Abstract
Objective
To compare the value of bispectral index as a monitor of sedation in critically ill children with a validated sedation scoring system.
Design
Prospective convenience sample.
Setting
Paediatric intensive care unit in a tertiary paediatric centre.
Patients and participants
Forty-three critically ill children receiving sedation and mechanical ventilation.
Measurements and results
Simultaneous recording of bispectral index (BIS) and assessment of depth of sedation using the Comfort score were performed at regular intervals. To determine if BIS could detect episodes of arousal, times of endotracheal suctioning and the corresponding BIS score were recorded. There was an overall moderate correlation between BIS scores and Comfort scores (r=0.50, r 2=0.25, p<0.0001). Children who had a neurological reason for their current admission (n=25) showed a weaker correlation (r=0.26, r 2=0.06, p<0.007) than those (n=15) with normal neurology (r=0.51, r 2=0.26, p<0.0001). There were no significant differences in the rise in BIS following endotracheal suctioning among any of the predefined depths of sedation. There was a correlation of r=0.84 (r 2=0.71) (SE of slope 0.49, CI95 1.79–3.88) for mean BIS values for each individual Comfort score from 8–23. Using Spearman's rank correlation of Comfort versus mean BIS, the correlation coefficient was r=0.92.
Conclusions
Bispectral index scores correlate with Comfort scores to a moderate degree. BIS is able to discriminate between light and deep levels of sedation, but not between deep and very deep levels of sedation. The BIS monitor may provide a useful method for assessing sedation in critically ill children, especially those receiving neuromuscular blockers.
Similar content being viewed by others
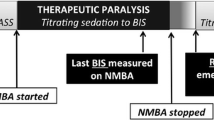
Introduction
Children present with a broad spectrum of conditions which all have differing sedative requirements. The provision of an appropriate level of sedation requires the careful administration of selected sedative agents combined with frequent assessment of the response. This allows the accurate titration of the agents to achieve the optimal level of sedation. The benefits of such an approach are good analgesia, a reduction in anxiety and agitation, enhanced synchronisation with mechanical ventilation, alleviation of the physiological response to stress and the possibility to perform diagnostic tests and other invasive procedures. The recollections of children who have been sedated and ventilated in a Paediatric Intensive Care Unit (PICU) appear to be mainly positive or neutral [1]. Over-sedation may lead to complications including cardiovascular depression, prolonged duration of mechanical ventilation [2], ventilator-associated pneumonia or lung injury [3] and the development of withdrawal symptoms on cessation [4, 5]. Early reports from Anand et al. in paediatric cardiac surgery [6] and Kress et al. in adult ICU [7] highlight the importance of adequate sedation and analgesia on influencing outcome and reducing morbidity. A recent review of current sedation practice in PICUs in the UK found that clinical sedation guidelines were only available in 45% of units, sedation was formally assessed in only 40% of units and neuromuscular blockers were administered in 31% of all cases, during which time sedation was frequently inadequately assessed [8].
Intensivists have reported that the ideal level of sedation would result in their patients being asleep but easily rousable [9]. In order to achieve this, a number of sedation scoring systems have been devised for use in the critically ill to allow more accurate titration of sedatives to specific target end points [10]. These scoring systems measure specific clinical and physiological parameters. The Comfort scale was devised as a measurement of "behavioural distress" for the specific assessment of ventilated critically ill children [11], in contrast to scoring systems initially designed for adults such as the Ramsay scale. The Comfort scale examines eight parameters, five behavioural and three physiological, and scores each with a value of 1–5 (total range 8–40) and does not require repeated disturbance of the patient unlike other scoring systems. Studies have validated its use and shown it to be reliable in all ages with differing neurodevelopment, although it can not be used in children receiving neuromuscular blocking agents [12].
The continuing development of the BIS monitor (Aspect Medical Systems) provides a potential method for the indirect measurement of the depth of sedation of a patient by analysis of the electroencephalogram (EEG). This is displayed as a single value on the screen ranging between 0 and 100, where 100 is awake. Intra-operative studies have shown the BIS monitor to be a reliable indicator of the depth of sedation or anaesthesia where most of the commonly used anaesthetic and sedative agents have been used [13, 14], with the notable exception of ketamine [15]. In children, the BIS monitor has been shown to respond in a predictable linear fashion to volatile agents [16]. Amnesia has been shown to be reliably produced with BIS scores of 64–80 [14, 17]. A recent study of 24 sedated children on a PICU with normal neurology found good correlation with 478 BIS readings and the Ramsay score used to assess sedation [18]. The results confirmed that the Ramsay score and bedside nurse assessment are inadequate for monitoring the depth of sedation in paralysed children and suggest that the bispectral index would be a useful adjunct in assessing sedation in a paralysed patient.
The following study was designed to examine the value of the BIS monitor as a reliable indicator of the depth of sedation in critically ill children with normal and abnormal neurology receiving assisted ventilation using a more validated paediatric sedation scoring system.
Method
Subjects were recruited from a PICU in a tertiary level paediatric teaching hospital. The local ethics committees approved the study. All children admitted to the intensive care unit and receiving sedation were considered for inclusion in the trial. Infants aged less than 1 month were excluded. Also excluded was any child receiving a ketamine infusion. Specially designed paediatric sensors were used and applied following appropriate preparation of the skin for optimal contact. The sensors were connected to the BIS monitor (Aspect Medical Systems, software version 3.1, Newton, MA) and were reapplied if the quality of the detected signal was not suitable to provide a reliable BIS score, i.e. a signal quality index better than 50%. The BIS scores were recorded by connecting the BIS monitor to a laptop computer via a RS/2 serial cable and live data capture performed using Hyperterminal software (Windows 98, Microsoft) as directed by the manufacturers. Electromyography (EMG) and lead impedance data were also collected.
Throughout the study repeated assessment of the level of sedation was performed using the Comfort score, during periods of stability. Each Comfort score was performed over a 2-min period of observation as recommended [11]. A score of between 8 and 40 was recorded by the nursing staff according to assessment of the eight parameters comprising the Comfort score (see Appendix A). The corresponding BIS score was determined from the mean value recorded over a 1-min period assuming a good quality signal. Also, in an attempt to limit recorder bias in this study, the nursing staff was not instructed in the significance of the displayed BIS value. During the study period, the times of endotracheal suction were recorded in order to provide a standardised deep stimulus from which ease of arousal could be assessed. The study period was continued for a minimum of 2 h up to 16 h when possible and was terminated at the request of the nursing staff when specific cares or treatments needed to be performed. The children receiving muscle relaxants were considered separately since Comfort scores could not be determined.
Baseline physiological variables, ventilator and neurological status of the children were recorded and all sedative agents administered at the time of the study documented. The use of neuromuscular blockers was also noted. Statistical analysis was performed using a software package (StatsDirect). The means ± standard deviation (SD) were used to describe the age and weight of the population. Analysis of the BIS data collected was determined after tests for normality of the data and allowed for measurements between subjects as well as multiple measurements on each subject [19, 20]. A Shapiro-Wilk W test for non-normality was used to test all data. The presence of correlation was determined using Spearman Rank correlation test and linear regression analysis. Measurement of agreement of the two methods of assessment was performed using a Bland-Altman plot. The Comfort score was converted into a percentage of its maximum score in order to compare scales. BIS scores were also divided into groups representing increasing depths of sedation; light (>80), moderate (60–80), deep (40–60), very deep (<40). The mean Comfort scores were determined for each BIS group of depth of sedation and Kruskal-Wallis multi-group analysis used to determine any difference between the groups. Finally, ease of arousal was examined in each group by determining the maximum increase in captured BIS score after the standard stimulus, endotracheal suction, had been performed. The difference between the mean BIS score for the minute preceding endotracheal suction and the peak BIS score in the following 2 min was recorded. Between groups analysis was performed using the Kruskal-Wallis test. A p value less than 0.05 was considered significant in all tests.
Results
Forty-three children were studied, three subjects were subsequently excluded: two received intermittent boluses of muscle relaxant during the study period and one received a single bolus of ketamine. A total of 373 data sets were collected from the remaining subjects. The age of the patients ranged from 1 month to 16 years (mean 3.9 years ± SD 4.5 years) and the mean weight was 15.6±14.1 kg. The distribution of male to female subjects was 25:15. The diagnostic categories for admission to the PICU included respiratory (n=12), head injury (n=5), neurology (n=5), sepsis (n=5), oncology (n=4), metabolic (n=4) and cardiac (n=3). Following case note review and clinical assessment, 25 were considered to be neurologically normal and the remaining 15 were found to have a neurological reason for current admission such as head injuries or encephalopathy. All patients were receiving mechanical ventilation and a sedative agent, of which the commonest combination was morphine (n=33) and midazolam (n=31). Other sedative agents included triclofos (n=7) and vallergan (n=3). No subject received ketamine. Six subjects received an infusion of a muscle relaxant during the study period. The data from these children were considered separately since Comfort scores could not be assessed.
Live BIS data capture was performed during each study period which ranged from 2 to 16 h. A maximum of 10 data sets were collected on each subject during this period (range 4–10). No adverse reactions to the skin probes were noted on their removal. Any BIS score recorded with a signal quality index (SQI) of less than 50 was excluded. Review of the data collected from the BIS monitor confirmed there was no evidence of excessive electromyographic (EMG) activity (EMG >40dB) in any of the cases. Data capture was stopped early in one case as the BIS monitor displayed an isoelectric EEG. The child died a few hours later.
Recorded Comfort scores ranged from 8 to 34 (mean 14.5±4.2) representing very deep to light sedation. The BIS scores collected ranged from 1 to 97 (mean 48.1±21.2). The correlation coefficient between BIS and Comfort scores for the whole group, using Spearman rank correlation, was r=0.50 (r 2=0.25, p<0.0001) (Fig. 1). No individuals were receiving muscle relaxants. As a measure of agreement, a Bland-Altman plot [21] was performed (Fig. 2). As two different measuring scales were being compared, each Comfort score was converted into a percentage of its maximum possible score, i.e. 40. The solid horizontal line represents the mean of the differences between each data pair. If the two methods of measurement were in full agreement, this line should cross the y-axis at 0. In this plot, the mean difference was 25.3±18.7 and represents the bias between the two measurement methods, i.e. the BIS score measurements were consistently at a higher part of its scale compared to the adjusted Comfort score. The limits of agreement are represented by the two horizontal lines plotted at two standard deviations either side of the mean. Less than 5% of the measurements lie outside this range and the agreement is therefore significant, but it is the size of these limits which determines whether the possible variation of the measurements is clinically relevant.
Bland-Altman Plot. The Comfort score has been converted into a percentage of its maximum score. The mean "sedation score" for each data set is plotted against the difference between the BIS and Comfort sedation scores. The horizontal solid line represents the mean of the differences ( 25.3±18.7). The horizontal dotted lines represent the limits of agreement at ± 2SD. The sloping dashed line represents the line of best fit
The line of best fit is also shown, which demonstrates that the bias is variable and appears to increase with progressively lighter sedation, i.e. higher sedation scores. The data were re-examined after dividing the group into two sub-sets based upon whether they had a neurological reason for current admission resulting in abnormal neurology or whether they had normal neurology at the time of study. The correlation coefficient between the BIS score and the Comfort score for subjects with abnormal neurology was r=0.26 (r 2=0.06, p<0.007) compared to r=0.51 (r 2=0.26, p<0.0001) (Fig. 3) in those considered to have normal neurology. Using multiple observations from each subject may tend to a falsely elevated correlation coefficient [15, 16]. To limit the contribution of inter-individual variability, correlation analysis using only the highest and lowest BIS scores with corresponding Comfort scores for each subject was also performed. This generated a correlation coefficient of r=0.51 (r 2=0.26), which was identical to that found when all data sets were included.
The Comfort scores recorded were separated into pre-defined BIS groups representing differing levels of sedation: light sedation (>80), moderate sedation (61–80), deep sedation (41–60) and very deep sedation (<40). The mean Comfort scores were determined for each category of sedation and Kruskal-Wallis multi-group analysis used to determine any significant difference between the groups. Non-parametric analysis of variance of the four groups was significant (p<0.001) and a significant difference between the groups was detected (light vs moderate, p=0.005; moderate vs deep, p<0.0001) with the exception of deep versus very deep sedation (p=0.11) (Fig. 4). In the children studied, 63% were considered appropriately sedated using the BIS score and 24% over-sedated (BIS score <40). In the very deep sedation group with a BIS score value less than 40, there was a higher incidence of head injuries (18.0 vs 11.9%) and metabolic encephalopathies (20.5 vs 4.5%) compared to the rest of the sedation groups. Of the individuals receiving muscle relaxants, 50% were deeply sedated (3/6). The mean BIS score for subjects receiving an infusion of muscle relaxant was 48.1±21.7, which was not significantly different to the mean for the other subjects (48.1±21.2).
The assessment of ease of arousal in response to endotracheal suction was also assessed. The difference between the mean BIS score for the minute preceding endotracheal suction and the peak BIS score in the following 2 min was recorded. The rise in BIS was tested against the category of sedation for the individual child. Between-group analysis was performed using the Kruskal-Wallis test. Mean increases in BIS scores from baseline following endotracheal suction were +13 for very deep, +18 for deep, +14.2 for moderate and +5.8 for light sedation. There was no significant difference in the increase in BIS among any of the groups.
Finally, using simple linear regression we correlated the mean of all the BIS values for each individual Comfort score from 8 to 23 and found a correlation coefficient of r=0.84 (r 2=0.71) (SE of slope 0.49, CI95 1.79–3.88) (Fig. 5). Using Spearman's rank correlation of Comfort versus mean BIS, a correlation coefficient of r=0.92 was obtained.
Discussion
Over-sedation may lead to increased morbidity with prolonged ventilation and duration of stay on the PICU [2, 3]. It is important to remember that achieving satisfactory sedation is also important in alleviating parental anxiety [22]. Existing sedation scoring systems rely on patient appearance, physiological variables and responses to stimuli. These may be masked when neuromuscular blockers are administered, but also the scales are exposed to the subjectivity of the practitioners using them. The BIS monitor provides the clinician with an objective assessment of the level of consciousness. Sedation can be maintained accurately when the patient is paralysed and decreased when he is weaned. The BIS monitor was designed for the assessment of intra-operative depth of anaesthesia and studies have shown good correlation between anaesthetic concentrations and BIS scores in the operating theatre [16].
The aim of this study was to determine whether the BIS monitor is a useful tool for assessing depth of sedation in children receiving intensive care and to avoid the administration of excessive amounts of sedative agents. We compared the BIS with a validated sedation scoring system for children, the Comfort score. The correlation coefficient between the BIS scores and the Comfort scoring scale observed in this study of R=0.5 (r2=0.25, p<0.0001) indicates a moderate degree of correlation between the two assessment tools. The BIS was also able to distinguish significantly between the different levels of sedation, except when deep was compared to very deep sedation.
Crain et al. studied neurologically normal children in the PICU and demonstrated good reliability between observers performing assessment and derived a similar correlation coefficient of r 2=0.26 [23]. Correlation of the BIS score with the Ramsay scale and tracheal suctioning scale in the PICU has generated r 2 values of 0.08 to 0.21 [24], respectively.
The BIS monitor was initially designed to measure the level of consciousness in adults receiving general anaesthesia and has shown good correlation for a number of agents [14]. The algorithm used in the monitor is derived from the analysis of raw EEG collected from healthy adult volunteers at specific clinically important end points, e.g. loss of consciousness. This has raised concerns as to whether the algorithm can be applied to infants and children. The EEG changes over the first few years of life as the central nervous system matures [25, 26] before reaching adult appearance after approximately 15 years [27]. The dominant frequency is observed to shift from 5 Hz in infants to 10 Hz during this time [28]. The monitor has been used in the paediatric population receiving general anaesthesia and studies have suggested a similar correlation between end tidal sevoflurane concentration and BIS scores to that observed in adults [13, 16, 29]. However, one study was not able to demonstrate this correlation in infants [27] and Bannister et al. reported difficulty in titrating sevoflurane concentrations to achieve target BIS scores again in infants [30]. A recent retrospective report has also failed to find a correlation between clinical end points during anaesthesia and BIS scores in the paediatric population [31].
The BIS monitor has been assessed in adult ICUs [32]. Validation studies have reported that excessive muscle movement, generating high EMG activity, weakened the observed correlation by generating abnormally high BIS readings [33, 34]. When this subgroup of readings was removed, there was a marked improvement in correlation from r 2=0.36 to 0.50. No elevated EMG activity was noted on any subject during our study. Indeed, the newest software released for the BIS monitor (version 4.0) has addressed this issue and reduced the interference caused by artefacts such as muscle activity [35]. Other sources of interference with BIS readings have also been reported, such as pacemaker spikes [36], external warmers [37], ketamine [38], catecholamine infusions [39] and also patient temperature [40]. In lightly sedated patients, noise has been shown to elevate the BIS score significantly [41]. The BIS monitor has also been found to be dependent on the sedative agent used. Whereas benzodiazepines and propofol have been shown to cause a dose-dependent change in the BIS score, opioids show poor correlation in adults [14, 42] and children [43].
Disturbances to the normal EEG are also observed in patients with neurological insults such as traumatic head injury or an encephalopathy. The pathological changes to the EEG in traumatic brain injury vary considerably and attempts to use raw EEG analysis to predict outcome in this group have been varied [44]. The BIS score has been found to correlate poorly with the Glasgow Coma Scale [45]. In contrast, when the BIS monitor was used on unsedated head injured adults, the BIS score was found to correlate with the severity of the neurological injury [46]. Children with head injuries or encephalopathies represent a marked proportion of the PICU population. When the results of our study were analysed for patients diagnosed with a neurological insult, the correlation between BIS scores and Comfort scores was dramatically weakened. This suggests that EEG analysis to assess pharmacological sedation may be less reliable in these children. Also, the BIS monitor does not distinguish between natural sleep and pharmacologically induced sleep. During REM sleep in adults, BIS scores of 40–45 have been reported, which are equivalent to values used to define deep sedation [47, 48]. The incidence of negative recollections following a period of therapeutic paralysis on an intensive care unit may be higher than imagined 49.
The BIS monitor has also been tested to see if specific scores could predict ease of arousal and hence used to titrate sedation to a sufficient depth prior to painful or stimulating procedures, which result in the most frequent negative recollections of children on PICUs [1]. In our study no significant difference could be identified between sedation groups in response to endotracheal suction. The elevation in BIS was similar between subjects who were deeply or lightly sedated, indicating that no specific range of BIS values could be recommended reliably to provide sufficiently deep sedation prior to such a procedure. The BIS monitor could also potentially allow continuous monitoring of sedation independent of these physiological variables and titration of sedatives to ensure amnesia [17, 50]. Of the children in a recent study [50], 24% were classified as over-sedated (BIS score <40) and this group was observed to contain a higher proportion of head injured or encephalopathic individuals. Of the individuals receiving neuromuscular blockers, only 10% had BIS scores less than 40.
The BIS monitor was not designed as a monitor for children or as a monitor of sedation in the intensive care and so its application for this purpose must be viewed with some caution. The subjects, the monitor and the environment have factors that may be potential sources of error when measuring BIS scores. The use of a heterogeneous group of PICU subjects may also obscure the accuracy of the monitor and delay further steps in the refinement of its software. It is unlikely that an algorithm based on a large database of paediatric EEGs will be collected and so continuing validation of the monitor is necessary in order to determine whether the BIS monitor can accurately differentiate depth of sedation in children. The results of our study suggest that, for various groups of children who are sedated in the PICU, the BIS monitor may have a useful role in helping to indicate their level of sedation and correlates well with other validated measures of sedation. The advantage over these other tools is that the measure it produces is continuous. Further studies with the next generation of software could examine whether widespread use of BIS may reduce incidence of withdrawal, time on a ventilator, the total sedation given and/or PICU stay.
References
Playfor SD, Thomas DA, Choonara II (2000) Recall following paediatric intensive care. Paediatr Anaesth 10:703–704
Kollef M, Levy NT, Ahrens TS, Schaiff R, Prentice D, Sherman G (1998) The use of continuous i.v. sedation is associated with prolongation of mechanical ventilation. Chest 114:541–548
Cook D, Walter SD, Cook RJ, Griffith LE, Guyatt GH, Leasa D, Jaeschke RZ, Brun-Buisson C (1998) Incidence of risk factors for ventilator-associated pneumonia in critically ill patients. Ann Intern Med 129:433–440
Cammarano W, Pittet JF, Weitz S, Schlobohm RM, Marks JD (1998) Acute withdrawal syndrome related to the administration of analgesic and sedative medications in adult intensive care units. Crit Care Med 26:676–684
Tobias JD (2000) Tolerance, withdrawal and physical dependency after long-term sedation and analgesia of children in the pediatric intensive care unit. Crit Care Med 28:2122–2132
Anand KJ, Sippell WG, Aynsley-Green A (1987) Pain, anaesthesia and babies. Lancet 2:1210–1212
Kress JP, Pohlman AS, Hall JB (2002) Sedation and analgesia in the intensive care unit. Am J Respir Crit Care Med 166:1024–1028
Playfor SD, Thomas DA, Choonara I (2003) Sedation and neuromuscular blockade in paediatric intensive care: a review of current practice in the UK. Paediatr Anaesth 13:147–151
Bion J, Ledingham IM (1987) Sedation in intensive care—a postal survey. Intensive Care Med 13:215–216
De Jonghe B, Cook D, Appere-de-Vecchi C, Guyatt G, Meade M, Outin H (2000) Using and understanding sedation scoring systems: a systematic review. Intensive Care Med 26:275–285
Ambuel B, Hamlett KW, Marx CM, Blumer JL (1992) Assessing distress in pediatric intensive care environments: the COMFORT scale. J Pediatr Psychol 17:95–109
Marx CM, Smith PG, Lowrie LH, Hamlett KW, Ambuel B, Yamashita TS, Blumer JL (1994) Optimal sedation of mechanically ventilated pediatric critical care patients. Crit Care Med 22:163–170
Denman WT, Swanson EL, Rosow D, Ezbicki K, Connors PD, Rosow CE (2000) Pediatric evaluation of the bispectral index (BIS) monitor and correlation of BIS with end-tidal sevoflurane concentration in infants and children. Anesth Analg 90:872–877
Glass PS, Bloom M, Kearse L, Rosow C, Sebel P, Manberg P (1997) Bispectral analysis measures sedation and memory effects of propofol, midazolam, isoflurane and alfentanil in healthy volunteers. Anesthesiology 86:836–847
Suzuki M, Edmonds HL Jr, Tsueda K, Malkani AL, Roberts CS (1998) Effect of ketamine on bispectral index and levels of sedation. J Clin Monit Comput 14:373
Degoute CS, Macabeo C, Dubreuil C, Duclaux R, Banssillon V (2001) EEG bispectral index and hypnotic component of anaesthesia induced by sevoflurane: comparison between children and adults. Br J Anaesth 86:209–212
Liu J, Singh H, White PF (1997) Electroencephalographic bispectral index correlates with intraoperative recall and depth of propofol-induced sedation. Anesth Analg 84:185–189
Aneja R, Heard AM, Fletcher JE, Heard CM (2003) Sedation monitoring of children by the Bispectral Index in the pediatric intensive care unit. Pediatr Crit Care Med 4:60–64
Bland JM, Altman DG (1995) Calculating correlation coefficients with repeated observations: Part 1—Correlation within subjects. BMJ 310:446
Bland JM, Altman DG (1995) Calculating correlation coefficients with repeated observations: Part 2—Correlation between subjects. BMJ 310:633
Bland JM, Altman DG (1986) Statistical methods for assessing agreement between two methods of clinical measurement. Lancet 1:307–310
Playfor SD, Thomas DA, Choonara I, Collier J, Jarvis A (2001) Parental perceptions of comfort during mechanical ventilation. Paediatr Anaesth 11:99–103
Crain N, Slonim, A, Pollack MM (2002) Assessing sedation in the paediatric intensive care unit by using BIS and the COMFORT scale. Pedatric Crit Care Med 3:11–14
Berkenbosch JW, Fichter CR, Tobias JD (2002) The correlation of the bispectral index monitor with clinical sedation scores during mechanical ventilation in the pediatric intensive care unit. Anesth Analg 94:506–511
Witte H, Putsche P, Eiselt M, Hoffmann K, Arnold M, Jager H, Leistritz L (1998) Multimodal time-variant signal analysis of neonatal EEG burst patterns. Medinfo 9:1250–1254
Eeg-Olofsson O (1980) Longitudinal developmental course of electrical activity of brain. Brain Dev 2:33–44
Davidson AJ, McCann ME, Devavaram P, Auble SA, Sullivan LJ, Gillis JM, Laussen PC (2001) The differences in the bispectral index between infants and children during emergence from anesthesia after circumcision surgery. Anesth Analg 93:326–330
Scher M (1999) Pediatric neurophysiologic evaluation. In: Swaiman KF, Ashwal S (ed) Pediatric neurology. Mosby, St Louis, pp 142–181
McCann ME, Bacsik J, Davidson A, Auble S, Sullivan L, Laussen P (2002) The correlation of bispectral index with end tidal sevoflurane concentration and haemodynamic parameters in preschoolers. Paediatr Anaesth 12:519–525
Bannister CF, Brosius KK, Sigl JC, Meyer BJ, Sebel PS (2001) The effect of bispectral index monitoring on anesthetic use and recovery in children anesthetized with sevoflurane in nitrous oxide. Anesth Analg 92:877–881
Chawathe MS, Hall JE, Mecklenburgh JS, Jones RM (2002) BIS-cautionary interpretation of data in children. Br J Anaesth 89:672P
Simmons LE, Riker RR, Prato BS, Fraser GL (1999) Assessing sedation during intensive care unit mechanical ventilation with the Bispectral Index and the Sedation-Agitation Scale. Crit Care Med 27:1499–1504
Nasraway SS Jr, Wu EC, Kelleher RM, Yasuda CM, Donnelly AM (2002) How reliable is the Bispectral Index in critically ill patients? A prospective, comparative, single-blinded observer study. Crit Care Med 30:1483–1487
Bruhn J, Bouillon TW, Shafer SL (2002) Electromyographic activity falsely elevates the bispectral index. Anesthesiology 92:1485–1487
Riker RR, Fraser GL (2002) Sedation in the intensive care unit: refining the models and defining the questions. Crit Care Med 30:1661–1663
Gallagher JD (1999) Pacer-induced artifact in the bispectral index during cardiac surgery. Anesthesiology 90:636
Guignard B, Chauvin M (2000) Bispectral index increases and decreases are not always signs of inadequate anesthesia. Anesthesiology 92:903
Sakai T, Singh H, Mi WD, Kudo T, Matsuki A (1999) The effect of ketamine on clinical endpoints of hypnosis and EEG variables during propofol infusion. Acta Anaesthesiol Scand 43:212–216
Andrzejowski J, Sleigh JW, Johnson IA, Sikiotis L (2000) The effect of intravenous epinephrine on the bispectral index and sedation. Anaesthesia 55:761–763
Riess ML, Graefe UA, Goeters C, Van AH, Bone HG (2000) Sedation assessment in critically ill patients with bispectral index. Eur J Anaesthesiol 19:18–22
Kim DW, Kil HY, White PF (2001) The effect of noise on the bispectral index during propofol sedation. Anesth Analg 93:1170–1173
Iselin-Chaves I, Flaishon R, Sebel PS, Howell S, Gan TJ, Sigl J, Ginsberg B, Glass PS (1998) The effect of the interaction of propofol and alfentanil on recall, loss of consciousness and the bispectral index. Anesth Analg 87:949–955
Kussman BD, Gruber EM, Zurakowski D, Hansen DD, Sullivan LJ, Laussen PC (2001) Bispectral index monitoring during infant cardiac surgery: relationship of BIS to the stress response and plasma fentanyl levels. Paediatr Anaesth 11:663–669
Theilen HJ, Ragaller M, Tscho U, May SA, Schackert G, Albrecht MD (2000) Electroencephalogram silence ratio for early outcome prognosis in severe head trauma. Crit Care Med 28:3522–3529
Gill M, Green SM, Krauss B (2003) Can the bispectral index monitor quantify altered level of consciousness in emergency department patients? Acad Emerg Med 10:175–179
Gilbert TT, Wagner MR, Halukurike V, Paz HL, Garland A (2001) Use of bispectral electroencephalogram monitoring to assess neurologic status in unsedated, critically ill patients. Crit Care Med 29:1996–2000
Nieuwenhuijs D, Coleman EL, Douglas NJ, Drummond GB, Dahan A (2002) Bispectral index values and spectral edge frequency at different stages of physiologic sleep. Anesth Analg 94:125–129
Sleigh J, Andrzejowski J, Steyn-Ross A, Steyn-Ross M (1999) The bispectral index: a measure of depth of sleep? Anesth Analg 88:659–661
Wagner BK, Zavotsky KE, Sweeney JB, Palmeri BA, Hammond JS (1998) Patient recall of therapeutic paralysis in a surgical critical care unit. Pharmacotherapy 18:358–363
Triltsch AE, Welte M, von Homeyer P, Welte M, von Homeyer P, Grosse J, Genahr A, Moshirzadeh M, Sidiropoulos A, Konertz W, Kox WJ, Spies CD (2002) Bispectral index-guided sedation with dexmedetomidine in intensive care: a prospective, randomized, double blind, placebo-controlled phase II study. Crit Care Med 30:1007–1014
Author information
Authors and Affiliations
Corresponding author
Appendix A
Appendix A
The Comfort scoring system [11]
Alertness | |
Deeply asleep | 1 |
Lightly asleep | 2 |
Drowsy | 3 |
Fully awake and alert | 4 |
Hyper-alert | 5 |
Calmness/agitation | |
Calm | 1 |
Slightly anxious | 2 |
Anxious | 3 |
Very anxious | 4 |
Panicky | 5 |
Respiratory response | |
No coughing and no spontaneous respiration | 1 |
Spontaneous respiration with little or no response to ventilation | 2 |
Occasional cough or resistance to ventilator | 3 |
Actively breathes against ventilator or coughs regularly | 4 |
Fights ventilator: coughing or choking | 5 |
Physical movement | |
No movement | 1 |
Occasional; slight movement | 2 |
Frequent slight movement | 3 |
Vigorous movement limited to extremities | 4 |
Vigorous movement including torso and head | 5 |
Blood pressure | |
Blood pressure below baseline | 1 |
Blood pressure consistently at baseline | 2 |
Infrequent elevations of 15%or more (1–3) | 3 |
Frequent elevations of 15%or more (more than 3) | 4 |
Sustained elevation >15% | 5 |
Heart rate | |
Heart rate below baseline | 1 |
Heart rate consistently at baseline | 2 |
Infrequent elevations of 15% or more above baseline (1–3) | 3 |
Frequent elevations of 15% or more above baseline (more than 3) | 4 |
Sustained elevation >15% | 5 |
Muscle tone | |
Muscles totally relaxed: no muscle tone | 1 |
Reduced muscle tone | 2 |
Normal muscle tone | 3 |
Increased muscle tone and flexion of fingers and toes | 4 |
Extreme muscle rigidity and flexion of fingers and toes | 5 |
Facial tension | |
Facial muscles totally relaxed | 1 |
Facial muscle tone normal; no facial muscle tension evident | 2 |
Tension evident in some facial muscles | 3 |
Tension evident throughout facial muscles | 4 |
Facial muscles contorted and grimacing | 5 |
Total Comfort score | 8–40 |
Rights and permissions
About this article
Cite this article
Courtman, S.P., Wardurgh, A. & Petros, A.J. Comparison of the bispectral index monitor with the Comfort score in assessing level of sedation of critically ill children. Intensive Care Med 29, 2239–2246 (2003). https://doi.org/10.1007/s00134-003-1997-3
Received:
Accepted:
Published:
Issue Date:
DOI: https://doi.org/10.1007/s00134-003-1997-3