Abstract
Background
A long-standing goal in genetic therapy for cancer is a systemic gene delivery system that selectively targets tumor cells, including metastases. Here we describe a novel cationic immunolipoplex system that shows high in vivo gene transfer efficiency and antitumor efficacy when used for systemic p53 gene therapy of cancer.
Materials and Methods
A cationic immunolipoplex incorporating a biosynthetically lipid-tagged, anti-transferrin receptor single-chain antibody (TfRscFv), was designed to target tumor cells both in vitro and in vivo. A human breast cancer metastasis model was employed to evaluate the in vivo efficacy of systemically administered, TfRscFv-immunolipoplex-mediated, p53 gene therapy in combination with docetaxel.
Results
The TfRscFv-targeting cationic immunolipoplex had a size of 60–100 nm, showed enhanced tumor cell binding, and improved targeted gene delivery and transfection efficiencies, both in vitro and in vivo. The p53 tumor suppressor gene was not only systemically delivered by the immunolipoplex to human tumor xenografts in nude mice but also functionally expressed. In the nude mouse breast cancer metastasis model, the combination of the p53 gene delivered by the systemic administration of the TfRscFv-immunolipoplex and docetaxel resulted in significantly improved efficacy with prolonged survival.
Conclusions
This is the first report using scFv-targeting immunolipoplexes for systemic gene therapy. The TfRscFv has a number of advantages over the transferrin (Tf) molecule itself: (1) scFv has a much smaller size than Tf producing a smaller immunolipoplex giving better penetration into solid tumors; (2) unlike Tf, the scFv is a recombinant protein, not a blood product; (3) large scale production and strict quality control of the recombinant scFv, as well as scFv-immunolipoplex, are feasible. The sensitization of tumors to chemotherapy by this tumor-targeted and efficient p53 gene delivery method could lower the effective dose of the drug, correspondingly lessening the severe side effects, while decreasing the possibility of recurrence. Moreover, this approach is applicable to both primary and recurrent tumors, and more significantly, metastatic disease. The TfRscFv-targeting of cationic immunolipoplexes is a promising method of tumor targeted gene delivery that can be used for systemic gene therapy of cancer with the potential to critically impact the clinical management of cancer.









Similar content being viewed by others
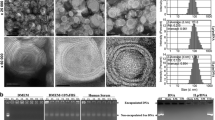
References
Meng RD, EL-Deiry WS. (1999) Tumor suppressor genes as targets for cancer gene therapy. In: Lattime EC and Gerson SL, eds., Gene Therapy of Cancer, Academic Press, San Diego, CA. pp. 3–20.
Zhang W, Fujiwara T, Grimm EA, Roth JA. (1997) Advances in Cancer Gene Therapy. Adv. Pharmacol. 32: 289–333.
Chen QR, Mixson JA. (1998) Systemic gene therapy with p53 inhibits breast cancer: recent advances and therapeutic implications. Front. Biosci. 3: D997–D1004.
Chang EH, XuL, Pirollo KF. (2000) Targeted p53 Gene Therapy Mediated Radiosensitization and Chemosensitization. In: Gutking JS ed. Signaling Networks and Cell Cycle Control: The Molecular Basis of Cancer and Other Diseases. The Humana Press Inc., Totowa, NJ. pp. 519–536.
Huang L, Viroonchatapan E. (1999) Introduction. In: Non-viral Vectors for Gene Therapy. Huang L, Hung MC and Wagner E. eds., Academic Press, San Diego, CA. pp. 3–22.
Ledley FD. (1995) Nonviral gene therapy: the promise of genes as pharmaceutical products. Hum. Gene Ther. 6: 1129–1144.
Felgner PL, Tsai YJ, Sukhu L, et al. (1995) Improved cationic lipid formulations for in vivo gene therapy. Ann. NY Acad. Sci. 772: 126–139.
Felgner PL. (1999) Progress in gene delivery research and development. In: Non-viral Vectors for Gene Therapy. Huang L, Hung MC and Wagner E. eds., Academic Press, San Diego, CA. pp. 26–38.
Christiano RJ, Curiel DT. (1996) Strategies to accomplish gene therapy via the receptor-mediated endocytosis pathways. Cancer Gene Ther. 3: 457–497.
Keer HN, Kozlowski JM, Tsai MC. (1990) Elevated transferrin receptor content in human prostate cancer cell lines assessed in vitro and and in vivo. J. Urol. 143: 381–385.
Rossi MC, Zetter BR. (1992) Selective stimulation of prostate carcinoma cell proliferation by transferrin. Proc. Natl. Acad. Sci. USA 89: 6197–6201.
Elliott RL, Elliott MC, Wang F, Head JF. (1993) Breast carcinoma and the role of iron metabolism. a cytochemical, tissue culture, and ultrastructural study. Ann. NY Acad. Sci. 698: 159–166.
Miyamoto T, Tanaka N, Eishi Y, Amagasa, T. (1994) Transferrin receptor in oral tumors. Int. J. Oral Maxillofac. Surg. 23: 430–433.
Thorstensen K, Romslo I. (1993) The Transferrin receptor: its diagnostic value and its potential as therapeutic target. Scand. J. Clin. Lab. Invest. Suppl. 215: 113–120.
Xu L, Pirollo KF, Chang EH. (1997) Transferrin-liposome-mediated p53 sensitization of squamous cell carcinoma of the head and neck radiationin vitro. Hum. Gene Ther. 8: 467–475.
Xu L, Pirollo KF, Tang W, Rait A, Chang EH. (1999) Transferrin-liposome-mediated systemic p53 gene therapy in combination with radiation results in regression of human head and neck cancer xenografts. Hum. Gene Ther. 10: 2941–2952.
Keinanen K, Laukkanen ML. (1994) Biosynthetic lipid-tagging of antibodies. FEBS Letters 346: 123–126.
de Kruif J, Storm G, van Bloois L, Logtenberg T. (1996) Biosynthetically lipid-modified human scFv fragments from phage display libraries as targeting molecules for immunoliposomes. FEBS Letters 399: 232–236.
Haynes BF, Hemler M, Cotner T, et al. (1981) Characterization of a monoclonal antibody (5E9) that defines a human cell surface antigen of cell activation. J. Immunol. 127: 347–351.
Xu L, Pirollo KF, Rait A, Murray AL, Chang EH. (1999) Systemic p53 Gene Therapy In Combination with Radiation Results in Human Tumor Regression. Tumor Targeting 4: 92–104.
Chen L, Agrawal S, Zhou W, Zhang R, Chen J. (1998) Synergistic activation of p53 by inhibition of MDM2 expression and DNA damage. Proc. Natl. Acad. Sci. USA 95: 195–200.
Leonessa F, Green D, Licht T, et al. (1996) MDA435/LCC6 and MDA435/LCC6MDR1: ascites models of human breast cancer. Br. J. Cancer. 73: 154–161.
Laukkanen ML, Teeri TT, Keinanen K. (1993) Lipid-tagged antibodies: bacterial expression and characterization of a lipoprotein-single-chain antibody fusion protein. Protein. Eng. 6: 449–454.
Laukkanen ML, Alfthan K, Keinanen K. (1994) Functional immunoliposomes harboring a biosynthetically lipid-tagged single-chain antibody. Biochemistry 33: 11664–11670.
New RRC. (1989) Preparation of liposomes. In: Liposomes-a practical approach. New RRC, Ed., IRL Press, Oxford, UK. pp. 33–104.
Pirollo KF, Hao Z, Rait A, Jang YJ, Fee WE Jr, Ryan P, Chiang Y, Chang EH. (1997) p53 mediated sensitization of squamous cell carcinoma of the head and neck to radiotherapy. Oncogene 14: 1735–1746.
Wagner E. (1999) Ligand-polycation conjugates for receptortargeted gene transfer. In: Non-viral Vectors for Gene Therapy. Huang L, Hung MC and Wagner E. eds., Academic Press, San Diego, CA. pp. 208–227.
Pirollo KF, Xu L, Chang EH. (2000) Non-viral gene delivery for p53. Curr. Opin. Mol. Ther. 2: 168–175.
Allen TM, Hansen CB, Zalipsky S. (1995) Antibody-targeted stealth liposomes. In: Stealth Liposomes, Lasic D., and Martin F. (Eds), CRC Press, Boca Raton, Florida. pp. 233–244.
Hansen CB, Kao GY, Moase EH, Zalipsky S, Allen TM. (1995) Attachment of antibodies to sterically stabilized liposomes: evaluation, comparison and optimization of coupling procedures. Biochim. Biophys. Acta. 1239: 133–144.
Mastrobattista E, Koning GA, Storm G. (1999) Immunoliposomes for the targeted delivery of antitumor drugs. Adv. Drug Deliv. Rev. 40: 103–127.
Park JW, Hong K, Carter P, et al. (1995) Development of anti-p185HER2 immunoliposomes for cancer therapy. Proc. Natl. Acad. Sci. USA 92: 1327–1331.
Suzuki S, Inoue K, Hongoh A, Hashimoto Y, Yamazoe Y. (1997) Modulation of doxorubicin resistance in a doxorubicin-resistant human leukaemia cell by an immunoliposome targeting transferring receptor. Br. J. Cancer 76: 83–89.
Huwyler J, Yang J, Pardridge WM. (1997) Receptor mediated delivery of daunomycin using immunoliposomes: pharmacokinetics and tissue distribution in the rat. J. Pharmacol. Exp. Ther. 282: 1541–1546.
Weinberg ED. (1992) Roles of iron in neoplasia. Promotion, prevention, and therapy. Biol. Trace Elements Res. 34: 123–140.
Pelegrin M, Marin M, Noel D, Piechaczyk M. (1998) Genetically engineered antibodies in gene transfer and gene therapy. Hum. Gene Ther. 9: 2165–2175.
Huang SK, Martin FJ, Friend DS, Papahadjopoulos D. (1995) Mechanism of stealth liposome accumulation in some pathological tissues. In: Stealth Liposomes, Lasic D., and Martin F. eds, CRC Press, Boca Raton, Florida. pp. 119–125.
Feng D, Nagy JA, Hipp J, Pyne K, Dvorak HF, Dvorak AM. (1997) Reinterpretation of endothelial cell gaps induced by vasoactive mediators in guinea-pig, mouse and rat: many are transcellular pores. J. Physiol. 504: 747–761.
Ozbun MA, Butel JS. (1995) Tumor suppressor p53 mutations and breast cancer: a critical analysis. Adv Cancer Res. 66: 71–141.
Allred DC, Elledge R, Clark GM, Fuqua SA. (1994) The p53 tumor-suppressor gene in human breast cancer. Cancer Treat. Res. 71: 63–77.
Kerr JF, Winterford CM & Harmon BV. (1994) Apoptosis: Its significance in cancer and cancer therapy. Cancer 7: 2013–2026.
Yeung TK, Germond C, Chen X, Wang Z. (1999) The mode of action of taxol: apoptosis at low concentration and necrosis at high concentration. Biochem. Biophys. Res. Commun. 263: 398–404.
Lowe SW. (1995) Cancer therapy and p53. Curr. Opin. Oncol. 7: 547–553.
Xu L, Pirollo KF, Chang EH. (2001) Tumor-targeted p53-gene therapy enhances the efficacy of conventional chemo/radiotherapy. J. Control. Release 74: 115–128.
Hortobagyi GN. (1999) Recent progress in the clinical development of docetaxel (Taxotere). Semin. Oncol. 26: 32–36.
Zeng S, Chen YZ, Fu L, Johnson KR, Fan W. (2000) In vitro evaluation of schedule-dependent interactions between docetaxel and doxorubicin against human breast and ovarian cancer cells. Clin. Cancer Res. 6: 3766–3773.
Miyake H, Hara S, Arakawa S, Kamidono S, Hara I. (2001) Overexpression of Bcl-2 regulates sodium butyrate- and/or docetaxel-induced apoptosis in human bladder cancer cells both in vitro and in vivo. Int. J. Cancer 93: 26–32.
Muenchen HJ, Poncza PJ, Pienta KJ. (2001) Different docetaxel-induced apoptotic pathways are present in prostate cancer cell lines LNCaP and PC-3. Urology 57: 366–370.
Khayat D, Chollet P, Antoine EC, Monfardini S, Ambrosini G, Benhammouda A, Mazen MF, Sorio R, Borg-Olivier O, Riva A, Ramazeilles C, Azli N. (2001) Phase II study of sequential administration of docetaxel followed by doxorubicin and cyclophosphamide as first-line chemotherapy in metastatic breast cancer. J. Clin. Oncol. 19: 3367–3375.
Viens P, Roche H, Kerbrat P, Fumoleau P, Guastalla JP, Delozier T. (2001) Epirubicin-docetaxel combination in firstline chemotherapy for patients with metastatic breast cancer: final results of a dose-finding and efficacy study. Am. J. Clin. Oncol. 24: 328–335.
Hainsworth JD, Burris HA 3rd, Yardley DA, Bradof JE, Grimaldi M, Kalman LA, Sullivan T, Baker M, Erland JB, Greco FA. (2001) Weekly docetaxel in the treatment of elderly patients with advanced breast cancer: a Minnie Pearl Cancer Research Network phase II trial. J. Clin. Oncol. 19: 3500–3505.
Liu TJ, Zhang WW. Taylor DL, Roth JA, Goepfert H, Clayman GL. (1994) Growth suppression of human head and neck cancer cells by the introduction of a wild-type p53 gene via a recombinant adenovirus. Cancer Res 54: 3662–3667.
Acknowledgments
We are grateful to Dr. David Fitzgerald for providing the plasmid pDFH2T-vecOK containing the TfRscFv cDNA, Dr. John de Kruif for providing the expression vector pLP1, Dr. Arnold Levine for providing the plasmid pBP100, Ms. Tina Wilson and Kelle Reames for assistance with the in vivo animal studies, Dr. Wei-Qun Huang for assistance with Western analysis, Dr. Karen Creswell for assistance with flow cytometry analysis, and Leanne Sleer for assistance with the manuscript. This work was supported in part by NCI grant R01 CA45158 (E. C.) and NCI STTR Phase I grant R41 CA80449 (E. C.), and SynerGene Therapeutics, Inc.
Author information
Authors and Affiliations
Corresponding author
Rights and permissions
About this article
Cite this article
Xu, L., Tang, WH., Huang, CC. et al. Systemic p53 Gene Therapy of Cancer with Immunolipoplexes Targeted by Anti-Transferrin Receptor scFv. Mol Med 7, 723–734 (2001). https://doi.org/10.1007/BF03401962
Accepted:
Published:
Issue Date:
DOI: https://doi.org/10.1007/BF03401962