Abstract
Objective
To determine whether bovine surfactant given in cases of severe pediatric acute respiratory distress syndrome (ARDS) improves oxygenation.
Design
Single-center study with 19 patients, followed by a multicenter randomized comparison of surfactant with a standardized treatment algorithm. Primary endpoint PaO2/FIO2 at 48 h, secondary endpoints: PaO2/FIO2 at 2, 4, 12, and 24 h, survival, survival without rescue, days on ventilator, subgroups analyzed by analysis of variance to identify patients who might benefit from surfactant
Setting
Multicenter study in 19 reference centers for ARDS.
Patients
Children after the 44th postconceptional week and under 14 years old, admitted for at least 4 h, ventilated for 12–120 h, and without heart failure or chronic lung disease. In the multicenter study 35 patients were recruited; 20 were randomized to the surfactant group and 15 to the nonsurfactant group. Decreasing recruitment of patients led to a preliminary end of this study.
Interventions
Administration of 100 mg/kg bovine surfactant intratracheally under continuous ventilation and PEEP, as soon as the PaO2/FIO2 ratio dropped to less than 100 for 2 h (in the pilot study increments of 50 mg/kg as long as the PaO2/FIO2 did not increase by 20%). A second equivalent dose within 48 h was permitted.
Results
In the pilot study the PaO2/FIO2 increased by a mean of 100 at 48 h (n=19). A higher PaO2/FIO2 ratio was observed in the surfactant group 2 h after the first dose (58 from baseline vs. 9), at 48 h there was a trend towards a higher ratio (38 from baseline vs. 22). The rate of rescue therapy was significantly lower in the surfactant group. Outcome criteria were not affected by a second surfactant dose (n=11). A significant difference in PaO2/FIO2 in favor of surfactant at 48 h was found in the subgroup with an initial PaO2/FIO2 ratio higher than 65 and in patients without pneumonia.
Conclusions
Surfactant therapy in severe ARDS improves oxygenation immediately after administration. This improvement is sustained only in the subgroup of patients without pneumonia and that with an initial PaO2/FIO2 ratio higher than 65
Similar content being viewed by others
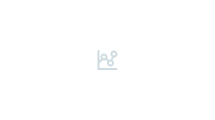
Introduction
Acute respiratory distress syndrome (ARDS) is defined by radiographic diagnosis of diffuse bilateral alveolar infiltrates, the degree of hypoxemia, lung function, and histopathology. It is the final generalized inflammatory response of the lung to catastrophic events of various pulmonary and nonpulmonary origins and occurs in all age groups. The diagnostic criteria have been established by an American-European Consensus Conference [1, 2, 3]. ARDS in children is still associated with a high mortality. Mortality is correlated with the severity of the underlying disease [4, 5]; however, it is still generally accepted that the degree of hypoxemia predicts outcome [5, 6, 7, 8]. Mortality in children with a PaO2/FIO2 ratio lower than 100 in central Europe is still greater than 50% [5]. Another outcome criteria is the aggressiveness of ventilatory support, reflected in the peak inspiratory pressure (PIP), mean airway pressure, and ventilation index [9, 10]. The enforcement of standardized ventilation protocols and the lower incidence of both multiple trauma and sepsis in central Europe have caused a continuous decrease in severe ARDS incidence in children [11, 12, 13].
As the mortality is still high in children with profound hypoxemia and severe underlying conditions such as immunosuppression [4, 5, 8, 13], many other therapies in addition to baro- and volutrauma preventing ventilation strategies have been reported. Nevertheless, randomized controlled studies evaluating new therapeutic strategies for the treatment of severe hypoxemic ARDS as nitric oxide, high-frequency oscillatory ventilation (HFOV), extracorporeal membrane oxygenation (ECMO), and surfactant treatment are lacking in the pediatric age group [14, 15, 16, 17, 18]. Exogenous surfactant improves oxygenation in neonates not only with respiratory distress syndrome but also in other conditions with secondary surfactant deficit such as meconium aspiration syndrome and congenital pneumonia, comparable with ARDS [19]. Oxygenation improved after surfactant administration in several case reports on adult ARDS patients [11, 20] and in a controlled study in moderate pediatric ARDS [10]. A retrospective and prospective survey of pediatric ARDS in all major German pediatric intensive care facilities demonstrated that the mortality and chronic illness after ARDS in patients with PaO2/FIO2 ratios above 150 is very rare, and that most patients with a PaO2/FIO2 ratio around 200 are not even ventilated [5]. For this reason the German-Austrian working group on ARDS performed a controlled, randomized study in severe, hypoxemic ARDS in children, associated with a very high mortality, and no evidence-based treatment options available.
Patients and methods
A single-center pilot study was carried out in 19 pediatric patients with ARDS and PaO2/FIO2 ratio lower than 100, aimed at dose finding and calculating the number of patients required. This pilot study treated patients with ARDS defined by consensus conference criteria and aged between the 44th postconceptional week and 14 years of age. They were included if their PaO2/FIO2 ratio was below 100 for at least 2 h. Ventilation in the pilot study was in accordance with the ventilation algorithm established by the German Working Group on Pediatric ARDS (Fig. 1). A bovine surfactant (Alveofact, Boehringer, Ingelheim, Germany) was administered in 50 mg/kg increments (intratracheal bolus under continuous ventilation and PEEP over maximally 5 min) as long as the PaO2/FIO2 ratio did not increase by 20% or decrease by 10%. The PaO2/FIO2 ratio was determined at 48 h The increase/decrease from baseline was evaluated using the Mann-Whitney U test. No other exclusion criteria were applicable [17, 19]. This pilot study as the following mulitcenter study were approved by the ethics review board of the principal investigators' institution (Medical University of Lübeck).
The subsequent multicenter study was an open, randomized, parallel group comparison performed at 19 German pediatric intensive care units between May 1997 and November 1999. These units were referral centers for children with severe respiratory failure. All children were randomized if they fulfilled the following criteria: ARDS Consensus Conference criteria, lung injury score [21] of at least 2, ventilation between 12 and 120 h, age between 44th postconceptional week and 14 years, admission for at least 4 h, no echocardiographically detectable left heart failure, and a PaO2/FIO2 ratio lower than 100. Written informed consent was obtained from the parents or legal guardians. Patients were excluded if they were under other investigational or experimental therapies: nitric oxide, high frequency ventilation with current disease, liquid ventilation, prostaglandins, steroids for therapy of ARDS, ECMO; chronic lung disease such as bronchopulmonary dysplasia or cystic fibrosis, participation in other clinical studies except treatment protocols and studies for oncological diseases. In addition, patients with severe hypoxemia, i.e., PaO2 lower than 50 after 4 h of treatment in the referral center were excluded.
Thirty-eight patients were recruited over the 18-month period (mean age 3.9 years), but three randomized patients were not included in the study, as their PaO2/FIO2 ratio improved to 100 or higher before the start of treatment. All patients in the participating centers with ARDS in the specified age group who were not included as they did not fulfill all entry criteria were recorded and evaluated as "intended to treat patients" if their PaO2/FIO2 ratio was below 100. All patients in the participating centers were ventilated according to a ventilation algorithm (Fig. 1) [4], blood pressure was kept above the 50th percentile for age, fluid intake between 90% and 100% maintenance [22], and hemoglobin levels above 12 g/dl. As the PaO2/FIO2 ratio was less than 100, patients were randomized either to receive 100 mg/kg bovine surfactant (Alveofact, Boehringer-Ingelheim, Germany), administered under continuous PEEP and ventilation as a bolus over not longer than 5 min to the distal tip of the endotracheal tube (n=20), or be continuously treated based on the ventilation algorithm and concomitant therapy outlined above (n=15). In the surfactant group an additional dose of 100-mg/kg surfactant during the 48-h observation period was allowed if the PaO2 decreased by 20% from the maximum level reached. Diagnoses, age, weight and other demographic characteristics are depicted in Table 2, including the ratio of immunosuppressed patients (after bone marrow transplant or under chemotherapy), PRISM III score, and pneumonia; there were no significant differences between the two groups in regard to these factors.
PaO2, FIO2, paCO2, pH, peak inspiratory pressure, PEEP, ventilatory rate, tidal volume, blood pressure, heart rate, hemoglobin, all medications administered, fluid balance, and the derived variables (PaO2/FIO2, Hallman oxygenation index, ventilatory index, mean airway pressure) were recorded 2, 4, 12, 24, and 48 h after randomization. The patients were followed to day 30 or to discharge/transfer from the referral center to document outcome data: survival, rescue therapy, days on ventilator, days in the ICU, Pediatric Risk of Mortality (PRISM) III score [23], lung injury (Murray score) [21], and days in hospital.
Random allocation was performed centrally by telephone (24 h coverage). The randomization schedule was designed to achieve a 1:1 randomization at each participating center. If the PaO2 decreased to below 50 mmHg for at least 1 h in any patient, all rescue therapies considered appropriate by the principal investigator of the center were allowed (e.g., NO, HFOV, additional surfactant, ECMO, vasodilators). Rotational therapy or prone positioning was obligatory.
The study was conducted as a multicenter, open, randomized parallel comparison. The primary variable was the change from baseline in the PaO2/FIO2 ratio at 48 h after the first administration of surfactant or randomization to the control group. Secondary endpoints were: peak inspiratory pressure, positive end-expiratory pressure, mean airway pressure, in- and expiration time, respiratory rate, FIO2, PaO2, paCO2, SaO2, pH, heart rate, and blood pressure 2, 4, 12, 24, 48, and 120 h after randomization. In addition Murray Score, PRISM III score at baseline, 48 and 120 h, clinical status at 30 days after randomization, days on ventilator, days in intensive care, days on supplemental oxygen, mortality at day 30, ventilator-free days at day 30, and the necessity of rescue therapy as ECMO, HFOV, NO, or rescue surfactant. The primary hypothesis defined was tested by the Mann-Whitney U test at an error level of α≤0.05. A secondary analysis was performed to detect changes from baseline for all other time points up to 48 h by the same test procedure.
For all other variables the same procedures were performed. The number of deaths was compared between groups by means of Fisher's exact test, as were patients who received rescue therapy (each rescue therapy was summarized by frequencies). In a third analysis the combined event death and/or rescue therapy was investigated. We performed multiple regression analyses for changes in the oxygenation index at 2, 4, 12, 24, and 48 h to analyze differences between special subgroups. We used a linear model for repeated measurements. For this post hoc analysis the following subgroups were evaluated: baseline PaO2/FIO2 less than vs. 65 or higher, baseline PRISM III score less than vs. 12 or higher, age under 1 year vs. 2 years or older, girls vs. boys, body weight less than vs. 12 kg or higher, time since FIO2 being higher than 0.5 at randomization shorter than 24 vs. 24 h or longer, time since PIP being higher than 30 cmH2O shorter than 30 h or longer, pneumonia vs. no pneumonia, sepsis vs. no sepsis, and immunosuppression vs. no immunosuppression.
Results
In the pilot study the average increase in PaO2/FIO2 was 54 at 4 h and 103 after 48 h (p<0.01). Seven patients died despite improved oxygenation. The average dose of surfactant given was 94 mg/kg (Table 3). This led to the multicenter study dose of 100 mg/kg.
Regarding the primary variable, change in PaO2/FIO2 over the 48-h observation period, PaO2/FIO2 was significantly higher in the surfactant group 2 h after the first surfactant dose (p<0.003). Even after 48 h the surfactant group patients still showed a greater, albeit not significantly greater, increase in PaO2/FIO2 ratio (Fig. 2). Using the Hallman oxygenation index as oxygenation parameter produced similar results; oxygenation was significantly improved after 2 and 4 h (p<0.00222 and p<0.05). Considering secondary endpoints, mortality and mortality and/or rescue therapy was lower in the surfactant group, however not significantly so at all times during the study period. Mortality in both groups was considerably lower than that in the "intended to treat" patients (64%). Patients in the surfactant group received significantly less rescue therapy than those in the nonsurfactant group (p<0.05).
There was a reduction in mean airway pressures used in the surfactant group after 2 and 24 h (p<0.05, p<0.007; (Fig. 3, Tables 4, 5) – there was no difference between paCO2 or pH in the two groups at any time of the 48-h observation period. Tidal volumes were kept below 10 ml/kg in all cases as specified in Fig. 1. Lung injury and PRISM III scores decreased from 0 to 48 h; however, only the decrease in PRISM III reached the level of significance (p 0.05). No other significant differences in secondary outcome criteria were detected. Eleven patients in the surfactant group received the possible second dose of 100–mg/kg surfactant; no significant increase in PaO2/FIO2 after this second dose was observed at any time. No treatment associated adverse events were observed in the surfactant group; however, the expected risk of intermittent obstruction of the endotracheal tube with a short time deterioration in oxygenation was observed in three patients.
In a post hoc analysis of the PaO2/FIO2 changes from baseline between 2 and 48 h considering various patient characteristics as additional information no significant differences were found regarding Murray Score PRISM III score, age, sex, time with FIO2 longer than 0.5, PIP higher than 30 cmH2O, sepsis vs. no sepsis, or immunosuppression vs. no immunosuppression (Table 6). However, a significant difference in PaO2/FIO2 increase was found between those whose initial ratio was higher than 65 and those whose initial ratio was less and 65 and group 2 patients (p<0.028, Fig. 4); patients with the lower ratio had a 100% mortality in both groups even when rescue therapy was applied. In addition, surfactant patients without pneumonia had significantly better oxygenation after 48 h than nonsurfactant patients with pneumonia (p<0.0017, Fig. 5). In addition, a trend to efficacy of surfactant was seen in patients weighing at least 12 kg (p=0.055).
The study had to be stopped earlier than originally planned due to increasing recruitment difficulty. To demonstrate that the trial was not being stopped at a prejudicial time point the primary comparison between treatments was performed sequentially, post hoc beginning with the first ten evaluable patients. The resulting Z statistics, which are approximately normally distributed, are depicted graphically vs. the number of patients (Fig. 4).
Discussion
In patients with ARDS less endogenous surfactant is produced, and this is inactivated, modified, and not reused, thus causing an absolute and relative surfactant deficiency [20, 24, 25, 26, 23]. As surfactant is a major biological factor for alveolar recruitment, surfactant deficit is a key problem in ARDS, and substitution of surfactant in ARDS could be an important therapeutic tool [19, 23, 26]. As early as 1989 it was hypothesized that surfactant could be of therapeutic value not only in premature infant's respiratory distress syndrome but also in ARDS [24]. In that year the first case report of a successfully surfactant-treated child with severe hypoxemic ARDS was published [27]. Surfactant therapy in preterm infants is now based on sound data (e.g., [28, 29, 30]). In respiratory failure of term infants, in some ways resembling ARDS, a randomized study demonstrated a significant reduction in the need for ECMO [31]. Several uncontrolled studies have been published on adult ARDS [32, 33]; a large controlled study using small doses of aerosolized synthetic surfactant found no advantage of surfactant [33], and a smaller administering a bolus reported improved oxygenation and slightly increased survival in the treatment group [34]. In the pediatric population surfactant has been used in several case reports and in small uncontrolled studies of near fatal ARDS [17, 19, 24, 35]. In mild to moderate ARDS (Hallman oxygenation index less than 10) a randomized study demonstrated improved oxygenation immediately after surfactant administration and most ventilation-associated parameters [36]. In patients with severe hypoxemia we observed a short period of improved oxygenation, as in the study by Willson et al. [36] in patients with less severe hypoxemia.
The originally planned number of patients, however, could not be recruited in the time period scheduled. This might be due to the fact that the overall incidence of ARDS in children decreased dramatically in central Europe during the study period [13, 37]. The study was stopped as the recruitment dropped in this obviously high mortality group. As a sequential analysis of the primary endpoint (normalized Z statistics) demonstrated, the study was not stopped at a point at which there was a significant difference in respect of the primary outcome criteria; this would have been true at an earlier time point.
The overall mortality rate in this study is comparable to that of other studies carried out in severe hypoxemic pediatric ARDS [4, 5, 13]. The trend towards a decreased mortality and need for rescue medication in such a high mortality population might nevertheless be important as no other evidence-based treatment option exists. Surfactant may be only one factor in improving oxygenation in severe ARDS; it enhances the benefits of the "open lung concept," prone positioning, and NO-induced pulmonary vasodilatation [25, 38, 39]. The improvement in oxygenation in the control group, which was managed only on a strictly enforced ventilation algorithm and blood pressure and fluid intake protocol, suggests that standardized treatment alone using conventional methods leads to an overall improvement in patient outcome. This is in line with similar findings by Steinhard et al. [12] who reported improved patient outcome simply by introducing enforced ventilatory management protocols. Surfactant might also be directly involved in improving the balance of pro- and anti-inflammatory mediators in ARDS and therefore be a causative approach [39, 40, 41]. The design of modified surfactant solutions being more prone against inactivation by different proteins in the course of ARDS could enhance and prolong the effect of surfactant on oxygenation [42].
As patients without pneumonia showed a significant improvement and patients weighing more than 12 kg had a benefit from surfactant, it can be speculated that these small infants, in whom pneumonia is the principal cause of ARDS, would require much higher surfactant doses. This would be in accordance with experimental results in which in pneumonia surfactant inactivation was increased, compared with other causes of ARDS [38]. Higher doses of the surfactant preparation used cannot be applied as they would obstruct the major airways. A second dose showed no benefit in our study, either in the data analysis of primary and secondary endpoint criteria or in the post hoc analysis of subgroups. If at all, higher initial doses in selected patients could be considered if a preparation were available with lower volume, lower viscosity, and perhaps more prone to inactivation. Surfactant treatment with a listed price of €200–400/100 mg is still very expensive.
Conclusions
This study reveals the difficulty in obtaining conclusive results from randomized studies in an intensive care setting and patients with a high mortality. Many "intended to treat" patients could not be randomized as they had underlying lung or heart disease, were dying at arrival in the ICU, or had been ventilated for more than 5 days in regional hospitals. An additional difficulty for such a study is the decreasing incidence of ARDS in children. Our study confirmed results of a previous randomized study by Willson et al. [38] reporting improved oxygenation in pediatric patients with mild to moderate ARDS. The improvement in oxygenation was sustained for patients without pneumonia as underlying disease and with a PaO2/FIO2 ratio greater than 65. The latter subgroup does not seem to benefit from any other available rescue tool at this moment. Ventilation variables could be reduced in the surfactant group. The devastating consequences of aggressive ventilation in these children with severe ARDS can probably be ameliorated with surfactant treatment. We conclude that surfactant treatment in severe pediatric ARDS might offer benefits to the patients.
References
Bernard GR, Artigas A, Brigham KL, Carlet J, Falke K, Hudson L, Lamy M, LeGall JR, Morris A, Spragg R, the Consensus Committee (1994) Report of the American-European Consensus Conference on ARDS: definitions, mechanisms, relevant outcomes and clinical trial coordination. Intensive Care Med 20:225–232
Artigas A, Bernard GR, Carlet J, Dreyfuss D, Gattinoni L, Hudson L, Lamy M, Marini JJ, Matthay MA, Pinsky MR, Spragg R, Suter PM, Consensus Committee (1998) The American European consensus conference on ARDS, Part 2, Intensive Care Med 24:378–398
Pfenniger J, Gerber A, Tschäppeler H, Zimmermann A (1982) Adult respiratory distress syndrome in children. J Pediatr 101:352–357
Peters MJ, Tasker RC, Kiff KM, Yates R, Hatch DJ (1998) Acute hypoxemic respiratory failure in children: case mix and the utility of respiratory severity indices. Intensive Care Med 24:699–705
Kühl PG, Appel R, Lasch P, Möller JC, Bindl L (1996) ARDS in pediatrics. Results of a survey in German children's hospitals and recommendations of the "working group pediatric ARDS" on respiratory management. Monatsschr Kinderheilkd 144:1110–1116
Möller JC (1995) Predictive factors of death in pediatric acute respiratory failure. Eur Respir J 8:1436
Möller JC, Raszynski A, Richter A, Tegtmeyer FK (1992) The prognostic value of respiratory indices in pediatric ARDS. Anaesthesist 41:399–402
Bojko T, Notterman DA, Greenwald BM, DeBruin WJ, Magid MS, Godwin T (1995) Acute hypoxemic respiratory failure in children following bone marrow transplantation: an outcome and pathologic study. Crit Care Med 23:755–759
Paret G, Ziv R, Barzilai A, Ben-Abraham R, Vardi A, Manisterki Y, Barzilay Z (1998) Ventilation index and outcome in children with acute respiratory distress syndrome. Pediatr Pulmonol 26:125–128
Willson DF, Jiao JH, Baumann LA, Zaritsky A, Craft H Dockery K, Conrad D, Dalton H (1996) Calf's lung surfactant extract in acute hypoxemic respiratory failure in children. Crit Care Med 24:1316–1322
Amato MB, Barbas CS, Medeiros DM, Magaldi RB, Schettino GP, Lorenzi-Filho G, Kairalla RA, Deheinzelin D, Munoz C, Oliveira R, Takagaki TY, Carvalho CR (1998) Effect of a protective-ventilation strategy on mortality in the acute respiratory distress syndrome. N Engl J Med 338:347–354
Steinhard C, Fackler J, Heulitt M, Nichols D, Green T, Ware J (1996) Randomized trial of protocol driven mechanical ventilation versus ECMO in pediatric patients with ARDS. Pediatrics 98:543
Bindl L, Demirakca S, Fedora M, Goldner M, Huth K, Kohl M, Kühl G, Kachel W, Merz U, Moamad Y, Porz W, Roth J, Scharf J, Varnholt V (2000) ARDS in children: comparison of 1991–1993 vs. 1997–1999. Z Geburthilfe Neonatol 204:S17
Greene JH, Klinger JR (1998) The efficacy of inhaled nitric oxide in the treatment of acute respiratory distress syndrome. An evidence based medicine approach. Crit Care Clin 14:387–409
Abman SH, Griebel JL, Parker DK, Schmidt JM, Swanton D, Kinsella JP (1994) Acute effects of inhaled nitric oxide in children with severe hypoxemic respiratory failure. J Pediatr 124:881–888
Biarent D (1999) New tools in ventilatory support: high frequency ventilation, nitric oxide, tracheal gas insufflation, non-invasive ventilation. Pediatr Pulmonol 18 Suppl:178–181
Möller JC, Schaible TF, Reiss I, Artlich A, Gortner L (1995) Treatment of severe non-neonatal ARDS with surfactant and nitric oxid in a "pre ECMO situation." Int J Artif Organs 18:598–602
Moler FW, Palmisano JM, Custer JR, Meliones JN, Bartlett RH (1994) Alveolar-arterial oxygen gradients beforte extracorporeal life support for severe pediatric respiratory failure: improved outcome for extracorporeal life support managed patients? Crit Care Med 22:620–625
Möller JC, Reiss I, Schaible T, Tegtmeyer FK, Gortner L (1995) Surfactant treatment in children with the acquired respiratory distress syndrome. Monatsschr Kinderheilkd 143:685–690
Parker MM (1996) Surfactant replacement in pediatric respiratory failure: promising therapy for the future? Crit Care Med 24:1281–1282
Murray JF, Matthay MA, Luce JM, Flick MR (1988) An expanded definition of the adult respiratory distress syndrome. Am Rev Respir Dis 138:720–723
Schuster DP (1995) Fluid management in ARDS: keep them dry or does it matter? Intensive Care Med 21:101–103
Pollak MM, Rutimann UE, Getson PR (1988) Pediatric risk of mortality (PRISM) score. Crit Care Med 16:1110–1116
Holm BA, Matalon S (1989) Role of pulmonary surfactant in the development and treatment of adult respiratory distress syndrome. Anesth Analg 69:805–818
Hartog A, Vazquez de Anda GF, Gommers D, Kaisers U, Lachmann B (2000) At surfactant deficiency, application of "the open lung concept" prevents protein leakage and attenuates changes in lung mechanics. Crit Care Med 28:1450–1454
Gommers D, Eijking EP, So KL, van't Veen A, Lachmann B (1998) Broncheoalveolar lavage with a diluted surfactant suspension prior to surfactant instillation improves the effectiveness of surfactant therapy in experimental acute respiratory distress syndrome. Intensive Care Med 24:494–500
Joka T, Obertacke U (1989) New treatment in ARDS: effect of surfactant replacement therapy. Z Herz Thorax Gefasschir 3 [Suppl]:21–24
Gortner L, Wauer RR, Hammer H, Stock GJ, Heitmann F, Reiter HL, Kuhl PG, Möller JC, Friedrich HJ, Reiss I, Hentschel R, Jorch G, Hieronimi G, Kuhls E (1998) Early versus late surfactant treatment in preterm infants of 27–32 weeks gestational age: a multicenter controlled clinical trial. Pediatrics 102:1153–1160
Lopez-Herce J, de Lucas N, Carillo A, Bustinza A, Moral R (1999) Surfactant treatment for acute respiratory distress syndrome. Arch Dis Child 80:248–252
Taeusch HW (2000) Treatment of acute respiratory distress syndrome—the holy grail of surfactant therapy. Biol Neonate 77 [Suppl]:2–8
Lotze A, Mitchell BR, Bulas DI, Zola EM, Shalwitz RA, Gunkel H (1998) Survanta in term infants study group. Multicenter study of surfactant use in the treatment of term infants with severe respiratory failure. J Pediatr 132:40–47
Walmrath D, Günther A, Ghofrani HA, Schermuly R, Schneider T, Grimminger F, Seeger W (1996) Bronchoscopic surfactant administration in patients with severe adult respiratory distress syndrome and sepsis. Am J Respir Crit Care Med 154:57–62
Anzueto A, Baughman R, Guntupalli KK, Weg JG, Wiedemann HP, Raventos AA, Lemaire F, Long W, Zaccardelli DS, Patthishall EN (1996) Aerolsolized surfactants in adults with sepsis induced acute respiratory distress syndrome. N Engl J Med 334:1417–1421
Gregory TJ, Steinberg KP, Spragg R, Gadek JE, Hyers TM, Longmore WJ, Moxley MA, Cai GZ, Hite RD, Smith RM, Hudson LD, Crim C, Newton P, Mitchell BR, Gold AJ (1997) Bovine surfactant therapy for patients with acute respiratory distress syndrome. Am J Respir Crit Care Med 155:1309–1315
Perez-Benavides F, Riff E, Franks C (1995) Adult respiratory distress syndrome and artificial surfactant replacement in the pediatric patient. Pediatr Emerg Care 11:153–156
Willson DF, Zaritsky A, Bauman LA, Dockery K, James RL, Conrad D, Craft H, Novotny WE, Egan EA, Dalton H and Members of the Mid-Atlantic Pediatric Critical Care Network (1999) Instillation of calf lung surfactant extract is beneficial in pediatric acute hypoxemic respiratory failure. Crit Care Med 27:188–195
Möller JC, Vossbeck S, Bindl L and the German ARDS-Surfactant Study Group (2000) Decreasing incidence of severe ARDS in pediatric ICUs in Germany and Austria. Z Geburtshilfe Neonatol 204:S18
Zhao DH, Sun B, Wu ZH, Lindwall R, Robertson B (2000) Mitigation of endotoxin induced acute lung injury in ventilated rabbits by surfactant and inhaled nitric oxide. Intensive Care Med 26:229–238
Ulrich R, Lorber C, Röder G, Urak G, Faryniak B, Sladen RN, Germann P (1999) Controlled airway pressure therapy, nitric oxide inhalation, prone position and ECMO as components of an integrated approach to ARDS. Anesthesiology 91:1577–1586
Dupont H, Mentec H, Cheval C, Moine P, Fierobe L, Timsit JF (2000) Short term effect of inhaled nitric oxide and prone positioning on gas exchange in patients with severe acute respiratory distress syndrome. Crit Care Med 28:304–308
Schultz C, Rott C, Richter N, Bucsky P (1999) Intracytoplasmatic detection of cytokines in neonatal lymphocytes and monocytes by flow cytometry. Blood 93:3566–3567
Walker TA (1999) The acute respiratory distress syndrome in children: recent UMMC experience. J Mississippi Med Assoc Nov:371–375
Acknowledgements
This study was funded by Boehringer Ingelheim Pharma KG, Ingelheim, Germany. The ARDS Surfactant Study Group includes the following: Departments of Pediatrics/PICU: University of Aachen: H. Hörnchen MD, PhD, U. Merz MD PhD; Humboldt University of Berlin, PICU: V. Varnholt MD; University of Bonn: L. Bindl MD, S. Buderus MD; University of Erlangen: J. Scharf MD; University of Essen: C. Roll MD, L. Hanssler MD, PhD, University of Freiburg: R. Hentschel MD, T. Höhn MD; University of Giessen: S. Demirakca MD, I. Reiss MD, L. Gortner MD, PhD; University of Göttingen: H. Schiffmann MD, E. Herting MD, PhD; University of Graz: S. Rödl MD, G. Zobel MD, PhD; Medical College of Hanover: T. Paul MD, PhD, M. Sasse MD; University of Innsbruck: B. Simma MD, PhD, R. Trawöger; University of Cologne: B. Roth MD, PhD, A. Vierzig MD; Central Hospital Cologne: P. Groneck MD, PhD; Medical University of Lübeck: J.C. Möller, MD, PhD, FCCM, M. Kohl MD; University of Mainz: R.G. Huth MD, C.F. Wippermann MD; Central Hospital Mannheim/University of Heidelberg: P. Lasch MD, T. Schaible MD; University of Ulm: S. Voßbeck MD, F. Pohlandt MD, PhD; University of Würzburg: L. Schrod MD, PhD, G. Hofmann MD, Medical Sciendes Department, Boehringer Ingelheim Pharma KG: P. Schmidt PhD, H Schumacher PhD, H. von Seefeld PhD.
Author information
Authors and Affiliations
Consortia
Corresponding author
Rights and permissions
About this article
Cite this article
Möller, J.C., Schaible, T., Roll, C. et al. Treatment with bovine surfactant in severe acute respiratory distress syndrome in children: a randomized multicenter study. Intensive Care Med 29, 437–446 (2003). https://doi.org/10.1007/s00134-003-1650-1
Received:
Accepted:
Published:
Issue Date:
DOI: https://doi.org/10.1007/s00134-003-1650-1