Abstract
Introduction
Extracellular vesicles (EVs) are promising carriers for the delivery of biotherapeutic cargo such as RNA and proteins. We have previously demonstrated that the innate EV mitochondria in microvesicles (MVs), but not exosomes (EXOs) can be transferred to recipient BECs and mouse brain slice neurons. Here, we sought to determine if the innate EV mitochondrial load can be further increased via increasing mitochondrial biogenesis in the donor cells. We hypothesized that mitochondria-enriched EVs (“mito-EVs”) may increase the recipient BEC ATP levels to a greater extent than naïve MVs.
Methods
We treated NIH/3T3, a fibroblast cell line and hCMEC/D3, a human brain endothelial cell (BEC) line using resveratrol to activate peroxisome proliferator-activated receptor-gamma coactivator-1α (PGC-1α), the central mediator of mitochondrial biogenesis. Naïve EVs and mito-EVs isolated from the non-activated and activated donor cells were characterized using transmission electron microscopy, dynamic light scattering and nanoparticle tracking analysis. The effect of mito-EVs on resulting ATP levels in the recipient BECs were determined using Cell Titer Glo ATP assay. The uptake of Mitotracker Red-stained EVs into recipient BECs and their colocalization with recipient BEC mitochondria were studied using flow cytometry and fluorescence microscopy.
Results
Resveratrol treatment increased PGC-1α expression in the donor cells. Mito-MVs but not mito-EXOs showed increased expression of mitochondrial markers ATP5A and TOMM20 compared to naïve MVs. TEM images showed that a greater number of mito-MVs contained mitochondria compared to naïve MVs. Mito-MVs but not mito-EXOs showed a larger particle diameter compared to their naïve EV counterparts from the non-activated cells suggesting increased mitochondria incorporation. Mito-EVs were generated at higher particle concentrations compared to naïve EVs from non-activated cells. Mito-EVs increased the cellular ATP levels and transferred their mitochondrial load into the recipient BECs. Mito-MV mitochondria also colocalized with recipient BEC mitochondria.
Conclusions
Our results suggest that the pharmacological modulation of mitochondrial biogenesis in the donor cells can change the mitochondrial load in the secreted MVs. Outcomes of physicochemical characterization studies and biological assays confirmed the superior effects of mito-MVs compared to naïve MVs—suggesting their potential to improve mitochondrial function in neurovascular and neurodegenerative diseases.









Similar content being viewed by others
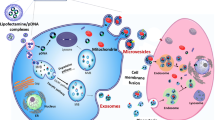
References
Ahluwalia, M., M. Kumar, P. Ahluwalia, S. Rahimi, J. R. Vender, R. P. Raju, et al. Rescuing mitochondria in traumatic brain injury and intracerebral hemorrhages—a potential therapeutic approach. Neurochem. Int.150:105192, 2021.
Andreux, P. A., R. H. Houtkooper, and J. Auwerx. Pharmacological approaches to restore mitochondrial function. Nat. Rev. Drug Discov. 12(6):465–483, 2013.
Bernardo-Castro, S., J. A. Sousa, A. Brás, C. Cecília, B. Rodrigues, L. Almendra, et al. Pathophysiology of blood–brain barrier permeability throughout the different stages of ischemic stroke and its implication on hemorrhagic transformation and recovery. Front. Neurol. 11:1605, 2020.
Busatto, S., A. Pham, A. Suh, S. Shapiro, and J. Wolfram. Organotropic drug delivery: synthetic nanoparticles and extracellular vesicles. Biomed. Microdevices. 21(2):46, 2019.
Chazotte, B. Labeling mitochondria with MitoTracker dyes. Cold Spring Harb. Protoc. 2011(8):990–992, 2011.
Chen, S., Q. Fan, A. Li, D. Liao, J. Ge, A. M. Laties, et al. Dynamic mobilization of PGC-1α mediates mitochondrial biogenesis for the protection of RGC-5 cells by resveratrol during serum deprivation. Apoptosis. 18(7):786–799, 2013.
Csiszar, A., N. Labinskyy, J. T. Pinto, P. Ballabh, H. Zhang, G. Losonczy, et al. Resveratrol induces mitochondrial biogenesis in endothelial cells. Am. J. Physiol. Heart Circ. Physiol. 297(1):H13–H20, 2009.
Currais, A. Ageing and inflammation—a central role for mitochondria in brain health and disease. Ageing Res. Rev. 21:30–42, 2015.
D’Souza, A., K. M. Dave, R. A. Stetler, and D. S. Manickam. Targeting the blood–brain barrier for the delivery of stroke therapies. Adv. Drug Deliv. Rev. 171:332–351, 2021.
Dai, X., J. Chen, F. Xu, J. Zhao, W. Cai, Z. Sun, et al. TGFalpha preserves oligodendrocyte lineage cells and improves white matter integrity after cerebral ischemia. J. Cereb. Blood Flow Metab. 40(3):639–655, 2020.
Dave, K., M. J. Reynolds, D. Stolz, R. Babidhan, D. Dobbins, H. Yankello, et al. Extracellular vesicles deliver mitochondria and HSP27 protein to protect the blood–brain barrier. bioRxiv. 2022. https://doi.org/10.1101/2021.10.29.466491.
Dave, K. M., W. Zhao, C. Hoover, A. D’Souza, and D. S. Manickam. Extracellular vesicles derived from a human brain endothelial cell line increase cellular ATP levels. AAPS PharmSciTech. 22(1):18, 2021.
Davinelli, S., N. Sapere, M. Visentin, D. Zella, and G. Scapagnini. Enhancement of mitochondrial biogenesis with polyphenols: combined effects of resveratrol and equol in human endothelial cells. Immunity Ageing. 10(1):28, 2013.
de Jong, O. G., S. A. A. Kooijmans, D. E. Murphy, L. Jiang, M. J. W. Evers, J. P. G. Sluijter, et al. Drug delivery with extracellular vesicles: from imagination to innovation. Acc. Chem. Res. 52(7):1761–1770, 2019.
Delmas, D., V. Aires, D. J. Colin, E. Limagne, A. Scagliarini, A. K. Cotte, et al. Importance of lipid microdomains, rafts, in absorption, delivery, and biological effects of resveratrol. Ann. N. Y. Acad. Sci. 1290(1):90–97, 2013.
Doll, D. N., H. Hu, J. Sun, S. E. Lewis, J. W. Simpkins, and X. Ren. Mitochondrial crisis in cerebrovascular endothelial cells opens the blood–brain barrier. Stroke. 46(6):1681–1689, 2015.
D’Souza, A., A. Burch, K. M. Dave, A. Sreeram, M. J. Reynolds, D. X. Dobbins, et al. Microvesicles transfer mitochondria and increase mitochondrial function in brain endothelial cells. J. Control. Release. 338:505–526, 2021.
Duchen, M. R. Mitochondria in health and disease: perspectives on a new mitochondrial biology. Mol. Aspects Med. 25(4):365–451, 2004.
Elsharkasy, O. M., J. Z. Nordin, D. W. Hagey, O. G. de Jong, R. M. Schiffelers, S. E. L. Andaloussi, et al. Extracellular vesicles as drug delivery systems: why and how? Adv. Drug Deliv. Rev. 159:332–343, 2020.
Evers, M. J. W., S. I. van de Wakker, E. M. de Groot, O. G. de Jong, J. J. J. Gitz-François, C. S. Seinen, et al. Functional siRNA delivery by extracellular vesicle-liposome hybrid nanoparticles. Adv. Healthc. Mater. 11(5):e2101202, 2022.
Fernandez-Marcos, P. J., and J. Auwerx. Regulation of PGC-1α, a nodal regulator of mitochondrial biogenesis. Am. J. Clin. Nutr. 93(4):884S-S890, 2011.
Fiskum, G., A. N. Murphy, and M. F. Beal. Mitochondria in neurodegeneration: acute ischemia and chronic neurodegenerative diseases. J. Cereb. Blood Flow Metab. 19(4):351–369, 1999.
Friedman, J. R., and J. Nunnari. Mitochondrial form and function. Nature. 505(7483):335–343, 2014.
Gray, W. D., A. J. Mitchell, and C. D. Searles. An accurate, precise method for general labeling of extracellular vesicles. MethodsX. 2:360–367, 2015.
Hayakawa, K., S. J. Chan, E. T. Mandeville, J. H. Park, M. Bruzzese, J. Montaner, et al. Protective effects of endothelial progenitor cell-derived extracellular mitochondria in brain endothelium. Stem Cells. 36(9):1404–1410, 2018.
Hayakawa, K., E. Esposito, X. Wang, Y. Terasaki, Y. Liu, C. Xing, et al. Transfer of mitochondria from astrocytes to neurons after stroke. Nature. 535(7613):551–555, 2016.
Herrmann, I. K., M. J. A. Wood, and G. Fuhrmann. Extracellular vesicles as a next-generation drug delivery platform. Nat. Nanotechnol. 16(7):748–759, 2021.
Ikeda, G., M. R. Santoso, Y. Tada, A. M. Li, E. Vaskova, J. H. Jung, et al. Mitochondria-rich extracellular vesicles from autologous stem cell-derived cardiomyocytes restore energetics of ischemic myocardium. J. Am. Coll. Cardiol. 77(8):1073–1088, 2021.
Islam, M. N., S. R. Das, M. T. Emin, M. Wei, L. Sun, K. Westphalen, et al. Mitochondrial transfer from bone-marrow-derived stromal cells to pulmonary alveoli protects against acute lung injury. Nat. Med. 18:759, 2012.
Kaushik, P., M. Ali, M. Salman, H. Tabassum, and S. Parvez. Harnessing the mitochondrial integrity for neuroprotection: therapeutic role of piperine against experimental ischemic stroke. Neurochem. Int.149:105138, 2021.
Kim, A., W. B. Ng, W. Bernt, and N.-J. Cho. Validation of size estimation of nanoparticle tracking analysis on polydisperse macromolecule assembly. Sci. Rep. 9(1):2639, 2019.
Komen, J. C., and D. R. Thorburn. Turn up the power—pharmacological activation of mitochondrial biogenesis in mouse models. Br. J. Pharmacol. 171(8):1818–1836, 2014.
Lamichhane, T. N., A. Jeyaram, D. B. Patel, B. Parajuli, N. K. Livingston, N. Arumugasaamy, et al. Oncogene knockdown via active loading of small RNAs into extracellular vesicles by sonication. Cell. Mol. Bioeng. 9(3):315–324, 2016.
Lee, M. J., Y. Jang, J. Han, S. J. Kim, X. Ju, Y. L. Lee, et al. Endothelial-specific Crif1 deletion induces BBB maturation and disruption via the alteration of actin dynamics by impaired mitochondrial respiration. J. Cereb. Blood Flow Metab. 40(7):1546–1561, 2020.
Lin, J., C. Handschin, and B. M. Spiegelman. Metabolic control through the PGC-1 family of transcription coactivators. Cell Metab. 1(6):361–370, 2005.
López-Lluch, G., P. M. Irusta, P. Navas, and R. de Cabo. Mitochondrial biogenesis and healthy aging. Exp. Gerontol. 43(9):813–819, 2008.
Madineni, A., Q. Alhadidi, and Z. A. Shah. Cofilin inhibition restores neuronal cell death in oxygen-glucose deprivation model of ischemia. Mol. Neurobiol. 53(2):867–878, 2016.
Manickam, D. S. Delivery of mitochondria via extracellular vesicles—a new horizon in drug delivery. J. Control. Release. 343:400–407, 2022.
Moreira, P. I., C. Carvalho, X. Zhu, M. A. Smith, and G. Perry. Mitochondrial dysfunction is a trigger of Alzheimer’s disease pathophysiology. Biochim. Biophys. Acta. 1802(1):2–10, 2010.
Morrison, T. J., M. V. Jackson, E. K. Cunningham, A. Kissenpfennig, D. F. McAuley, C. M. O’Kane, et al. Mesenchymal stromal cells modulate macrophages in clinically relevant lung injury models by extracellular vesicle mitochondrial transfer. Am. J. Respir. Crit. Care Med. 196(10):1275–1286, 2017.
Murphy, D. E., O. G. de Jong, M. Brouwer, M. J. Wood, G. Lavieu, R. M. Schiffelers, et al. Extracellular vesicle-based therapeutics: natural versus engineered targeting and trafficking. Exp. Mol. Med. 51(3):1–12, 2019.
Murphy, D. E., O. G. de Jong, M. J. W. Evers, M. Nurazizah, R. M. Schiffelers, and P. Vader. Natural or synthetic RNA delivery: a stoichiometric comparison of extracellular vesicles and synthetic nanoparticles. Nano Lett. 21(4):1888–1895, 2021.
Neves, A. R., C. Nunes, and S. Reis. Resveratrol induces ordered domains formation in biomembranes: Implication for its pleiotropic action. Biochim. Biophys. Acta. 1858(1):12–18, 2016.
Nian, K., I. C. Harding, I. M. Herman, and E. E. Ebong. Blood–brain barrier damage in ischemic stroke and its regulation by endothelial mechanotransduction. Front. Physiol. 11:1681, 2020.
van Niel, G., D. R. F. Carter, A. Clayton, D. W. Lambert, G. Raposo, and P. Vader. Challenges and directions in studying cell–cell communication by extracellular vesicles. Nat. Rev. Mol. Cell Biol. 23:369–382, 2022.
Oldendorf, W. H., M. E. Cornford, and W. J. Brown. The large apparent work capability of the blood–brain barrier: a study of the mitochondrial content of capillary endothelial cells in brain and other tissues of the rat. Ann. Neurol. 1(5):409–417, 1977.
Peng, K., Y. Tao, J. Zhang, J. Wang, F. Ye, G. Dan, et al. Resveratrol regulates mitochondrial biogenesis and fission/fusion to attenuate rotenone-induced neurotoxicity. Oxid. Med. Cell Longev. 2016:6705621, 2016.
Phinney, D. G., M. Di Giuseppe, J. Njah, E. Sala, S. Shiva, C. M. St Croix, et al. Mesenchymal stem cells use extracellular vesicles to outsource mitophagy and shuttle microRNAs. Nat. Commun. 6(1):8472, 2015.
Puhm, F., T. Afonyushkin, U. Resch, G. Obermayer, M. Rohde, T. Penz, et al. Mitochondria are a subset of extracellular vesicles released by activated monocytes and induce type I IFN and TNF responses in endothelial cells. Circ. Res. 125(1):43–52, 2019.
Ragonese, F., L. Monarca, A. De Luca, L. Mancinelli, M. Mariani, C. Corbucci, et al. Resveratrol depolarizes the membrane potential in human granulosa cells and promotes mitochondrial biogenesis. Fertil. Steril. 115(4):1063–1073, 2021.
Raposo, G., and W. Stoorvogel. Extracellular vesicles: exosomes, microvesicles, and friends. J Cell Biol. 200(4):373–383, 2013.
Razmara, A., L. Sunday, C. Stirone, X. B. Wang, D. N. Krause, S. P. Duckles, et al. Mitochondrial effects of estrogen are mediated by estrogen receptor alpha in brain endothelial cells. J. Pharmacol. Exp. Ther. 325(3):782–790, 2008.
Sansone, P., C. Savini, I. Kurelac, Q. Chang, L. B. Amato, A. Strillacci, et al. Packaging and transfer of mitochondrial DNA via exosomes regulate escape from dormancy in hormonal therapy-resistant breast cancer. Proc. Natl Acad. Sci. 114(43):E9066, 2017.
Scarpulla, R. C. Metabolic control of mitochondrial biogenesis through the PGC-1 family regulatory network. Biochim. Biophys. Acta. 1813(7):1269–78, 2011.
Scarpulla, R. C., R. B. Vega, and D. P. Kelly. Transcriptional integration of mitochondrial biogenesis. Trends Endocrinol. Metab. 23(9):459–466, 2012.
Silva, J. D., Y. Su, C. S. Calfee, K. L. Delucchi, D. Weiss, D. F. McAuley, et al. MSC extracellular vesicles rescue mitochondrial dysfunction and improve barrier integrity in clinically relevant models of ARDS. Eur. Respir. J. 58(1):2002978, 2020.
Spees, J. L., S. D. Olson, M. J. Whitney, and D. J. Prockop. Mitochondrial transfer between cells can rescue aerobic respiration. Proc. Natl Acad. Sci. U.S.A. 103(5):1283, 2006.
Torralba, D., F. Baixauli, and F. Sánchez-Madrid. Mitochondria know no boundaries: mechanisms and functions of intercellular mitochondrial transfer. Front. Cell Dev. Biol. 4:107, 2016.
Torralba, D., F. Baixauli, C. Villarroya-Beltri, I. Fernández-Delgado, A. Latorre-Pellicer, R. Acín-Pérez, et al. Priming of dendritic cells by DNA-containing extracellular vesicles from activated T cells through antigen-driven contacts. Nat. Commun. 9(1):2658, 2018.
Vader, P., X. O. Breakefield, and M. J. A. Wood. Extracellular vesicles: emerging targets for cancer therapy. Trends Mol. Med. 20(7):385–393, 2014.
Vader, P., E. A. Mol, G. Pasterkamp, and R. M. Schiffelers. Extracellular vesicles for drug delivery. Adv. Drug Deliv. Rev. 106:148–156, 2016.
Ventura-Clapier, R., A. Garnier, and V. Veksler. Transcriptional control of mitochondrial biogenesis: the central role of PGC-1α. Cardiovasc. Res. 79(2):208–217, 2008.
Walker, S., S. Busatto, A. Pham, M. Tian, A. Suh, K. Carson, et al. Extracellular vesicle-based drug delivery systems for cancer treatment. Theranostics. 9(26):8001–8017, 2019.
Wallace, D. C. A mitochondrial paradigm of metabolic and degenerative diseases, aging, and cancer: a dawn for evolutionary medicine. Annu. Rev. Genet. 39:359–407, 2005.
Weissig, V. Drug Development for the therapy of mitochondrial diseases. Trends Mol. Med. 26(1):40–57, 2020.
Weissig, V., S.-M. Cheng, and G. G. M. D’Souza. Mitochondrial pharmaceutics. Mitochondrion. 3(4):229–244, 2004.
Wen, Y., W. Li, E. C. Poteet, L. Xie, C. Tan, L. J. Yan, et al. Alternative mitochondrial electron transfer as a novel strategy for neuroprotection. J. Biol. Chem. 286(18):16504–16515, 2011.
Wenz, T. Regulation of mitochondrial biogenesis and PGC-1α under cellular stress. Mitochondrion. 13(2):134–142, 2013.
Whitaker, R. M., D. Corum, C. C. Beeson, and R. G. Schnellmann. Mitochondrial biogenesis as a pharmacological target: a new approach to acute and chronic diseases. Annu. Rev. Pharmacol. Toxicol. 56:229–249, 2016.
Witwer, K. W., and J. Wolfram. Extracellular vesicles versus synthetic nanoparticles for drug delivery. Nat. Rev. Mater. 6(2):103–106, 2021.
Zhang, Z., Z. Ma, C. Yan, K. Pu, M. Wu, J. Bai, et al. Muscle-derived autologous mitochondrial transplantation: a novel strategy for treating cerebral ischemic injury. Behav. Brain Res. 356:322–331, 2019.
Zhao, Q., Z. Tian, G. Zhou, Q. Niu, J. Chen, P. Li, et al. SIRT1-dependent mitochondrial biogenesis supports therapeutic effects of resveratrol against neurodevelopment damage by fluoride. Theranostics. 10(11):4822–4838, 2020.
Zhou, J., Z. Yang, R. Shen, W. Zhong, H. Zheng, Z. Chen, et al. Resveratrol improves mitochondrial biogenesis function and activates PGC-1α pathway in a preclinical model of early brain injury following subarachnoid hemorrhage. Front. Mol. Biosci. 8:223, 2021.
Funding
Maura Farinelli and Abigail Sullivan’s NURE fellowship were supported through a grant from the National Institute of Neurological Disorders and Stroke (5R25NS100118-04) and this study was supported via start-up funds to Devika S. Manickam (Duquesne University).
Conflict of interest
The authors declare no conflicts of interest.
Author information
Authors and Affiliations
Corresponding author
Additional information
Associate Editor Michael R. King oversaw the review of this article.
Publisher's Note
Springer Nature remains neutral with regard to jurisdictional claims in published maps and institutional affiliations.
This article is part of the 2022 CMBE Young Innovators special issue.
Supplementary Information
Below is the link to the electronic supplementary material.
Rights and permissions
Springer Nature or its licensor holds exclusive rights to this article under a publishing agreement with the author(s) or other rightsholder(s); author self-archiving of the accepted manuscript version of this article is solely governed by the terms of such publishing agreement and applicable law.
About this article
Cite this article
Dave, K.M., Dobbins, D.X., Farinelli, M.N. et al. Engineering Extracellular Vesicles to Modulate Their Innate Mitochondrial Load. Cel. Mol. Bioeng. 15, 367–389 (2022). https://doi.org/10.1007/s12195-022-00738-8
Received:
Accepted:
Published:
Issue Date:
DOI: https://doi.org/10.1007/s12195-022-00738-8