Abstract
For sake of improving our current understanding on soil erosion processes in the hilly–gully loess regions of the middle Yellow River basin in China, a digital elevation model (DEM)-based runoff and sediment processes simulating model was developed. Infiltration excess runoff theory was used to describe the runoff generation process while a kinematic wave equation was solved using the finite-difference technique to simulate concentration processes on hillslopes. The soil erosion processes were modelled using the particular characteristics of loess slope, gully slope, and groove to characterize the unique features of steep hillslopes and a large variety of gullies based on a number of experiments. The constructed model was calibrated and verified in the Chabagou catchment, located in the middle Yellow River of China and dominated by an extreme soil-erosion rate. Moreover, spatio-temporal characterization of the soil erosion processes in small catchments and in-depth analysis between discharge and sediment concentration for the hyper-concentrated flows were addressed in detail. Thereafter, the calibrated model was applied to the Xingzihe catchment, which is dominated by similar soil erosion processes in the Yellow River basin. Results indicate that the model is capable of simulating runoff and soil erosion processes in such hilly–gully loess regions. The developed model are expected to contribute to further understanding of runoff generation and soil erosion processes in small catchments characterized by steep hillslopes, a large variety of gullies, and hyper-concentrated flow, and will be beneficial to water and soil conservation planning and management for catchments dealing with serious water and soil loss in the Loess Plateau.










Similar content being viewed by others
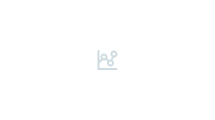
References
Beasley DB, Huggins LF, Monke EJ (1980) ANSWERS: a model for watershed planning. Trans ASAE 23(4):938–944
Bek S, Ježek J (2011) Optimization of interpolation parameters when deriving DEM from contour lines. Stoch Environ Res Risk Assess. doi:10.1007/s00477-011-0482-8
Beven KJ (1992) The future of distributed models: model calibration and uncertainty predication. Hydrol Process 6:279–298
Beven KJ (1993) Prophecy, reality and uncertainty in distributed hydrological modeling. Adv Water Resour 16:41–51
Bureau of Resource, Environmental Science and Technology, Chinese Academy of Sciences (BREST-CAS) (1992) Development and comprehensive treatment on small catchment in Loess Plateau. China Science and Technology Literature Press, Beijing
Cai QG, Lu ZX (1998) Sediment yield model and simulation in a small watershed in the hilly loess region. Science Press, Beijing
Cai QG, Lu ZX, Wang GP (1996) Physical process-based soil erosion model in a small watershed in the hilly loess region. Acta Geogr Sinica 51(2):108–117 (in Chinese with English Abstract)
Chen LD, Wang J, Fu BJ, Qiu Y (2001) Land use change in a small catchment of northern Loess Plateau, China. Agric Ecosyst Environ 86:163–172
Chen CN, Tsai CH, Tsai CT (2006) Simulation of sediment yield from watershed by physiographic soil erosion–deposition model. J Hydrol 327(3–4):293–303
De Roo APJ, Wesseling CG, Ritsema CJ (1996) LISEM: a single-event physically based hydrological and soil erosion model for drainage basins: I. Theory, input and output. Hydrol Process 10:1107–1117
Engman ET (1986) Roughness coefficients for routing surface runoff. J Irrig Drain Eng 112(1):39–53
Fu BJ (1989) Soil erosion and its control in the Loess Plateau of China. Soil Use Manag 5:76–82
Fu B, Gulinck H (1994) Land evaluation in an area of severe erosion: the Loess Plateau of China. Land Degrad Rehabil 5:33–40
Hairsine PB, Rose CW (1991) Rainfall detachment and deposition: sediment transport in the absence of flow-driven processes. Soil Sci Soc Am J 55:320–324
Li T, Wang GQ, Xue H, Wang Kai (2009) Spatial scaling issues in sediment yield and transport properties in hilly–gully loess regions. Science China: E 39(6):1095–1103
Masoudi M, Patwardhan AM, Gore SD (2006) Risk assessment of water erosion for the Qareh Aghaj subbasin, southern Iran. Stoch Environ Res Risk Assess 21(1):15–24
Misra RK, Rose CW (1989) Manual for use of program GUEST. Division of Australian Environmental Studies Report, Griffith University, Brisbane, QLD, p 1411
Morgan RPC, Quinton JN, Smith RE, Govers G, Poesen JWA, Chisci G, Torri D (1998) The EUROSEM model. In: Boardman J, Favis-Mortlock D (eds) Global change: modeling soil erosion by water. Springer, New York, pp 373–382
Murakami S, Hayashi S, Kameyama S, Watanabe M (2001) Fundamental study on sediment routing through forest and agricultural area in watershed. Ann J Hydraul Eng JSCE 45:799–804 (in Japanese)
Nearing MA, Foster GR, Lane LJ, Finkner SC (1989) A process-based soil erosion model for USDA-Water erosion prediction project technology. Trans ASAE 32(5):1587–1593
Parlange JY, Hogarth WL, Rose CW, Sander GC, Hairsine P, Lisle I (1999) Addendum to unsteady soil erosion model. J Hydrol 217:149–156
Shi H, Shao MA (2000) Soil and water loss from the Loess Plateau in China. J Arid Environ 45:9–20
Tang LQ (2003) Problems needed to be solved in sediment yield model based on physical processes. J Sediment Res 14(16):35–41 (in Chinese with English Abstract)
Tang LQ, Chen GX (1990) The numerical model of sediment yield for small loess basin. J Hohai Univ 12(6):23–28 (in Chinese with English Abstract)
Tang LQ, Chen GX (1997) A dynamic model of runoff and sediment yield from small watershed. J Hydrodyn12(2):44–54 (in Chinese with English Abstract)
Wongsa S, Nakui T, Iwai M, Shimizu Y (2002) Runoff and sediment transport modeling for mountain river. In: Proceeding of international conference on fluvial hydraulic, Belgium, River Flow, pp 683–691
World Wildlife Fund (WWF) (2004). Report suggests China’s ‘Grain-to-Green’ plan is fundamental to managing water and soil erosion. http://www.wwfchina.org/english/
Xie SN, Wang ML, Zhang R (1990) Study on storm event-based sediment yield modeling in hilly–gully loess region in the middle stream of the Yellow River. Beijing. Tsinghua University Press, Beijing (in Chinese with English Abstract)
Yang T, Zhang Y, Chen JR, He S, Xie HH (2005) A distributed hydrologic modelling in Chabagou basin of middle stream of Yellow River based on digital platform. J Hydraul Eng 36(4):456–460 (in Chinese with English Abstract)
Yang T, Zhang Y, Chen JR, Li BH (2007) Distributed soil loss evaluation and prediction modelling and simulation in an area with high and coarse sediment yield of the Yellow River, China. In: Methodology in hydrology, vol 311. IAHS Publications, China, pp 118–125
Yang T, Zhang Q, Chen YD, Tao X, Xu CY, Chen X (2008) A spatial assessment of hydrologic alteration caused by dam construction in the middle and lower Yellow River, China. Hydrol Process 22:3829–3843
Yang T, Xu CY, Chen X, Singh VP, Shao QX, Hao ZC, Tao X (2009a) Assessing the impact of conservation measures on hydrological and sediment changes in nine major catchments of the Loess Plateau. River Res Appl 24:1–19. doi:10.1002/rra.1267
Yang T, Xu CY, Shao QX, Chen X, Singh VP (2009b) Temporal and spatial patterns of low-flow hydrological components in the Yellow River during past 50 years. Stoch Environ Res Risk Assess. doi:10.1007/s00477-009-0318-y
Yao W, Tang LQ (2001) Runoff-induced soil erosion processes and modeling. The Yellow River Press (in Chinese)
Acknowledgments
The work was jointly supported by grants from the National Natural Science Foundation of China (40901016, 40830639, 40830640), a grant from the State Key Laboratory of Hydrology-Water Resources and Hydraulic Engineering (2009586612, 2009585512), the National Basic Research Program of China “973 Program” (2010CB428405, 2010CB951101, 2010CB951003), and the Fundamental Research Funds for the Central Universities (2010B00714). Cordial thanks are also extended to the editor, Professor George Christakos and three referees for their valuable comments which greatly improved the quality of this paper.
Author information
Authors and Affiliations
Corresponding author
Appendix: List of symbols
Appendix: List of symbols
- RS :
-
The infiltration excess runoff (mm/min)
- PE :
-
The precipitation minus evaporation (mm/min)
- F :
-
The infiltration rate (mm/min)
- P :
-
The precipitation (mm/min)
- f t :
-
The infiltration rate at time t (mm/min)
- f c :
-
The constant or equilibrium infiltration rate after soil has been saturated or minimum infiltration rate (mm/min)
- f 0 :
-
The initial infiltration rate (mm/min)
- k d :
-
A decay constant specific to the soil (dimensionless)
- K c :
-
The concentration routing coefficient (dimensionless)
- v :
-
The cross-sectional velocity (m/s)
- q :
-
The overland discharge per unit width (m2/s)
- h :
-
The water depth in meters (m)
- r e (t):
-
The rainfall excess rate, or lateral inflow rate (mm/min)
- l 1 :
-
The length of loess slope (m)
- l 2 :
-
The length of gully slope (m)
- l 3 :
-
The length of groove (m)
- T :
-
The duration of a storm event (min)
- x :
-
The streamwise distance (m)
- t :
-
Time (min)
- S f :
-
The friction-induced head loss per unit length between the moving fluid and the bed (m/m)
- S 0 :
-
The slope of the overland surface (m/m)
- S :
-
The slope of the flow surface (m/m)
- f :
-
The Darcy–Weisbach friction loss coefficient (dimensionless), which can be determined from the Moody diagram
- g :
-
The local gravitational acceleration, ≈ 9.8 m/s²
- R :
-
The hydraulic radius (m)
- n :
-
The Manning’s roughness (dimensionless)
- σ, λ :
-
Constant
- θ :
-
A weighting factor in the Preissmann implicit scheme (dimensionless)
- ε :
-
A weighting factor in the Preissmann implicit scheme (dimensionless)
- Ks :
-
The hydraulic roughness coefficient
- η 1 :
-
A distance related coefficient \( \left( \frac{1}{m} \right) \)
- γ :
-
The bulk density of clear water (kg/m3)
- γ s :
-
The bulk density of dry sediment (kg/m3)
- γ m :
-
The bulk density of wet sediment (kg/m3)
- e 1 :
-
The soil erosion rate of a loess slope (kg/s)
- α 1 :
-
The degree (or angle) of a loess slope (°)
- \( W_{f1} \) :
-
The effective power of soil erosion of the loess slope per unit area (W)
- τ o :
-
The shear stress (N/m2)
- τ c :
-
The critical yield stress (N/m2)
- V :
-
The average cross-sectional velocity of surface flow (m/s)
- A :
-
A non-dimensional coefficient
- \( A_{1} = \frac{A}{{g \cdot \eta_{1} \cdot tg\alpha_{1} }} \) :
-
A sediment erosion model coefficient for loess slope erosion (s2)
- γ :
-
The bulk density of the flow
- SC :
-
The sediment concentration (kg/km3)
- Q h :
-
The discharge of the clear-water flow (m3/s)
- Q c :
-
The discharge of the muddy flow (m3/s)
- J 1 :
-
The loess slope (‰)
- J 0 :
-
The slope of the surface flow (‰)
- e 2 :
-
The gully slope erosion rate (kg/s)
- \( \zeta_{2} \) :
-
The energy coefficient for gully soil erosion (dimensionless)
- h 2 :
-
The flow depth of the gully (m)
- J 2 :
-
The slope of the gully (‰)
- α 2 :
-
The degree (or angle) of the gully slope (°)
- \( A_{2} = \zeta_{2} A_{1} , \) :
-
A sediment erosion model coefficient for gully slope erosion
- \( W_{s3} \) :
-
The power of soil erosion of the groove per unit area (W)
- \( \eta_{3} \) :
-
A distance related coefficient \( \left( \frac{1}{m} \right) \) for groove erosion
- e 3 :
-
The groove soil erosion rate (kg/s)
- ω :
-
The settling velocity (cm/s)
- \( W_{f3} \) :
-
The actual power of soil erosion of the groove per unit area (W)
- U * :
-
The friction velocity (m/s)
- h 3 :
-
The groove depth (m)
- J 3 :
-
The groove slope (‰)
- κ :
-
The Karman constant
- \( \zeta_{3} \) :
-
The energy coefficient for groove soil erosion (dimensionless)
- C :
-
A dimensionless coefficient
- B 0 :
-
A dimensionless coefficient
- A 3 :
-
A sediment erosion model coefficient for groove erosion
- CE :
-
The model efficiency measure (dimensionless)
- \( \sigma_{e}^{2} \) :
-
Variance of model residuals (dimensionless)
- \( \sigma_{o}^{2} \) :
-
Variance of observations (dimensionless)
Rights and permissions
About this article
Cite this article
Yang, T., Xu, Cy., Zhang, Q. et al. DEM-based numerical modelling of runoff and soil erosion processes in the hilly–gully loess regions. Stoch Environ Res Risk Assess 26, 581–597 (2012). https://doi.org/10.1007/s00477-011-0515-3
Published:
Issue Date:
DOI: https://doi.org/10.1007/s00477-011-0515-3