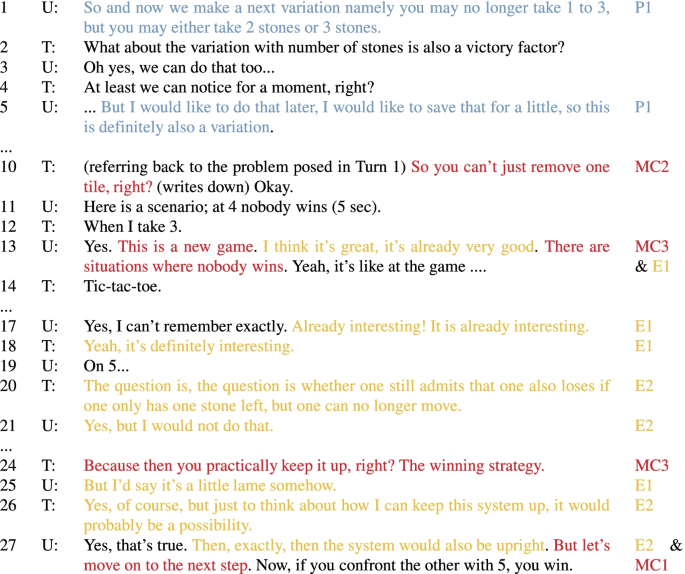
In turn 1, Ulrich poses a new variation, in which only 2 or 3 stones may be removed from the table, as starting point. This new starting point is derived from a previous task (1 to 3 pieces may be removed). Since Ulrich sets a new focus for the upcoming problem-posing activity, this statement is coded as planning (
). After Tino has thrown in what happened to one of the previous ideas, Ulrich refocuses on the problem he just posed and says that the task Tino mentions can be dealt with later. Therefore, this statement is coded as planning (
).
In turn 10, Tino tries to find a formulation for the problem that was posed in turn 1. He writes down this task as a negation that one may not just remove one stone from the table. His thinking about the formulation of the question represents a control of the notation or representation of the problem and is therefore coded as monitoring & control (
).
Ulrich says that this change results in a “new game.” He probably means a new kind of outcome of the game, where nobody wins. This assessment of the consequences that their variation has for the Nim game was coded as monitoring & control (
). Ulrich states that he likes the consequences that follow from their variation since they are different from the initial task. Therefore, this is coded as evaluation (
). In turn 18, Tino agrees with Ulrich’s positive evaluation of the game.
Tino interjects in turn 20 whether they should modify the new game due to this situation by adding that a player loses even if s/he can no longer remove stones from the table. Ulrich says that he would not make this change. In both statements, the participants consider to modify the posed problem so that the game has a definite winner. Therefore, statements related to that consideration are coded as evaluation (
).
Tino states in turn 24 that this change would restore the original winning strategy of the initial task. By that, he assesses the consequences of his slight modification and compares it to the initial task. Therefore, this statement is coded as monitoring & control (
).
Ulrich does not seem to like this change, perhaps because it would bring him too close to the initial task. In turn 13, he seemed to like this new element very much. This statement is coded as evaluation (
).
Tino reflects in turn 26 that one could modify the game with his suggestion in order to maintain the original winning strategy of the initial task. This is a reflection on their modification and, thus, is coded as evaluation (
).
Ulrich initially agrees with Tino’s previous assessment (
). Then, he focuses on a solution strategy of the modified game again and thinks about the situation in which five stones lie on the table. With his statement, he is controlling the process which is why this statement is coded as monitoring & control (
).

In turn 36, Tino now assesses the changes in the solution strategy of the new game against the background of the previously posed tasks. In the next turn, Ulrich contrasts the solution strategy of the new game against the background of the solution strategy of previous game and tries to bring both together under one mathematical thought. As both statements are reflections on the winning strategy of the new game and how it is related to the previous game, they are coded as monitoring & control (
).
Tino focuses on another variation that seems to result from the above considerations. The background could be that Ulrich’s consideration in Turn 37 is to be checked on a similar task. In that way, Tino sets a new focus for the process which is why this statement is coded as planning (
).
Overall, it can be seen that Ulrich has a controlling influence on their process (
). In both Turn 5 and Turn 27, he determines the direction in which the process should continue. First, by specifying the focus of the next considerations and then by wanting to better understand the problem that they have posed, Tino’s behavior is characterized by the fact that he wants to further modify the game that has been posed (
) so that the inevitable situation that has arisen can be avoided.
Valerie & Wenke — Analysis of the Metacognitive Behavior
The following excerpt from the process of Valerie and Wenke takes place quite early in the process. Beforehand, they both analyzed the solution to the initial problem and then asked themselves how they could now come to new problems or what they could specifically vary about the game in order to come to new problems. Afterwards, they pose the variation that you may only remove 2 or 3 stones from the table. The following excerpt takes 1m 28s and shows the creation of this problem. As in the section before, the codes for the metacognitive behavior are already set and marked in color. The coding is commented on after the transcript.
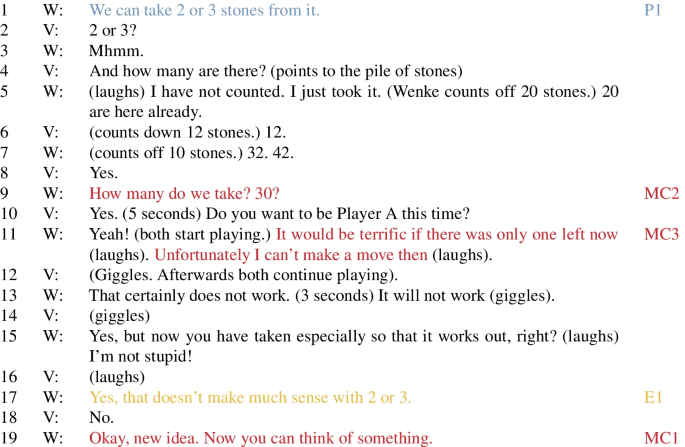
Wenke brings a new problem into the focus in turn 1. This set of a new focus is coded as planning (
). After counting how many stones they have on the table, Wenke asks in turn 9 how many stones to use for the new game. This statement is interpreted as controlling the representation of the posed problem, as the focus is already set in the form of a new game and they now have to agree on a number of stones at the beginning in order to be able to play the game (
). Wenke notices in turn 11 that the change they have posed can cause a situation in which one does not know who will win. This assessment of the consequences of the new game through their modification is coded as monitoring & control (
). In turn 17, Wenke says that their new problem does not make sense because of this new situation in which nobody wins. This statement is coded as evaluation since Wenke does not see much meaning in the new game where an undecidable situation can occur (
). Wenke obviously does not intend to pursue this problem further in turn 19 and suggests that a new problem should be posed. She has a steering effect on the process which is why this statement is coded as monitoring & control (
).
Overall, in this process, Valerie and Wenke show noticeably insecurities. This can be seen through the numerous occurrences of laughter. Nevertheless, they also show metacognitive behavior — although less frequently than Tino and Ulrich. Like Tino and Ulrich, they realize during the game that an inevitable situation could occur. In Turn 17, Wenke also evaluates this, but they see this situation as a reason to reject the posed game.
Discussion
Research Objective (1): Development of a framework for identifying problem-posing-specific aspects of metacognitive behavior (i.e. planning, monitoring & control, and evaluating) in pre-service teachers’ problem-posing processes
Tables 3, 4, and 5 summarize the identified metacognitive activities. In total, four planning activities, four monitoring & control activities, and two evaluating activities were identified. Some of these activities may be considered as cognitive, but being able to intentionally use these kinds of cognitive behavior is a sign for metacognitive abilities. However, when metacognitive behavior is mentioned here, it always means the primarily metacognitive behavior in interaction with cognitive behavior. For example, searching for a solution can be seen as cognitive behavior, but considering the solution in order to get a better idea whether the posed problem is, for example, solvable or appropriate for a specific target group can be seen as metacognitive behavior. Most of the identified activities are indeed problem-posing specific. However, there are also activities (e.g.
: Reflect necessary knowledge) that are not problem-posing specific. Moreover, not all codes (i.e. subcategories) within the superordinate categories of planning, monitoring & control, and evaluating are separable from each other. However, a clear separation between the superordinate categories should be recognizable.
We want to highlight parallels and differences between the category system for classifying teacher and students metacognitive activities in class discussions by Cohors-Fresenborg and Kaune (2007) (see Table 1) and our developed framework. The category
(indication of a focus of attention, in particular with regard to tools/methods to be used or (intermediate) results or representations to be achieved) in their system can be found in the categories
and
(see Table 3) in the problem-posing-specific framework developed here.
is considered separately as it is a central component of problem posing (Baumanns & Rott, 2022; Brown & Walter, 2005).
(express general procedure for problem posing) corresponds to a specification for problem posing of the category
in Table 1. For monitoring & control,
represents a specification for problem posing of the category
and
represents a specification for problem posing of the category
. Categories
and
were merged into the problem-posing-specific category
and categories
–
were merged into the problem-posing-specific category
. Finally, for evaluation, rather rough parallels can be drawn between categories
–
and
as well as
–
and
.
Research Objective (2): Application of the framework developed in (1) as a proof of concept to make differences regarding metacognitive behavior in problem-posing processes visible
The analysis of metacognitive behavior in the problem-posing processes of Tino and Ulrich as well as Valerie and Wenke revealed differences. Tino and Ulrich’s process had a greater frequency and density of metacognitive behavior compared to Valerie and Wenke’s process. However, the coding does not allow for a statement about the depth of the metacognitive behavior, that is how sophisticated the specific metacognitive behaviors are. For example, in both processes, the posed problem was evaluated (
and/or
). In the process by Valerie and Wenke, the evaluation remains a single mention of futility in Turn 17 from which they discard the problem. Tino and Ulrich take their thoughts further and relate their evaluation to the new possible outcome of the game, which differs from the initial task (Turns 13, 17, and 18). They even reflect on whether they want to restore similarity to the initial task by modifying it further (Turns 20, 21, 26, and 27). In contrast to Valerie and Wenke, they do not discard the problem. From a quantitative perspective, the observed processes do not claim to be representative. Therefore, a quantitative counting on the frequency of the individually occurring metacognitive behaviors would yield only insufficiently helpful new insights.
The perspective on the metacognitive behavior of both pairs reveals differences between the processes. Since in the selected excerpts the posed problems are rather identical, no differences would have been attested by looking only at the products. It also matters that Tino and Ulrich have a better understanding of the posed problem by suggesting a solution strategy as well as their motivational beliefs. However, the task did not explicitly ask for solving the posed problems. Tino’s and Ulrich’s impetus to do so independently may attest motivational beliefs that are certainly relevant in the context of metacognitive behavior.
Conclusion
The aim of the present explorative study was to investigate metacognitive behavior in problem-posing processes, which has been widely disregarded in problem-posing research. Analyses of 32 problem-posing processes of pre-service teacher students were conducted to identify metacognitive behaviors of planning, monitoring & control, and evaluating. Tables 3, 4, and 5 summarize these inductively developed categories of problem-posing-specific aspects of metacognitive behavior. In addition, two transcript excerpts were analyzed using the previously developed codes as a proof of concept to make differences regarding metacognitive behavior in problem-posing processes visible. Although, in both transcript excerpts, the product in terms of the posed problem is almost identical, the metacognitive behavior, as the analysis has shown, differs. The identified problem-posing-specific aspects of metacognitive behaviors enabled the disclosure of these differences. In addition, the analyses have shown that the consideration of metacognitive behavior allows a tentative assessment of the quality of the activity in general. This assessment is a new perspective on problem-posing processes.
Limitations of this study lie especially in the method of analyzing statements of pairs of students in a video-based content analysis to assess metacognitive behavior. As Goos et al. (2002) pointed out “student-student interactions could either help or hinder metacognitive decision making during paired problem solving, depending on students’ flexibility in sharing metacognitive roles” (p. 197). This approach can be enriched in future studies by using stimulated recall interviews. Eye-tracking can serve as a potential stimulus of such interviews. Individual interviews with a thinking-aloud approach were not used because thinking aloud, unpracticed, can interfere with the natural flow of such processes and, in particular, affect metacognitive activities (McKeown & Gentilucci, 2007), which could distort the analyses.
The framework developed in this study provides numerous opportunities for follow-up research on problem posing. As this study is based on problem-posing processes of student teachers, it would be of interest to see if additional problem-posing-specific aspects of metacognitive behaviors can be identified in a sample of, for example, pupils or expert problem posers. A larger analysis could also address the question of which metacognitive behaviors of planning, monitoring & control, and evaluating are particularly prevalent. As in research on problem solving, a comparison between metacognitive behaviors of experts and novices could reveal whether metacognitive behavior related to successful problem posing or whether there are substantial differences in metacognitive behavior in general. The data of this study was collected through structured problem-posing situations. Future studies could address the question of whether there are different metacognitive behaviors in unstructured situations or whether there are significant differences in the frequency of the different metacognitive behaviors between structured and unstructured problem-posing situations. Often, the ability to pose problems is measured by analyzing the products of a problem-posing process (Bonotto, 2013; Singer et al., 2017; Van Harpen & Sriraman, 2013). The analysis of metacognitive behavior could be used to assess the quality of problem posing on a process-oriented level. It is likely that there is a strong correlation between the products and the processes of problem posing. However, there may also be high-quality processes by means of metacognitive behavior, but whose products attest lower quality because few and non-original problems were posed. Neglected in this study was the metacognitive facet knowledge about cognition. The importance of this facet of metacognition could also be the focus of future studies. Furthermore, metacognitive processes should be considered in addition to motivational beliefs (Zimmerman & Moylan, 2009). Those beliefs could certainly enrich the comparison of the two processes. Tino and Ulrich exhibit numerous behaviors indicative of their motivational beliefs. Even before the analyzed extract, Tino and Ulrich have numerous ideas about the Nim game. In turn 5, Ulrich shows that he would like to deal with the different ideas one after the other, presumably in order to do sufficient justice to all of them. Also, their numerous evaluative statements regarding their ideas (Turn 13; 17; 18; 25) as well as thinking their ideas further (Turn 20; 21; 26; 27) are an expression of intrinsic interest in posing new problems. In Valerie and Wenke’s process, such behaviors and especially the positive reference to the activity of problem posing are largely absent. Their laughter rather speaks for a general insecurity and a tendency towards low interest in problem posing. Future studies could focus more on this interplay between metacognitive behavior and motivational beliefs.
For teaching in school and university settings, these study’s findings can be used to plan problem-posing activities in the classroom (cf. Kontorovich et al., 2012). For example, students could be encouraged to engage in metacognitive behavior during problem posing through appropriate construction of the problem-posing situation by the teacher. The teacher could ask the students to solve their posed problems or they could encourage reflection on the tasks by requiring students to pose tasks of varying difficulty for their classmates.
This study provides a first, qualitative insight into metacognitive behavior in problem posing. We hope that the perspective of metacognition will stimulate further studies in the field of problem posing research to gain further insights.
Notes
The current version of this coding manual can be found at https://www.mathematik.uni-osnabrueck.de/fileadmin/didaktik/Projekte_KM/Kategoriensystem_EN.pdf.
References
Artzt, A. F., & Armour-Thomas, E. (1992). Development of a cognitive-metacognitive framework for protocol analysis of mathematical problem solving in small groups. Cognition and Instruction, 9(2), 137–175. https://doi.org/10.1207/s1532690xci0902_3
Artzt, A. F., & Armour-Thomas, E. (1997). Mathematical problem solving in small groups: Exploring the interplay of students’ metacognitive behaviors, perceptions, and ability levels. The Journal of Mathematical Behavior, 16(1), 63–74. https://doi.org/10.1016/S0732-3123(97)90008-0
Baumanns, L., & Rott, B. (2021a). Developing a framework for characterizing problem-posing activities: A review. Research in Mathematics Education, 24(1), 28–50. https://doi.org/10.1080/14794802.2021.1897036
Baumanns, L., & Rott, B. (2021b). Rethinking problem-posing situations: A review. Investigations in Mathematics Leaning, 13(2), 59–76. https://doi.org/10.1080/19477503.2020.1841501
Baumanns, L., & Rott, B. (2022). The process of problem posing: Development of a descriptive phase model of problem posing. Educational Studies in Mathematics, 110, 251–269. https://doi.org/10.1007/s10649-021-10136-y
Bonotto, C. (2013). Artifacts as sources for problem-posing activities. Educational Studies in Mathematics, 83(1), 37–55. https://doi.org/10.1007/s10649-012-9441-7
Brown, S. I., & Walter, M. I. (1983). The art of problem posing. Franklin Institute Press.
Brown, S. I., & Walter, M. I. (2005). The art of problem posing (3rd edn.). Lawrence Erlbaum Associates.
Butts, T. (1980). Posing problems properly. In S. Krulik & R. E. Reys (Eds.), Problem solving in school mathematics (pp. 23–33). NCTM.
Cai, J., & Hwang, S (2020). Learning to teach through mathematical problem posing: Theoretical considerations, methodology, and directions for future research. International Journal of Educational Research, 102, 1–8. https://doi.org/10.1016/j.ijer.2019.01.001
Cai, J., Hwang, S., Jiang, C., & Silber, S (2015). Problem-posing research in mathematics education: Some answered and unanswered questions. In F. M. Singer, N. F. Ellerton, & J. Cai (Eds.), Mathematical problem posing. from research to effective practice (pp. 3–34). Springer.
Cai, J., & Leikin, R. (2020). Affect in mathematical problem posing: Conceptualization, advances, and future directions for research. Educational Studies in Mathematics, 105, 287–301. https://doi.org/10.1007/s10649-020-10008-x
Carrillo, J., & Cruz, J (2016). Problem-posing and questioning: Two tools to help solve problems. In P. Felmer, E. Pehkonen, & J. Kilpatrick (Eds.), Posing and Solving Mathematical. Problems Advances and New Perspectives (pp. 24–36). Springer.
Christou, C., Mousoulides, N., Pittalis, M., Pitta-Pantazi, D., & Sriraman, B. (2005). An empirical taxonomy of problem posing processes. ZDM – Mathematics Education, 37(3), 149–158. https://doi.org/10.1007/s11858-005-0004-6
Cohors-Fresenborg, E., & Kaune, C. (2007). Modelling classroom discussions and categorizing discursive and metacognitive activities. In D. Pitta-Pantazi & G. Philippou (Eds.), Proceedings of the Fifth Congress of the European Society for Research in Mathematics Education (pp. 1180–1189). ERME.
Cohors-Fresenborg, E., Kramer, S., Pundsack, F., Sjuts, J., & Sommer, N. (2010). The role of metacognitive monitoring in explaining differences in mathematics achievement. ZDM – Mathematics Education, 42(2), 231–244. https://doi.org/10.1007/s11858-010-0237-x
Crespo, S. (2003). Learning to pose mathematical problems: Exploring changes in preservice teachers’ practices. Educational Studies in Mathematics, 52(3), 243–270. https://doi.org/10.1023/A:1024364304664
Cross, D. R., & Paris, S. G. (1988). Developmental and instructional analyses of children’s metacognition and reading comprehension. Journal of Educational Psychology, 80(2), 131–142. https://doi.org/10.1037/0022-0663.80.2.131
Cruz, M. (2006). A mathematical problem-formulating strategy. International Journal for Mathematics Teaching and Learning, 79–90.
Desoete, A., Roeyers, H., & Buysse, A. (2001). Metacognition and mathematical problem solving in grade 3. Journal of Learning Disabilities, 34(5), 435–447. https://doi.org/10.1177/002221940103400505
Ellerton, N. F., Singer, F. M., & Cai, J. (2015). Problem posing in mathematics: Reflecting on the past, energizing the present, and foreshadowing the future. In F. M. Singer, N. F. Ellerton, & J. Cai (Eds.), Mathematical Problem Posing. From Research to Effective Practice (pp. 547–556). Springer.
Erkan, B., & Kar, T. (2022). Pre-service mathematics teachers’ problem-formulation processes: Development of the revised active learning framework. Journal of Mathematical Behavior, 65, 100918. https://doi.org/10.1016/j.jmathb.2021.100918
Flavell, J. H. (1979). Metacognition and cognitive monitoring. A new area of cognitive-developmental inquiry. American Psychologist, 34(10), 906–911.
Flick, U. (2007). Managing quality in qualitative research. Sage.
Garofalo, J., & Lester, F. K. (1985). Metacognition, cognitive monitoring, and mathematical performance. Journal for Research in Mathematics Education, 16(3), 163–176.
Ghasempour, A. Z., Baka, M. N., & Jahanshahloo, G. R. (2013). Mathematical problem posing and metacognition: A theoretical framework. International Journal of Pedagogical Innovations, 1(2), 63–68.
Goos, M., Galbraith, P., & Renshaw, P. A. (2002). Socially mediated metacognition: Creating collaborative zomes of proximal development in small group problem solving. Educational Studies in Mathematics, 49, 192–223. https://doi.org/10.1023/A:1016209010120
Hadamard, J. (1945). The psychology of invention in the mathematical field. Dover Publications.
Halmos, P. R. (1980). The heart of mathematics. The American Mathematical Monthly, 87(7), 519–524.
Johnson, D., & Johnson, R. (1999). Learning together and alone: Cooperative, competitive and individualistic learning. Prentice Hall.
Joklitschke, J., Baumanns, L., & Rott, B. (2019). The intersection of problem posing and creativity: A review. In M. Nolte (Ed.), Including the highly gifted and creative students – Current ideas and future directions. Proceedings of the 11th International Conference on Mathematical Creativity and Giftedness (MCG11) (pp. 59–67). WTM.
Kaune, C. (2006). Reflection and metacognition in mathematics education – Tools for the improvement of teaching quality. ZDM – Mathematics Education, 38(4), 350–360. https://doi.org/10.1007/BF02652795
Kilpatrick, J. (1987). Problem formulating: Where do good problems come from? In A. H. Schoenfeld & J. Kilpatrick (Eds.) Cognitive science and mathematics education (pp. 123–147). Lawrence Erlbaum Associates.
Kim, Y. R., Park, M. S., Moore, T. J., & Varma, S. (2013). Multiple levels of metacognition and their elicitation through complex problem-solving tasks. The Journal of Mathematical Behavior, 37, 337–396. https://doi.org/10.1016/j.jmathb.2013.04.002
Koichu, B., & Kontorovich, I. (2013). Dissecting success stories on mathematical problem posing: A case of the billiard task. Educational Studies in Mathematics, 83(1), 71–86. https://doi.org/10.1007/s11251-012-9254-1
Kontorovich, I., Koichu, B., Leikin, R., & Berman, A. (2012). An exploratory framework for handling the complexity of mathematical problem posing in small groups. The Journal of Mathematical Behavior, 31(1), 149–161. https://doi.org/10.1016/j.jmathb.2011.11.002
Kuhn, D., & Dean, D. (2004). Metacognition: A bridge between cognitive psychology and educational practice. Theory into Practice, 43(4), 268–273. https://doi.org/10.1207/s15430421tip4304_4
Kuzle, A. (2013). Patterns of metacognitive behavior during mathematics problem-solving in a dynamic geometry environment. International Electronic Journal of Mathematics Education, 8(1), 20–40.
Lang, S. (1989). Faszination Mathematik – Ein Wissenschaftler stellt sich der Öffentlichkeit [The fascination of mathematics – A scientist faces the public]. Vieweg.
Lee, S.-Y. (2021). Research status of mathematical problem posing in mathematics education journals. International Journal of Science and Mathematics Education, 19, 1677–1693. https://doi.org/10.1007/s10763-020-10128-z
Mayring, P. (2000). Qualitative content analysis. Forum: Qualitative Social Research, 1(2), Art. 20.
McKeown, R. G., & Gentilucci, J. L. (2007). Think-aloud strategy: Metacognitive development and monitoring comprehension in the middle school second-language classroom. Journal of Adolescent & Adult Literacy, 51(2), 136–147. https://doi.org/10.1598/JAAL.51.2.5
Mevarech, Z., & Fridkin, S. (2006). The effects of IMPROVE on mathematical knowledge, mathematical reasoning and meta-cognition. Metacognition and Learning, 1(1), 85–97. https://doi.org/10.1007/s11409-006-6584-x
Özsoy, G., & Ataman, A. (2009). The effect of metacognitive strategy training on mathematical problem solving achievement. International Electronic Journal of Elementary Education, 1(2), 67–82.
Pelczer, I., & Gamboa, F. (2009). Problem posing: Comparison between experts and novices. In M. Tzekaki, M. Kaldrimidou, & H. Sakonidis (Eds.), Proceedings of the 33 Conference of the International Group for the Psychology of Mathematics Education (Vol. 4, pp. 353–360). PME.
Pintrich, P. R. (2000). The role of goal orientation in self-regulated learning. In M. Boekaerts, P. R. Pintrich, & M. Zeidner (Eds.), Handbook of self-regulation (pp. 451–529). Academic Press.
Pintrich, P. R. (2002). The role of metacognitive knowledge in learning, teaching, and assessing. Theory Into Practice, 41(4), 219–225. https://doi.org/10.1207/s15430421tip4104_3
Pólya, G. (1945). How to solve it. University Press.
Rott, B. (2013). Process regulation in the problem-solving processes of fifth graders. CEPS Journal, 3(4), 25–39. https://doi.org/10.26529/cepsj.221
Rott, B. (2014). Mathematische Problembearbeitungsprozesse von Fünftklässlern – Entwicklung eines deskriptiven Phasenmodells [Mathematical problem-solving processes of fifth graders - development of a descriptive phase model]. Journal für Mathematik-Didaktik, 35(2), 251–282. https://doi.org/10.1007/s13138-014-0069-2
Ruthven, K. (2020). Problematising learning to teach through mathematical problem posing. International Journal of Educational Research, 102, 1–7. https://doi.org/10.1016/j.ijer.2019.07.004
Schneider, W., & Artelt, C (2010). Metacognition and mathematics education. ZDM – Mathematics Education, 42(2), 149–161. https://doi.org/10.1007/s11858-010-0240-2
Schoenfeld, A. H. (1985a). Making sense of “out loud” problem-solving protocols. The Journal of Mathematical Behavior, 4, 171–191.
Schoenfeld, A. H. (1985b). Mathematical problem solving. Academic Press.
Schoenfeld, A. H. (1987). What’s all the fuss about metacognition? In A. H. Schoenfeld (Ed.) Cognitive science and mathematics education (pp. 189–215). Lawrence Erlbaum Associates.
Schoenfeld, A. H. (1992). Learning to think mathematically: Problem solving, metacognition and sense-making in mathematics. In D. Grouws (Ed.), Handbook of research on mathematics teaching and learning (pp. 334–370). MacMillan.
Schraw, G., & Moshman, D. (1995). Metacognitive theories. Educational Psychology Review, 7(4), 351–371. https://doi.org/10.1007/BF02212307
Schupp, H. (2002). Thema mit Variationen. Aufgabenvariationen im Mathematikunterricht [Theme with variations. Task variations in mathematics lessons]. Franzbecker.
Silver, E. A. (1994). On mathematical problem posing. For the Learning of Mathematics, 14(1), 19–28.
Silver, E. A. (1995). The nature and use of open problems in mathematics education: Mathematical and pedagogical perspectives. ZDM – Mathematics Education, 27(2), 67–72.
Silver, E. A. (1997). Fostering creativity through instruction rich in mathematical problem solving and problem posing. ZDM – Mathematics Education, 29(3), 75–80. https://doi.org/10.1007/s11858-997-0003-x
Silver, E. A. (2013). Problem-posing research in mathematics education: Looking back, looking around, and looking ahead. Educational Studies in Mathematics, 83(1), 157–162. https://doi.org/10.1007/s10649-013-9477-3
Singer, F. M., & Voica, C (2015). Is problem posing a tool for identifying and developing mathematical creativity? In F. M. Singer, N. F. Ellerton, & J. Cai (Eds.), Mathematical Problem Posing. From Research to Effective Practice (pp. 141–174). Springer.
Singer, F. M., Voica, C., & Pelczer, I. (2017). Cognitive styles in posing geometry problems: Implications for assessment of mathematical creativity. ZDM – Mathematics Education, 49(1), 37–52. https://doi.org/10.1007/s11858-016-0820-x
Stoyanova, E. (1997). Extending and exploring students’ problem solving via problem posing (Doctoral dissertation). Edith Cowan University.
Stoyanova, E. (1999). Extending students’ problem solving via problem posing. Australian Mathematics Teacher, 55(3), 29–35.
Stoyanova, E., & Ellerton, N. F (1996). A framework for research into students’ problem posing in school mathematics. In P. C. Clarkson (Ed.), Technology in mathematics education (pp. 518–525). Mathematics Education Research Group of Australasia.
Tichá, M, & Hošpesová, A (2013). Developing teachers’ subject didactic competence through problem posing. Educational Studies in Mathematics, 83(1), 133–143. https://doi.org/10.1007/s10649-012-9455-1
Van der Stel, M., Veenman, M. V. J., Deelen, K., & Haenen, J. (2010). The increasing role of metacognitive skills in math: A cross-sectional study from a developmental perspective. ZDM – Mathematics Education, 42(2), 219–229.
Van Harpen, X., & Sriraman, B. (2013). Creativity and mathematical problem posing: An analysis of high school students’ mathematical problem posing in China and the USA. Educational Studies in Mathematics, 82(2), 201–221. https://doi.org/10.1007/s10649-012-9419-5
Veenman, M. V. J., Van Hout-Wolters, B. H. A. M., & Afflerbach, P. (2006). Metacognition and learning: Conceptual and methodological considerations. Metacognition Learning, 1, 3–14. https://doi.org/10.1007/s11409-006-6893-0
Voica, C., Singer, F. M., & Stan, E. (2020). How are motivation and self-efficacy interacting in problem-solving and problem-posing?. Educational Studies in Mathematics, 105(3), 487–517.https://doi.org/10.1007/s10649-020-10005-0
Whitebread, D., Coltman, P., Pasternak, D. P., Sangster, C., Grau, V., Bingham, S., Almeqdad, Q., & Demetriou, D. (2009). The development of two observational tools for assessing metacognition and self-regulated learning in young children. Metacognition and Learning, 4(1), 63–85. https://doi.org/10.1007/s11409-008-9033-1
Yimer, A., & Ellerton, N. F. (2010). A five-phase model for mathematical problem solving: Identifying synergies in pre-service-teachers’ metacognitive and cognitive actions. ZDM – Mathematics Education, 42(2), 245–261. https://doi.org/10.1007/s11858-009-0223-3
Yuan, X., & Sriraman, B. (2011). An exploratory study of relationships between students’ creativity and mathematical problem-posing abilities. In B. Sriraman, K. H. Lee, & X. Yuan (Eds.), The Elements of Creativity and Giftedness in Mathematics (pp. 5–28). Sense Publishers.
Zimmerman, B. J., & Moylan, A. R. (2009). Self-regulation. In J. Hacker, J. Douglas, A. C. Graesser, & B. J. Zimmerman (Eds.), Where metacognition and motivation intersect (pp. 299–315). Routledge.
Funding
Open Access funding enabled and organized by Projekt DEAL.
Author information
Authors and Affiliations
Corresponding author
Ethics declarations
Ethical approval
Informed consent was obtained from all participants included in the study.
Data handling and analysis followed the rights of the General Data Protection Regulation (Datenschutz-Grundverordnung - DSGVO).
Additional information
Publisher’s Note
Springer Nature remains neutral with regard to jurisdictional claims in published maps and institutional affiliations.
Rights and permissions
Open Access This article is licensed under a Creative Commons Attribution 4.0 International License, which permits use, sharing, adaptation, distribution and reproduction in any medium or format, as long as you give appropriate credit to the original author(s) and the source, provide a link to the Creative Commons licence, and indicate if changes were made. The images or other third party material in this article are included in the article's Creative Commons licence, unless indicated otherwise in a credit line to the material. If material is not included in the article's Creative Commons licence and your intended use is not permitted by statutory regulation or exceeds the permitted use, you will need to obtain permission directly from the copyright holder. To view a copy of this licence, visit http://creativecommons.org/licenses/by/4.0/.
About this article
Cite this article
Baumanns, L., Rott, B. Identifying Metacognitive Behavior in Problem-Posing Processes. Int J of Sci and Math Educ 21, 1381–1406 (2023). https://doi.org/10.1007/s10763-022-10297-z
Received:
Accepted:
Published:
Issue Date:
DOI: https://doi.org/10.1007/s10763-022-10297-z