Abstract
This work focuses on a way to integrate the social, environmental and economic aspects (socio-eco-efficient aspects) together in the decisions concerning the sustainable process synthesis of a lignocellulosic (Agave bagasse) biorefinery. This challenge is addressed by the formulation and solution of a multiobjective optimization model of a process superstructure for the lignocellulosic biomass conversion into biofuels and products, where social (human toxicity potential), economic (cost), environmental (environmental impact) and socioeconomic (product demand) criteria are included in the formulation of the objective function. The optimization resulted model is a MINLP (Mixed Integer Non-Linear Programing) problem. To solve this problem, a process synthesis methodology is proposed, which includes the epsilon constraint (ε-constraint) method, the use of the GAMS software and a benchmarking using Aspen Plus process simulator to include the energy balance of the different processes. As results, four feasible configurations of biorefineries were obtained, including the best configuration selected after the benchmarking using energy requirements, reduced use of energy after integration and water used as indicators. With the approach proposed in this paper, it was possible to evaluate in a short time a vast number of options included in the superstructure, as well as to select the best option that fulfills the three aspects of sustainability.











Similar content being viewed by others
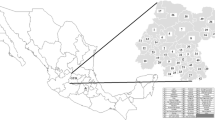
Abbreviations
- \({\text{Fobj}}\) :
-
Objective function
- \(F_{v,j}^{\text{in}}\) :
-
Input substrate flowrate to \(v_{j}\) (kg/day)
- \(F_{v,j}^{\text{out}}\) :
-
Output product flowrate from \(v_{j}\) (kg/day) \(R_{v,j}\)
- \(v_{j}\) :
-
Logic variable of decision, related to a specific processing unit
- \(W_{v,j}\) :
-
Output waste flowrate in \(v_{j}\) (kg/day)
- \({\mathcal{F}}_{v,j}^{k}\) :
-
Output flowrate of intermediate k in \(v_{j}\) (kg/day)
- \(v_{j}\) :
-
Logical decision variable (related to a specific processing unit)
- \(r_{vj}^{k}\) :
-
Yield of product k in the process \(v_{j}\)
- \(\varepsilon_{i}\) :
-
Epsilon restriction
- \({\text{COS}}\) :
-
Cost of total process resources ($)
- \({\text{Cb}}\) :
-
Biomas cost per mass unit ($/kg)
- \(C_{v,j}^{R}\) :
-
Cost of resource of process \(v_{j}\) ($)
- \(x_{v,j}^{R}\) :
-
Mass composition of resource R in process \(v_{j}\)
- \({\text{IMP}}\) :
-
Environmental impact generation
- \(x_{t,j}\) :
-
Mass composition of toxic chemical in \(v_{j}\)
- \(T_{t}\) :
-
Degree of toxicity of chemical t
- \(P_{q}\) :
-
Flowrate of product q (kg/day)
- \(x_{q}^{T}\) :
-
Mass composition of toxic product
- \(T_{q}\) :
-
Degree of toxicity of product q
- \(\rho_{q}\) :
-
Relative demand in the market of product q
- \({\text{TOX}}\) :
-
Toxic global flow
- \({\text{DEM}}\) :
-
Total demand in the market
References
Andiappan V, Ko ASY, Lau VWS, Ng LY, Ng RTL, Chemmangattupalavi NG, Ng DKS (2015) Synthesis of sustainable integrated biorefinery via reaction pathway synthesis: economic, incremental environmental burden and energy assessment with multiobjective optimization. AIChE J 61(1):132–146
Aranda JA, Orjuela JA (2015) Multiobjective optimization in biofuel supply management. A review of the literature. Ingeniería 20(1):21–47
Bozell JJ, Petersen GR (2010) Technology development for the production of biobased products from biorefinery carbohydrates—the US Department of Energy’s “Top 10” revisited. Green Chem 12:539–554
Cherubini F (2010) The biorefinery concept: using biomass instead of oil for producing energy and chemicals. Energy Convers Manag 51:1412–1421
Edgar TF, Himmelblau DM, Lasdon LS (2001) Optimization of chemical processes, 2nd edn. McGraw Hill, New York
El-Halwagi AM, Rosas C, Ponce-Ortega JM, Jiménez-Gutiérrez A, Mannan MS, El-Halwagi MM (2013) Multiobjective optimization of biorefineries with economic and safety objectives. AIChE J 59(7):2427–2434
Fernando S, Adhikari S, Chandrapal C, Murali N (2006) Biorefineries: current status, challenges and future direction. Energy Fuels 20:1727–1737
Floudas AC (1995) Nonlinear and mixed optimization: fundamental and applications. Oxford University Press, Oxford
Haimes YV, Lasdon LS, Wismer DA (1971) On a bicriterion formation of the problems of integrated system identification and system optimization. IEEE Trans Syst Man Cybern SMC 1(3):296–297
Iñiguez CG, Bernal CJJ, Ramírez MW, Villalvazo NJ (2014) Recycling Agave bagasse of Tequila industry. Adv Chem Eng Sci 4:135–142
Kravanja S (2006) The MINLP approach to structural optimization. Procedings of the 6th WSEAS. pp 49–54
Li H, Foston MB, Kumar R, Samuel R, Gao X, Hu F, Ragauskas AJ, Wyman CE (2012) Chemical composition and characterization of cellulose for Agave as a fast-growing, drought tolerant biofuels feedstock. RSC Advances 2:4951–4958
Martinkus N, Rijkhoff SAM, Hoard SA, Shi W, Smith P, Gaffner M, Wolcott M (2017) Biorefinery site selection using a stepwise biogeophysical and social analysis approach. Biomass Bioenergy 97:139–148
Mitchell JE (2002) Branch-and-cut algorithms for combinatorial optimization problems. Handbook of Applied Optimization; 65–77
Morales-Rodriguez R, Meyer AS, Gernaey KV, Sin G (2011) Dynamic model-based evaluation of process configurations for integrated operation of hydrolysis and co-fermentation for bioethanol production from lignocelluloses. Bioresour Technol 102:1174–1184
Mota B, Gomes MI, Carvalho A, Barbosa-Povoa AP (2014) Towards supply chain sustainability: economic, environmental and social design and planning. J Clean Prod 105:14–27
Othman MR, Reple J-U, Wozny G, Huang Y (2010) A Modular Approach to Sustainability Assessment and Decision Support in Chemical Process Design. Ind Eng Chem Res 49:7870–7881
Parada MP, Osseweijer P, Dique JAP (2017) Sustainable biorefineries, an analysis of practices for incorporating sustainability in biorefinery design. Ind Crops Prods 106:105–123
Pérez ATE, Camargo M, Rincón PCN, Marchant MA (2017) Key challenges and requirements for sustainable and industrialized biorefinery supply chain design and management: a bibliographic analysis. Renew Sustain Energy Rev 69:350–359
Pham V, El-Halwagi M (2012) Process Synthesis and Optimization of Biorefinery Configurations. AIChE J 58:1212–1221
Santibañez-Aguilar JE, González-Campos JB, Ponce-Ortega JM, Serna-González M, El-Halwagi M (2014) Optimal planning and site selection for distributed multiproduct biorefineries involving economic, environmental and social objectives. J Clean Prod 65:270–294
Santibañez-Aguilar JE, Morales-Rodriguez R, González-Campos JB, Ponce-Ortega JM (2016) Stochastic design of biorefinery supply chains considering economic and environmental objectives. J Clean Prod 136:224–245
Schmidt I, Meurer M, Saling P, Kicherer A, Reuter W, Gensch CO (2004) SEEbalance® Managing sustainability of products and processes with the socio-eco-efficiency analysis by BASF. Greener Manag Int 45:79–94
Yang L, Lu M, Carl S, Mayer JA, Cushman JC, Tian E, Lin H (2015) Biomass characterization of Agave and Opuntia as potential biofuel feedstocks. Biomass Bioenergy 76:43–53
You F, Tao L, Graziano DJ, Snyder SW (2011) Optimal design of sustainable cellulosic biofuel supply chains: multiobjective optimization coupled with life cycle assessment and input–output analysis. AIChE J 58:1157–1180
Acknowledegments
The authors would like to acknowledge to the Mexican National Council of Science and Technology (CONACyT).
Author information
Authors and Affiliations
Corresponding author
Rights and permissions
About this article
Cite this article
Álvarez del Castillo-Romo, A., Morales-Rodriguez, R. & Román-Martínez, A. Multiobjective optimization for the socio-eco-efficient conversion of lignocellulosic biomass to biofuels and bioproducts. Clean Techn Environ Policy 20, 603–620 (2018). https://doi.org/10.1007/s10098-018-1490-x
Received:
Accepted:
Published:
Issue Date:
DOI: https://doi.org/10.1007/s10098-018-1490-x