Abstract
Ballistic head injury remains a significant threat to military personnel. Studying such injuries requires a model that can be used with a military helmet. This paper describes further work on a skull-brain model using skulls made from three different polyurethane plastics and a series of skull ‘fills’ to simulate brain (3, 5, 7 and 10% gelatine by mass and PermaGel™). The models were subjected to ballistic impact from 7.62 × 39 mm mild steel core bullets. The first part of the work compares the different polyurethanes (mean bullet muzzle velocity of 708 m/s), and the second part compares the different fills (mean bullet muzzle velocity of 680 m/s). The impact events were filmed using high speed cameras. The resulting fracture patterns in the skulls were reviewed and scored by five clinicians experienced in assessing penetrating head injury. In over half of the models, one or more assessors felt aspects of the fracture pattern were close to real injury. Limitations of the model include the skull being manufactured in two parts and the lack of a realistic skin layer. Further work is ongoing to address these.
Similar content being viewed by others
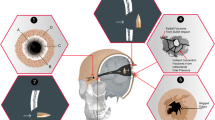
Introduction
Ballistic head injury remains a significant threat in modern conflict. Smith et al. [1] undertook a retrospective database review of patients presenting to UK field hospitals in Iraq and Afghanistan between 2003 and 2011. Eight hundred and thirteen patients on the database had suffered a penetrating head injury. Gunshot wound (GSW) was associated with a more severe injury and worse outcome than blast fragment injury. One of the study conclusions was that further work is needed to understand both the underlying anatomical lesions and the energy transfer distribution.
A further study [2] undertook a retrospective review of 71 casualties in Iraq and Afghanistan who had reached medical treatment facilities alive but subsequently died of their wounds. The most common cause of death (44 out of 71) was severe head injury from explosion, blast fragmentation and GSWs. Analysis of 42 of the patients (where full records were available) found that improved medical care would not have helped them and work should be concentrated on improving head protection.
All UK deaths on deployed operations are reviewed by a multidisciplinary panel [3]. A key output from these reviews has been identifying new injury patterns and informing the ongoing development of personal protective equipment.
Understanding and investigating these injury mechanisms and potentially suggesting improvements in head protection require suitable models.
There are many physical models used to assess head injury described in the literature. These include post mortem human specimens [4], anaesthetised animals [5], animal material [6] and synthetic materials [7].
Thali et al. [7] described development of a ‘skin-skull-brain model’ consisting of a ‘scalp’ made from silicon, a layered polyurethane sphere to represent the skull and gelatine 10% at 4 °C to simulate brain. The model was shot with a series of ammunition types and the authors reported that ‘injuries inflicted to this model are fully comparable to the morphology of equivalent real gunshot injuries’.
Raymond and Bir [8] assessed a similar model against post mortem human specimens using blunt impacts (a 103-g rigid impactor at 20 m/s) but found the fracture patterns to be different in the human bone compared to the polyurethane spheres.
Bir et al. [9] assessed two different synthetic femurs (SAWBONES® and SYNBONE®) against post mortem human material looking at both direct and indirect fractures from ballistic events and used a trained trauma surgeon to assess the injuries. The SYNBONE® produced similar fracture patterns to the human material but needed a higher direct impact velocity to create this. The SAWBONES® fracture patterns were different to the human material. The authors concluded that the bone surrogates did not approximate to the cadaveric bone under the experimental conditions used.
There are ethical and practical issues around the use of cadavers and animals which makes synthetic bone substitutes an attractive and practical option [10]. Smith et al. [10] subjected SYNBONE® polyurethane bone substitute (flat plates and spheres) to a series of ballistic impacts (0.243 in. Winchester Soft Point, 7.62 × 51 mm NATO Full Metal Jacket, 13.5 mm solid lead ball and 8.0 mm Perfectline alloy cross bow bolt) and compared both the macroscopic appearances and the microscopic damage to experimental animal bone samples and published examples of human injury. They noted clear differences between real bone and the synthetic materials but felt the SYNBONE® spheres offered a useful approximation to the damage seen in bone.
Thali et al. [7] stated that they chose a SYNBONE® sphere rather than a more complex skull form as it would offer ‘more reproducible and comparable results’. A sphere is, however, not suitable for studies incorporating helmets.
Preliminary work to develop a suitable anatomically correct skull brain model for ballistic studies incorporating a helmet have been reported [11]. This model consisted of an anatomically correct polyurethane skull with a 10% gelatine brain (by mass; 4 °C) impacted with 7.62 × 39 ammunition (M43 ball, Chinese, mild steel core, Factory 71, 1984) at a mean velocity of 675 m/s. Only six results were reported, but the fracture patterns generated were compared to the limited forensic anthropology literature that exists and demonstrated macroscopic similarities [12, 13].
The aim of this subsequent work was to assess if the fracture patterns produced under a series of further experimental conditions using simple simulants would be assessed as realistic by clinical experts.
Method
The research described in this paper was carried out in a number of stages.
Anatomically correct polymeric skulls were manufactured from rapid prototype data obtained by 3D mapping of both the internal and external surfaces of a human skull (ARRK Europe Ltd., Gloucester Technical Centre, Olympus Park, Quedgeley, Gloucester, Gloucestershire GL2 4NF).
-
a.
The first stage (n = 9 skulls) involved a comparison of skulls made from different polymers (Table 1).
The two parts of the skull were bonded using cyanoacrylate adhesive (Loctite, Henkel Corp., USA). A thin low-density polyethylene bag was inserted into the base of the skull and gelatine, 10% by mass, poured into the bag to fill the cranial cavity. The gelatine was allowed to set for 24 h at 17 °C (laboratory temperature) and then conditioned at 4 °C for a further 24 h [11].
-
b.
The second stage (n = 30 skulls) involved a comparison of different fills and conditioning temperatures. With regard to simulating brain tissue, two of the authors (PM and SH) felt that gelatine 10% seemed too stiff when compared to living brain tissue in recently ballistically injured casualties. This was the incentive to explore the behaviour of different gelatine concentrations. Gelatine 10% by mass has been used extensively in ballistic experiments, but there is still uncertainty as to how it relates to biological tissue [14].
Skulls made of polymer MU51 were filled with either gelatine made by mass to 3, 5, 7 and 10% or with PermaGel™.
The skulls were filled as described in the “Methods (item a)” section (above) and again allowed to set overnight at 17 °C. The gelatine was then either:
-
i.
conditioned for a further 24 h at 4 °C and removed from the fridge just before being shot or
-
ii.
allowed to remain at 17 °C until shot or
-
iii.
kept in an oven at 25 °C until shot.
Permagel™ is reportedly a remeltable and reusable ballistic test material equivalent to 10% gelatine, although ballistic testing with steel spheres has suggested that PermaGel™ is strain rate sensitive and its properties vary between 10 and 20% gelatine [15]. Permagel™ is melted at 110 °C, and therefore, a thin oven ‘roasting bag’ was used to contain the molten Permagel™ rather than the polyethylene bag used for the liquid gelatine. Once poured into the skulls, the Permagel™ was allowed to cool to 17 °C (laboratory temperature) and remained at this temperature until shot.
The different fill and temperature combinations are summarised in Table 2.
-
c.
The first nine skulls were shot with 7.62 × 39 mm Czech mild steel core ammunition (Sellier and Bellot, Prague; Factory in Zbrojovka Vlàsim, manufactured 1983, mean muzzle velocity 708 m/s, SD = 9 ms). The next 30 were shot with 7.62 × 39 mm Ukrainian mild steel core ammunition (Soviet State Factory, Lugansk, manufactured 1967, mean muzzle velocity 680 m/s, SD = 24 m/s) (Fig. 1).
The models were shot at a range of 10 m from a no. 3 Enfield proof mount fitted with an accurate barrel (Fig. 2a). Prior to each shot, the impact site on the model (Fig. 2b) was confirmed using a sighting laser. Bullet velocity was tracked using a Weibel Doppler and impacts filmed using two Phantom high speed cameras (V12 and V1212) (Fig. 2c, d).
The condition of the models in situ post impact was captured using a Nikon D3200 DSLR camera fitted with an AF-S NIKKOR 18–55-mm lens. The fractured skull pieces and gelatine or Permagel™ contents were collected post impact and the extent of the damage recorded. The temperature of the gelatine and Permagel™ was recorded immediately post impact using a calibrated digital thermometer (Table 2).
-
d.
The third stage was inviting five military and civilian clinicians with extensive experience of managing and/or assessing ballistic head injury to individually assess the fracture patterns in the skulls and score how clinically realistic they were using a four-point Likert-type scale [16] (Table 3).
The score sheet also included space for comments if the clinician wished to provide them (Fig. 3a, b).
The clinicians invited to assess the skull models have either looked after casualties with ballistic head injury, reviewed x-ray and CT images from such casualties or conducted post mortem examinations of fatalities. Two had been regular members of the Mortality Peer Review Panel, a multidisciplinary group undertaking peer review of UK military deaths including the nature of the injuries and treatment given [3]. The current study was an opportunity to harvest this extensive collective knowledge.
The backgrounds of the clinicians were two Civilian Home Office Forensic Pathologists, and a military radiologist, neurosurgeon and maxillofacial surgeon. The clinicians were briefed on the bullet type used (i.e. 7.62 × 39 mm) and that different polymers/gelatine concentrations had been shot but were not given the details of the gelatine concentrations with individual skulls. The Permagel™ brains do not degrade the way gelatine does and were presented at the assessments with the skulls. No formal training in an assessment method was given; the clinicians were invited to score the skulls based on their own prior experience.
Results
A typical fracture sequence captured with the V12 camera is shown in Fig. 4a–d.
The scores from the Likert-type scales were summarised in an Excel spreadsheet and analysed using International Business Machines Corporation’s Statistical Package for Social Services (IBM SPSS) version 23.
The free-text comments and notes made on the score sheets by the clinicians were also transcribed into an Excel spreadsheet so that comments about the wound characteristics and fracture patterns could be compared and assessed.
IBM SPSS analysis
The effect of polymer type on fracture score was determined using analysis of variance (ANOVA); homogeneity and normality of data were checked, and a significance level of 0.05 was applied. Significant differences were identified using Tukey’s honest significant difference (HSD) test. Mean and standard deviation data are provided in Table 4.
As shown, there was minimal difference among the scores for the different polymer types and the ANOVA found that polymer type did not affect fracture score (F 2,42 = 0.28, p = NS).
The effect of gelatine concentration (or use of PermaGel™) and temperature (rounded) on the fracture score was similarly assessed. For the purpose of analysis, temperatures between 17 and 19 °C were rounded to 17 °C. Mean and SD data are provided in Table 5.
Summary of Likert-type scores and free-text comments
Of the 39 skulls assessed, 23 were given a score of 3 by at least one assessor and seven a score of 4 by at least one assessor.
No skulls received the same scores from all five assessors.
Thirteen skulls had more than one score of 3 or 4 from separate assessors and are summarised in Appendix Table 7. Assessor comments, where given, are included to demonstrate the elements that they felt were or were not representative of real injury.
In addition to their overall Likert-type fracture pattern score, assessors also commented on how realistic some of the entry and exit wounds appeared, along with the impact of the post mortem cut line.
The frequency of comments is summarised in Table 6. Examples of impacted skulls are shown in Fig. 5.
Examples of impacted skulls. The skulls have been reconstructed where possible. Fracture lines are highlighted using black ink. The skull numbers correspond to those in Appendix Table 7. a Skull 12, entry wound and associated fracture lines (i); skull 12, view from above. Fracture line and exit site (ii); skull 12, exit site, looking through to rear aspect of entry wound (iii). b Skull 26, entry site and associated fracture lines (i); skull 26, view from above (ii); skull 26, exit site, looking through towards rear aspect of entry wound (iii). c Skull 28, entry site (i); skull 28, exit site, looking through towards rear aspect of entry wound (ii)
Discussion
Smith et al. [10] found that spheres filled with ballistic gelatine produced damage patterns that compared well with published examples of real gunshot trauma, similar to the findings of both Thali et al. [7] using spheres and Carr et al. [11] using synthetic skulls. In this present work, the overall fracture patterns in 23 of the 39 skulls (59%) were considered close to reality by at least one of the five assessors.
There were differences of opinion. For example, in two skulls (numbers 8 and 11), non-pathologists commented that the fracture patterns were too extreme to reflect reality. These were both scored high by one pathologist and the radiologist. The published literature includes cases of similar devastating head injury [17]. The useful observation is that experts will interpret based on past experience which needs to be matched to the injury being investigated or modelled.
The majority view from the clinicians was that many of the entrance wounds were not realistic. The ‘classical’ appearances of gunshot wounds to the skull are described in a number of forensic science and pathology text books [18–20].
A bullet penetrating the skull typically creates a round to oval shaped hole in the outer table of the bone with a large bevelled out hole on the inner table. The outer table defect usually has sharp edges with a ‘punched out’ appearance, and the inner table defect has an ‘excavated’ cone like appearance (Fig. 6). If a bullet has sufficient energy to exit the cranial cavity, a similar process occurs, except that the inner table is now the ‘entrance’ surface and the outer table the ‘exit’. Atypical appearances do occur including bevelling of entrance wounds [21].
Smith et al. [10] reported that the flat SYNBONE® samples and empty SYNBONE® spheres shot with both modern and obsolete ammunition types produced bevelled defects with similarities to those seen in real flat bone but lacked the complexity produced in real crania and that this was unsurprising given the differences in structure between real bone and the polymers used in the artificial bones. The same effect is seen here where the polyurethane material used for the skulls does not reflect the complex structure of actual cranial bone.
The bullet strike may cause direct secondary radial fractures originating from the impact sites [22]. In addition, the rapid rise in intracranial pressure from the temporary cavity in the brain tissue can cause indirect tertiary concentric fractures. If the pressures are high enough, an ‘explosive’ injury will be produced with skull comminution [18, 22]. These features are summarised in Fig. 6. The secondary and tertiary fractures in high-energy strikes can cause fragmentation of the original penetrating defects making assessment of which was the entry and exit wound complicated.
Limitations of the model
As described by Thali [7], a synthetic model that produces realistic injury patterns would be very useful for forensic reconstructions and be free of the ethical issues and biological variation inherent in using animals and cadaveric specimens [10].
The model used in the current work does have the disadvantage of the post mortem ‘cut’ line which is an inherent part of the manufacturing process (Email communication ARRK/Carr July 2016). As described by Viel et al. [23], applying Puppe’s rule in relation to ballistic skulls fractures, the pre-existing cut line impacts on fracture propagation within the model. Approaches to managing this are being explored.
Unlike Thali’s model [7], the one used in this work did not have a synthetic layer to simulate skin. A number of approaches to simulate skin have been reported in the literature [24], and work is ongoing to develop a suitable skin and soft tissue layers for this model.
Caveats
This paper only reports findings with two variants of one ammunition type. Other weapon systems or ammunition types may produce different results under these experimental conditions.
Conclusions
The aim of this work was to see if optimisation of an anatomically correct skull-brain model using simple simulants (polyurethane and gelatine or PermaGel™) would produce clinically realistic ballistic injury fracture patterns. At least one assessor out of five felt that the fracture pattern was close to real injury in over half of the models. Generally, the exit wounds were thought to be more realistic than the entry wounds. The model does have a number of limitations, and future work is planned to address the bonding between the two parts of the skull along with building realistic skin and tissue layers.
References
Smith JE, Kehoe A, Harrison SE, Russell R, Midwinter M (2014) Outcome of penetrating intracranial injuries in a military setting. Injury Int J Care Injured 45:874–878
Keene DD, Penn-Barwell JG, Wood PR, Hunt N, Delaney R, Clasper J, Russell RJ, Mahoney PF (2016) Died of wounds: a mortality review. J R Army Med Corps 162:355–360
Russell R, Hunt N, Delaney R (2014) The mortality peer review panel: a report on the deaths on operations of UK service personnel 2002-2013. J R Army Med Corps 160:150–154
Sarron JC, Dannawi M, Faure A, Caillou J-P, Da Cunha J, Robert R (2004) Dynamic effects of a 9 mm missile on cadaveric skull protected by aramid, polyethylene or aluminium plate: an experimental study. The Journal of Trauma, Infection and Critical Care 57:236–243
Lu H, Wang L, Zhong W, Rongfeng Q, Li N, You W, Su X, Zhuang Z, Cheng H, Shi J (2015) Establishment of a swine-penetrating craniocerebral gunshot wound model. J Surg Res 199:698–706
Puskas CM, Rumney DT (2003) Bilateral fractures of the coronoid processes: differential diagnosis of intra-oral gunshot trauma and scavenging using a sheep crania model. J Forensic Sci 48:1–7
Thali MJ, Kneubuehl BP, Zollinger U, Dirnhofer R (2002) The “skin-skull-brain model”: a new instrument for the study of gunshot effects. Forensic Sci Int 125:178–189
Raymond DE, Bir CA (2015) A biomechanical evaluation of skull-brain surrogates to blunt high-rate impacts to post mortem human subjects. J Forensic Sci 60:370–373
Bir C, Andrecovich C, Di Maio VJM, Dougherty PJ (2016) Evaluation of bone surrogates for indirect and direct ballistic fractures. Forensic Sci Int 261:1–7
Smith MJ, James S, Pover T, Ball N, Barnetson V, Foster B, Guy C, Rickman J, Walton V (2015) Fantastic plastic? Experimental evaluation of polyurethane bone substitutes as proxies for human bone in trauma simulations. Legal Med 17:427–435
Carr D, Lindstrom A-C, Jareborg A, Champion S, Waddell N, Miller D, Teagle M, Horsfall I, Kieser J (2015) Development of a skull/brain model for military wound ballistics studies. Int J Legal Med 129:505–510
Betz P, Steiefel D, Hausmann R, Eisenmenger W (1997) Fractures at the base of the skull in gunshots to the head. Forensic Sci Int 86:155–161
Fenton TW, Stefan VH, Wood LA, Sauer NJ (2005) Symmetrical fracturing of the skull from midline contact gunshot wounds: reconstruction of individual death histories from skeletonized human remains. J Forensic Sci 50:1–12
Mabbott A, Carr DJ, Champion S, Malbon C (2016) Comparison of porcine thorax to gelatine blocks for wound ballistics studies. Int J Legal Med 130:1353–1362
Mabbott A, Carr DJ, Champion S, Malbon C, Tichler C (2013) Comparison of 10% gelatine, 20% gelatine and PermaGel™ for ballistic testing. 27th International Symposium on Ballistics, Freiburg. pp 648–654
Clason DL, Dormody TJ (1994) Analyzing data measured by individual Likert-type items. J Agric Educ 35:31–35
Hejna P, Safr M, Zatopkova L (2011) Reconstruction of devastating head injuries: a useful method in forensic pathology. Int J Legal Med 125:587–590
Di Maio VJM (2016) Gunshot wounds: practical aspects of firearms, ballistics and forensic techniques, 3rd edn. CRC Press, Taylor and Francis Group, Boca Raton
Leestma IE, Kirkpatrick JB (2014) Gunshot, penetrating and blast related injuries to the nervous system. In: Leestma JE (ed) Forensic neuropathology, 3rd edn. CRC Press, Taylor and Francis Group, Boca Raton, pp 655–697
Saukko P, Knight B (2016) Gunshot and explosion deaths. In: Knights forensic pathology, 4th edn. CRC Press, Taylor and Francis Group, Boca Raton, pp 241–275
Coe JI (1982) External bevelling of entrance wounds by handguns. Am J Forensic Med Pathol 3:215–220
Karger B (2008) Forensic ballistics. In: Tsokos M (ed) Forensic pathology reviews volume 5. Humana Press, Totowa, pp 139–172
Viel G, Gehl A, Sperhake JP (2009) Intersecting fractures of the skull and gunshot wounds. Case report and literature review. Forensic Science, Medicine, and Pathology 5:22–27
Falland-Cheung L, Pittar N, Tong D, Waddell NJ (2015) Investigation of dental materials as skin stimulant for forensic skin/skull/brain model impact testing. Forensic Science, Medicine and Pathology 11:552–557
Acknowledgements
1. From Cranfield University, Defence Academy of the United Kingdom, Shrivenham, SN6 8LA:
Dr. Trevor Ringrose (statistical advice).
David Miller and Michael Teagle, the Small Arms Experimental Range.
Clare Pratchett, Art Director, CDS Learning Services (artwork for Fig. 6).
2. Craig Vickers Head of Prototyping—Europe, Arrk Europe Ltd., Gloucester.
Author information
Authors and Affiliations
Corresponding author
Appendix.
Appendix.
Rights and permissions
Open Access This article is distributed under the terms of the Creative Commons Attribution 4.0 International License (http://creativecommons.org/licenses/by/4.0/), which permits unrestricted use, distribution, and reproduction in any medium, provided you give appropriate credit to the original author(s) and the source, provide a link to the Creative Commons license, and indicate if changes were made.
About this article
Cite this article
Mahoney, P.F., Carr, D.J., Delaney, R.J. et al. Does preliminary optimisation of an anatomically correct skull-brain model using simple simulants produce clinically realistic ballistic injury fracture patterns?. Int J Legal Med 131, 1043–1053 (2017). https://doi.org/10.1007/s00414-017-1557-y
Received:
Accepted:
Published:
Issue Date:
DOI: https://doi.org/10.1007/s00414-017-1557-y