Abstract
Tangentially fired furnaces are vortex-combustion units and are widely used in steam generators of industrial plants. The present study provides a numerical investigation of the problem of turbulent reacting flows in a model furnace of a tangentially fired boiler. The importance of this problem is mainly due to its relation to large boiler furnaces used in thermal power plants. In the present work, calculation of the flow field, temperature and species concentration-contour maps in a tangentially-fired model furnace are provided. The safety of these furnaces requires that the burner be tripped (its fuel is cut off) if the flame is extinguished. Therefore, the present work provides an investigation of the influence of number of tripped burners on the characteristics of the flow and thermal fields. The details of the flow, thermal and combustion fields are obtained from the solution of the conservation equations of mass, momentum and energy and transport equations for scalar variables in addition to the equations of the turbulence model. Available experimental measurements were used for validating the calculation procedure. The results show that the vortex created due to pressure gradient at the furnace center only influenced by tripping at least two burners. However, the temperature distributions are significantly distorted by tripping any of the burners. Regions of very high temperature close to the furnace walls appear as a result of tripping the fuel in one or two of the burners. Calculated heat flux along the furnace walls are presented.










Similar content being viewed by others
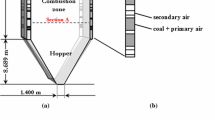
Abbreviations
- A/F:
-
Air-to-fuel ratio on a mass basis
- C 1 :
-
Constant (Eq. 6)
- C2, C3:
-
Constant (Eq. 8)
- C μ :
-
Constant (Eq. 4)
- D :
-
Diameter of the furnace used to present validation, diffusivity coefficient of species
- d i :
-
Inner diameter of air annulus
- d f :
-
Diameter of fuel nozzle
- d o :
-
Outer diameter of air annulus
- G :
-
Generation of kinetic energy of turbulence
- I :
-
Total radiation intensity
- J :
-
Diffusion flux of species
- k :
-
Kinetic energy of turbulence
- m i :
-
Mass of species
- P :
-
Pressure
- R :
-
Mass rate of reaction
- T :
-
Temperature
- S :
-
Source term
- \(\mathop U\limits^{\_\_} \) :
-
Mean velocity
- u :
-
Fluctuating velocity component
- W :
-
Width of the furnace, 0.5 m
- x, y:
-
Coordinates of the furnace cross-section measured from the center of the furnace
- X+:
-
Wall at x=0.5 W
- X−:
-
Wall at x=−0.5 W
- Y+:
-
Wall at y=0.5 W
- Y−:
-
Wall at y=−0.5 W
- z :
-
Axial distance of furnace measured from level of burners
- ɛ:
-
Dissipation rate of turbulent kinetic energy
- μ:
-
Dynamic viscosity
- κ:
-
Absorption coefficient
- λ:
-
Wavelength
- Φ:
-
Dependent variable
- ϕ:
-
Fluctuation in the dependent variable Φ
- ρ:
-
Average density
- σ k :
-
Effective Prandtl number for k
- σɛ:
-
Effective Prandtl number for ɛ
- θ:
-
Angle of burner inclination
- –:
-
Time average
- b:
-
Blackbody
- eff:
-
Effective
- k:
-
Turbulent kinetic energy
- λ:
-
Wavelength
- Φ:
-
Dependent variable
References
Romadin VP (1973) Furnaces with corner firing tangential burners. Therm Eng 20(7):79–89
Habib MA, El-Mahallawy FM, Abdel-Hafez A, Nasseef N, (1992) Stability limits and temperature measurements in a tangentially-fired model furnace. Energy Int J 17(3):283–294
Robinson GF (1985) A three-dimensional analytical model of a large tangentially fired furnace. J Ins Energy 58:116–150
Boyd RK, Roscarel KJ, Kent JH (1985) Gas flow and mixing in a tangentially fired furnace. In: Third Australasian conference on heat & mass transfer, St. Leonards, May 13–15, pp 51–58
Liu CH, Barkelew CH (1986) Numerical analysis of a jet-stirred reactors with turbulent flows and homogeneous reactions. AICHE J 32(11):1813–1820
Launder BE, Spalding DB (1974) The numerical computation of turbulent flows. Comput Methods Appl Mech Eng 3:269–289
Chen JY, Mann AP, Kent JH (1992) Computational modelling of pulverised fuel burnout in tangentially fired furnaces. Symposium (International) on Combustion, Combustion Inst, Pittsburg, PA, USA, pp 1381–1389
Baldyga J, Pohorecki R (1995) Turbulent micromixing in chemical reactors—a Review. Chem Eng J 58:183–195
Coelho PJ, Carvalho MG (1996) Evaluation of a three-dimensional mathematical model of a power station boiler. J Gas Turbines Power 118:887–895
Chungen Yin, Sebastien Caillat, Jean-Luc Harion, Bemard Baudoin, Everest Perez (2002) Investigation of the flow, combustion, heat transfer and emissions from a 609 mw utility tangentially fired, pulverized coal boiler. Fuel 81:997–1006
Zhou Y, Dou Y, Jiang H, Hui S, Xu T (1999) Effect of furnace structure on velocity deviation tangentially fired boiler. J Xi’an Jiaotong Univ 33(6):32–35
Dou W, Zhou Y, Zhou Q, Hui S, Xu T (1999) Effect of counter—tangent stream in burners on flue gas imbalance of large tangentially fired boiler. J Xi’an Jiaotong Univ 33(6):36–38
Thou YG, Zhang MC, Xu TM, Hui SF (2001) Experimental and numerical study on the flue gas velocity deviation in a tangentially fired boiler furnace. Proc Chin Soc Electrical Eng 21(1):68–72
Ma CY, Mahmoud T, Gaskell PH, Hampertsoumian E (1999) Numerical predictions of a turbulent diffusion flame in a cylindrical combustor using eddy dissipation flamelet combustion models. Proc Instn Mech Engrs 213(C):697–705
Fan JR, Zha XD, Cen KF (2001) Study on coal combustion characteristics in a W-shaped boiler furnace. Fuel 80:373–381
Hjertager LK, Osenbroch J, Hjertager BH, Solberg T, (2000) Validation of the eddy dissipation concept for fast chemical reactions in turbulent flows. Chemical Reaction Engineering VII: CFD, 6–11 August, Quebec City, Canada
Hjertager LK, Hjertager BH, Solberg T (2001) CFD modeling of fast chemical reactions in turbulent liquid flows. Chemical Engineering Laboratory, Aalborg University Esbjerg
Yaga M, Sasada K, Yamamoto T, Aoki H, Miura T (2000) An eddy characteristic time modeling in les for gas turbine combustor mitsuru. In: Proceedings of 2000 international joint power generation conference Miami Beach, Florida, 23–26 July, 2000, pp 1–6
Golovitchev VI, Chomiak J Chalmers (1996) Analysis of main assumptions underlying extended eddy dissipation model of turbulent combustion. University Of Technology Thermo-And Fluid Dynamics, GOteborg 412 96, Sweden
Soufiani A, Djavdan E (1994) A comparison between weighted sum of gray gases and statistical narrow-band radiation models for combustion applications. Combust Flame (97):240–250
Hartmann JM, Levi Di Leon R, Taine J (1984) Line by line and narrow band statiscal model calculations for H2O. J Quant Spectrosc Radiat Trasfer 32:119
Kim KT, Menart JA, Lee HS (1991) Nongray radiative gas analyses using the S-N discrete ordinates method. ASME J Heat Transfer 113:946–952
Modest MF (1991) The weighted-sum-of-gray-gases model for arbitrary solution methods in radiative transfer. J Heat Transfer 113:651–656
Hottel HC, Sarofim AF (1967) Radiative transfer. McGraw-Hill Book Company, New York
Soufiani A, Djavdan E (1994) A comparsion between weighted sum of gray gases and statistical narrow-band radiation models for combustion application. Combust Flame 97:240–250
Denison MK, Webb BW (1995a) The spectral line-based weighted-sum-of-gray-gases model in nonisothermal nonhomogeneous media. ASME J Heat Transfer 117:359–365
Denison MK, Webb BW (1995b) Development and application of an absorption-line blackbody distribution function for C02. Int J Heat Mass Transfer 38(10):1813–1821
Denison MK, Webb BW (1995c) The spectral line-based weighted-sum-of-gray-gases model for H2O/CO2 Mixtures. ASME J Heat Transfer 117:788–792
Zhang L, Soufiani A, Taine J (1988) Spectral correlated and non-correlated radiative transfer in a finite axisymmetric system containing an absorbing and emitting real gas-particle mixture. Int J Heat Mass Transfer 31(11):2261–2272
Liu F, Becker HA, Bindar Y (1998) A comparative study of radiative heat transfer modeling in gas-fired furnaces using the simple grey and the weighted-sum-of-grey-gases models. Int J Heat Mass Transfer 41:3357–3371
Modak AT (1979) Radiation from products of combustion. Fire Res 1:339–3361
Smith TF, Shen ZF, Friedman JN (1982) Evaluation of coefficients for the weighted sum of gray gases model. J Heat Transfer 104:602–608
Truelove JS (1976) A mixed grey gas model for flame radiation, United Kingdom atomic energy authority report AERER-8494, Harwell
Reynolds WC (1987) Fundamentals of turbulence for turbulence modeling and simulation. Lecture notes for Von Karman Institute, Agard Report No. 755
Shih TH, Liou WW, Shabbir A, Zhu J (1995) A new k-ɛ eddy-viscosity model for high Reynolds number turbulent flows—model development and validation. Comput Fluids 24(3):227–238
Habib MA, Attya AE, McEligot DM (1989) Calculation of turbulent flow and heat transfer in channels with streamwise periodic flow. ASME J Turbomachinery 110:405–411
Magnussen BF, Hjertager BH (1976) On mathematical models of turbulent combustion with special emphasis on soot formation and combustion. In: 16th Symposium (Int’l.) on combustion, The Combustion Institute
Siegel R, Howell J (2002) Thermal radiation heat transfer, 4th edn. Taylor & Francis, New York
Patankar SV (1980) Numerical heat transfer and fluid flow, 1st edn. Taylor and Francis, New York
Habib MA, Whitelaw JH (1982) The calculation of turbulent flow in wide angle diffusers. Num Heat Transfer 5:145–164
Attya AM, Habib MA (1990) The influence of spray characteristics and air preheat on the flame properties in a gas turbine combustor. In: Nejat T (ed) Multi-phase transport and particulate phenomena. Hemisphere Publishers Corportion, Washington
Shuja SZ, Habib MA (1996) Fluid flow and heat transfer characteristics in axisymmetric annular diffusers. Comput Fluids 25(2):133–150
Acknowledgement
The support of King Fahd University for this research work is acknowledged.
Author information
Authors and Affiliations
Corresponding author
Rights and permissions
About this article
Cite this article
Habib, M.A., Ben-Mansour, R. & Antar, M.A. Flow field and thermal characteristics in a model of a tangentially fired furnace under different conditions of burner tripping. Heat Mass Transfer 41, 909–920 (2005). https://doi.org/10.1007/s00231-004-0593-6
Received:
Published:
Issue Date:
DOI: https://doi.org/10.1007/s00231-004-0593-6