Abstract
On the evening of December 22, 2018, the coasts of the Sunda Strait, Indonesia, were hit by a tsunami generated by the collapse of a part of the Anak Krakatau volcano. Hundreds of people were killed, thousands were injured and displaced. This paper presents a preliminary modeling of the volcano flank collapse and the tsunami generated based on the results of a 2D depth-averaged coupled model involving a granular rheology and a Coulomb friction for the slide description and dispersive effects for the water flow part. With a reconstructed total volume (subaerial and submarine) of the landslide of 150 million \(\hbox {m}^{3}\) inferred from pre and post-collapse satellite and aerial images, the comparison of the simulated water waves with the observations (tide gauges located all around the strait, photographs and field surveys) is satisfactory. Due to the lack of information for the submarine part of the landslide, the reconstructed submarine slope is assumed to be approximately constant. A significant time delay on the results and particularly in the Bandar Lampung Bay could be attributed to imprecisions of bathymetric data. The sensitivity to the basal friction and to dispersive effects is analyzed through numerical tests. Results show that the influence of the basal friction angle on the simulated wave heights decreases with distance and that a value of \(2^{\circ }\) gives consistent results with the observations. The dispersive effects are assessed by comparing water waves simulated by a shallow water model and a Boussinesq model. Simulations with frequency dispersion produce longer wave periods and smaller wave amplitudes in the Sunda Strait and particularly in deep waters.













Similar content being viewed by others
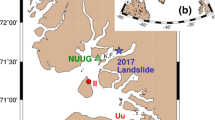
Notes
https://bnpb.go.id/volume-tubuh-gunung-anak-krakatau-berkurang-jumlah-korban-tsunami-bertambah, last accessed 10 september 2019.
References
Abadie, S., Harris, J., Grilli, S., & Fabre, R. (2012). Numerical modeling of tsunami waves generated by the flank collapse of the Cumbre Vieja Volcano (La Palma, Canary Islands): Tsunami source and near fiedl effects. Journal of Geophysical Research, 117, 5.
Assier-Rzadkiewicz, S., Heinrich, P., Sabatier, P., Savoye, B., & Bourillet, J. (2000). Numerical modelling of a landslide-generated tsunami: The 1979 Nice event. Pure and Applied Geophysics, 157(10), 1707–1727.
Bondevik, S., Løvholt, F., Harbitz, C., Mangerud, J., Dawson, A., & Svendsen, J. (2005). The Storegga slide tsunami—comparing field observations with numerical simulations. Marine and Petroleum Geology, 22, 195–208.
Camus, G., Gourgaurd, A., & Vincent, P. (1987). Petrologic evolution of Krakatau (Indonesia): Implications for a future activity. Journal of Volcanology and Geothermal Research, 33, 299–316.
Choi, B., Pelinovsky, E., Kim, K., & Lee, J. (2003). Simulation of the trans-oceanic tsunami propagation due to the 1883 Krakatau volcanic eruption, Copernicus Publications on behalf of the European Geosciences Union. Natural Hazards and Earth System Science, 3(5), 321–332.
Cooke, R. (1981). Eruptive history of the volcano at Ritter Island. Geological Survey of Papua New Guinea Memoir, 10, 115–123.
Deplus, C., Bonvalot, S., Dahrin, D., Diament, M., Harjono, H., & Dubois, J. (1995). Inner structure of the Krakatoa volcanic complex (Indonesia) from gravity and bathymetry data. Journal of Volcanology and Geothermal Research, 64, 23–51.
Fine, I. V., Rabinovich, A. B., Thomson, R. E., & Kulikov, E. A. (2003). Numerical modeling of tsunami generation by submarine and subaerial landslides (pp. 69–88). Dordrecht: Springer.
Franz, M., Jaboyedoff, M., Locat, J., & Podladchikov, Y. (2015). Testing a landslide-generated tsunami model. The case of the Nicolet landslide (Québec, Canada), In Conference GEOQuébec 2015, Québec City, QC, Canada.
Fritz, H., Mohammed, F., & Yoo, J. (2009). Lituya Bay landslide impact generated mega-tsunami 50th anniversary. Pure and Applied Geophysics, 166, 153–175.
Giachetti, T., Paris, R., Kelfoun, K., & Ontowirjo, B. (2012). Tsunami hazard related to a flank collapse of Anak Krakatau volcano, Sunda Strait, Indonesia. Geological Society London Special Publication, 361, 79–89.
Giachetti, T., Paris, R., Kelfoun, K., & Pérez-Torrado, F. (2011). Numerical modelling of the tsunami triggered by the Güìmar debris avalanche, Tenerife (Canary Islands): Comparison with field-based data. Marine Geology, 284, 189–202.
Glimsdal, S., L’Heureux, J.-S., Harbitz, C., & Løvholt, F. (2016). The 29th January 2014 submarine landslide at Statland, Norway-landslide dynamics, tsunami generation, and run-up. Landslides, 13(6), 1435–1444.
Glimsdal, S., Pedersen, G., Harbitz, C., & Løvholt, F. (2013). Dispersion of tsunamis: Doest it really matter? Natural Hazards and Earth System Sciences, 13, 1507–1526.
Gouhier, M., & Paris, R. (2019). SO2 and tephra emissions during the December 22, 2018 Anak Krakatau flank-collapse eruption. Volcanica, 2(2), 91–103.
Grilli, S., O’Reilley, C., Harris, J., Tajalli Bakhsh, T., Tehranirad, B., Banihashemi, S., et al. (2015). Modeling of SMF tsunami hazard along the upper US East Coast: Detailed impact around Ocean City, MD. Natural Hazards, 76, 705–746.
Grilli, S., Tappin, D., Carey, S., Watt, S., Ward, S., Grilli, A., et al. (2019). Modelling of the tsunami from the December 22, 2018 lateral collapse of Anak Krakatau volcano in the Sunda Straits, Indonesia. Scientific Reports, 9, 11946.
Grilli, S., & Watts, P. (2005). Tsunami generation by submarine mass failure. I: Modeling, experimental validation, and sensitivity analyses. Journal of Waterway, Port, Coastal and Ocean Engineering, 131(6), 283–297.
Haflidason, H., Sejrup, H., Nygard, A., Meinert, J., Bryn, P., Lien, R., et al. (2004). The Storegga slide: Architecture, geometry and slide development. Marine Geology, 213(1–4), 201–234.
Harbitz, C. (1992). Model simulations of tsunamis generated by the Storegga slides. Marine Geology, 105(1–4), 1–21.
Harbitz, C., Glimsdal, S., Løvholt, F., Kveldsvik, V., Pedersen, G., & Jensen, A. (2014). Rockslide tsunamis in complex fjords: From an unstable rock slope at Åkerneset to tsunami risk in western Norway. Coastal Engineering, 88, 101–122.
Harbitz, C., Pedersen, G., & Gjevik, B. (1993). Numerical simulations of large water waves due to landslides. Journal of Hydraulic Engineering, 119(12), 1325–1342.
Harjono, H., Diament, M., Dubois, J., & Larue, M. (1991). Seismicity of the Sunda strait: Evidence for crustal extension and volcanological implications. Tectonics, 10, 17–30.
Heidarzadeh, M., Ishibe, T., Sandanbata, O., Muhari, A., & Wijanarto, A. (2020). Numerical modeling of the subaerial landslide source of the 22 December 2018 Anak Krakatoa volcanic tsunami Indonesia. Ocean Engineering, 20, 195.
Heidarzadeh, M., Krastel, S., & Yalçiner, A. (2014). The state-of-the-art numerical tools for modeling landslide tsunamis: A short review. In S. Krastel, J.-H. Behrmann, D. Völker, M. Stipp, C. Berndt, R. Urgeles, J. Chaytor, K. Huhn, M. Strasser, & C. Harbitz (Eds.), Submarine mass movements and their consequences: 6th international symposium (pp. 483–495). Cham: Springer.
Heinrich, P., Boudon, G., Komorowski, J. C., Sparks, R. S. J., Herd, R., & Voight, B. (2001b). Numerical simulation of the December 1997 Debris Avalanche in Montserrat, Lesser Antilles. Geophysical Research Letters, 28(13), 2529–2532.
Heinrich, P., & Piatanesi, A. (2000). Near-field modeling of the July 17, 1998 tsunami in Papua New Guinea. Geophysical Research Letters, 27(19), 3037–3040.
Heinrich, P., Piatanesi, A., & Hébert, H. (2001a). Numerical modelling of tsunami generation and propagation from submarine slumps: The 1998 Papua New Guinea event. Geophysical Journal International, 145(1), 97–111.
Horrillo, J., Wood, A., Kim, G.-B., & Parambath, A. (2013). A simplified 3-D Navier–Stokes numerical model for landslide-tsunami: Application to the Gulf of Mexico. Journal of Geophysical Research: Oceans, 118(12), 6934–6950.
Huang, B., Yin, Y., Wang, S., Tan, J., & Liu, G. (2017). Analysis of the Tangjiaxi landslide-generated waves in the Zhexi Reservoir, china, by a granular flow coupling model. Natural Hazards and Earth System Sciences, 17, 657–670.
Hébert, H., Piatanesi, A., Heinrich, P., & Schindelé, F. (2002). Numerical modeling of the September 13, 1999 landslide and tsunami on Fatu Hiva Island (French Polynesia). Geophysical Research Letters, 29(10), 10–13.
Jiang, L., & LeBlond, P. (1992). The coupling of a submarine slide and the surface waves which it generates. Journal of Geophysical Research: Oceans, 97(C8), 12731–12744.
Johnson, R. (1987). Large-scale volcanic cone collapse; the 1888 slope failure of Ritter volcano. Bulletin of Volcanology, 49, 669–679.
Kelfoun, K., Giachetti, T., & Labazuy, P. (2010). Landslide-generated tsunamis at Réunion Island. Journal of Geophysical Research: Earth Surface, 115, F4.
Kirby, J., Shi, F., Nicolsky, D., & Misra, S. (2016). The 27 April 1975 Kitimat, British Columbia, submarine landslide tsunami: A comparison of modeling approaches. Landslides, 13, 1421–1434.
Labbé, M., Donnadieu, C., Daubord, C., & Hébert, H. (2012). Refined numerical modeling of the 1979 tsunami in Nice (French Riviera): Comparison with coastal data. Journal of Geophysical Research: Earth Surface, 117, F1.
Le Friant, A., Heinrich, P., Deplus, C., & Boudon, G. (2003). Numerical simulation of the last flank-collapse event of Montagne Pelée, Martinique, Lesser Antilles. Geophysical Research Letters, 30, 2.
L’Heureux, J.-S., Glimsdal, S., Longva, O., Hansen, L., & Harbitz, C. (2011). The 1888 shoreline landslide and tsunami in Trondheimsfjorden, central Norway. Marine Geophysical Research, 32(1), 313–329.
Liu, P.-F., Woo, S.-B., & Cho, Y.-S. (1998). Computer programs for tsunami propagation and inundation (p. 25). Cornell: Cornell University.
Løvholt, F., Pedersen, G., & Gisler, G. (2008). Oceanic propagation of a potential tsunami from the La Palma Island. Journal of Geophysical Research: Oceans, 113(9), 1–21.
Ma, G., Kirby, J., Hsu, T.-J., & Shi, F. (2015). A two-layer granular landslide model for tsunami wave generation: Theory and computation. Ocean Modelling, 93, 40–55.
Ma, G., Shi, F., & Kirby, J. (2012). Shock-capturing non-hydrostatic model for fully dispersive surface wave processes. Ocean Modelling, 43–44, 22–35.
Mangeney, A., Heinrich, P., & Roche, R. (2000). Analytical solution for testing debris avalanche numerical models. Pure and Applied Geophysics, 157, 1081–1096.
Masson, D., Harbitz, C., Wynn, R., Pedersen, G., & Løvholt, F. (2006). Submarine landslides: Processes, triggers and hazard prediction. Philosophical Transactions of the Royal Society A: Mathematical, Physical and Engineering Sciences, 364(1845), 2009–2039.
Miller, D. J. (1960). Giant waves in Lituya Bay, Alaska. US Geological Survey Professional Paper, 354-C.
Muhari, A., Heidarzadeh, M., Susmoro, H., Nugroho, H., Kriswati, E., Supartoyo, W. A., et al. (2019). The December 2018 Anak Krakatau Volcano tsunami as inferred from post-tsunami field surveys and spectral analysis. Pure and Applied Geophysics, 21, 1–5.
Nishimura, S., Nishida, J., Yokoyama, T., & Hehuwat, F. (1986). Neotectonics of the Straits of Sunda, Indonesia. Journal of Southeast Asian Earth Science, 1, 81–91.
Nomanbhoy, N., & Satake, K. (1995). Generation mechanism of tsunamis from the 1883 Krakatau eruption. Geophysical Reasearch Letters, 22(4), 509–512.
Okal, E. A., & Synolakis, C. E. (2003). A theoretical comparison of tsunamis from dislocations and landslides. Pure and Applied Geophysics, 160(10–11), 2177–2188.
Paris, A., Okal, E., Guérin, C., Heinrich, P., Schindelé, F., & Hébert, H. (2019). Numerical modeling of the June 17, 2017 landslide and tsunami events in Karrat Fjord, west Greenland. Pure and Applied Geophysics, 176(7), 3035–3057.
Pawlowicz, R., Beardsley, B., & Lentz, S. (2002). Classical tidal harmonic analysis including error estimates in MATLAB using T\_TIDE. Computers and Geosciences, 28, 929–937.
Pedersen, G. & Løvholt, F. (2008). Documentation of a global Boussinesq solver. Preprint series in Applied Mathematics 1. http://urn.nb.no/URN:NBN:no-27775.
Poupardin, A., Heinrich, P., Frère, A., Imbert, D., Hébert, H., & Flouzat, M. (2017). The 1979 submarine landslide-generated tsunami in Mururoa, French Polynesia. Pure and Applied Geophysics, 174, 3293–3311.
Rodriguez, M., Chamot-Rooke, N., Hébert, H., Fournier, M., & Huchon, P. (2013). Owen Ridge deep-water submarine landslides: Implications for tsunami hazard along the Oman coast. Natural Hazards and Earth System Science, 13, 417–424.
Satake, K., & Kato, Y. (2001). The 1741 Oshima–Oshima eruption: Extent and volume of submarine debris avalanche. Geophysical Research Letters, 28, 427–430.
Satake, K., Smith, J., & Shinozaki, K. (2002). ‘Three-dimensional reconstruction and tsunami model of the Nuuanu and Wailau giant landslides (pp. 333–346). Hawaii: American Geophysical Union Geophysical Monograph Series.
Savage, S. B., & Hutter, K. (1989). The motion of a finite mass of granular material down a rough incline. Journal of Fluid Mechanics, 199, 177–215.
Savage, S. B., & Hutter, K. (1991). The dynamics of avalanches of granular materials from initiation to runout. Part I: Analysis. Acta Mechanica, 86(1), 201–223.
Shi, F., Kirby, J., Harris, J., Geiman, J., & Grilli, S. (2012). A high-order adaptive time-stepping TVD solver for Boussinesq modeling of breaking waves and coastal inundation. Ocean Modelling, 43–44, 36–51.
Sigurdsson, H., Carey, S., Mandeville, C., & Bronto, S. (1991). Pyroclastic flows of the 1883 Krakatau eruption. Eos Transactions of the American Geophysical Union, 72(36), 377–392.
Simkin, T., & Fiske, R. (1983). Krakatau 1883: The volcanic eruption and its effects. Washington, D.C.: Smithsonian Institution Press.
Skvortsov, A., & Bornhold, B. (2007). Numerical simulation of the landslide-generated tsunami in Kitimat Arm, British Columbia, Canada, 27 April 1975. Journal of Geophysical Research: Earth Surface, 112, 2.
Stehn, C. (1929). The geology and volcanism of the Krakatau Group. In Proceedings of the Fourth Pacific science congress, pp. 1–55.
Sudradjat, A. (1982). The morphological development of Anak Krakatau Volcano, Sunda Strait. Geologi Indonesia, 9, 1–11.
Susilohadi, S., Gaedicke, C., & Djajadihardja, D. (2009). Structures and sedimentary deposition in the Sunda Strait, Indonesia. Tectonophysics, 467, 55–71.
Synolakis, C. E., Bardet, J.-P., Borrero, J. C., Davies, H. L., Okal, E. A., Silver, E. A., Sweet, S., & Tappin, D. R. (2002). The slump origin of the 1998 Papua New Guinea tsunami. In Proceedings of the Royal Society of London, series A: Mathematical, physical and engineering sciences, Vol. 458, The Royal Society, pp. 763–789.
Takabatake, T., Shibayama, T., Esteban, M., Achiari, H., Nurisman, N., Gelfi, M., et al. (2019). Field survey and evacuation behaviour during the 2018 Sunda Strait tsunami. Coastal Engineering Journal, 20, 1–21.
Tappin, D., Grilli, S., Harris, J., Geller, R., Masterlark, T., Kirby, J., et al. (2014). Did a submarine landslide contribute to the 2011 Tohoku tsunami? Marine Geology, 357, 344–361.
Tappin, D., Watts, P., & Grilli, S. (2008). The Papua New Guinea tsunami of 17 July 1998: Anatomy of a catastrophic event. Natural Hazards and Earth System Sciences, 8, 243–266.
TDMRC (2019) The latest update from post-Sunda Strait tsunami survey. http://tdmrc.unsyiah.ac.id/the-latest-update-from-post-sunda-strait-tsunami-survey/.
Walter, T., Haghighi, M., Schneider, F., Coppola, D., Motagh, M., Saul, J., Babeyko, A., Dahm, T., Troll, V., Tilmann, F., Heimann, S., Valade, S., Triyono, R., Khomarudin, R., Kartadinata, N., Laiolo, M., Massimetti, F., & Gaebler, P. (2019). Precursors and processes culminating in the Anak Krakatau December 2018 sector collapse and tsunami. Nature Communications(in revision).
Wang, X., & Liu, P.-F. (2006). An analysis of 2004 Sumatra earthquake fault plane mechanisms and Indian Ocean tsunami. Journal of Hydraulic Research, 44, 147–154.
Yavari-Ramshe, S., & Ataie-Ashtiani, B. (2016). Numerical modeling of subaerial and submarine landslide-generated tsunami waves-recent advances and future challenges. Landslides, 13, 1325–1368.
Acknowledgements
We thank Abdul Muhari, from the Directorate of Sustainable Utilization of Coastal Zone and Small Islands, Ministry of Marine Affairs and Fisheries, Indonesia, for providing us with the tide gauges data and the KKP field survey data, and Gegar Prasetya, for providing us the BMKG field survey data.
Author information
Authors and Affiliations
Corresponding author
Additional information
Publisher's Note
Springer Nature remains neutral with regard to jurisdictional claims in published maps and institutional affiliations.
Rights and permissions
About this article
Cite this article
Paris, A., Heinrich, P., Paris, R. et al. The December 22, 2018 Anak Krakatau, Indonesia, Landslide and Tsunami: Preliminary Modeling Results. Pure Appl. Geophys. 177, 571–590 (2020). https://doi.org/10.1007/s00024-019-02394-y
Received:
Revised:
Accepted:
Published:
Issue Date:
DOI: https://doi.org/10.1007/s00024-019-02394-y