Abstract
In this work, effective thermal conductivity (λEff) of fractured rock masses was numerically investigated. A two-dimensional Discrete Fracture Network (DFN) model of the fractured rock masses was established based on the statistic results of natural fracture development in a potential area for high level radioactive waste disposal in China. Steady state heat transfer processes in the fractured granite rock masses were numerically simulated using finite element method (FEM). The calculated λEff values of the fractured granite rock masses in dry and saturated conditions are 1.99 W/(m K) and 2.31 W/(m K), respectively. Compared with the thermal conductivity of intact granite [λIntact, 2.5 W/(m K)], the drop rates are 20.4% and 7.6%, respectively. Sensitivity analysis was conducted on the main model parameters including fracture density (FDensity), trace length (FLength), thermal contact resistance (FTCR), and λIntact. The results indicate the relation between λEff and three fracture parameters (FDensity, Flength and FTCR) can be fitted using power law or negative exponent functions with good consistency. When fracture network parameters remain unchanged, λEff is in linear positive correlation to λIntact. The slop of the fitted line is determined by the fracture network parameters. Due to the fact that distribution of generated fractures in different directions are quite uniform, λEff did not show significant difference in different directions. On the basis of the above-mentioned results, an estimation model was proposed for the determination of λEff of fractured rock masses using P21 (total length of fracture traces per unit area), FTCR, and λIntact. The proposed estimation model shows good consistency to the calculated results of FEM model.










Similar content being viewed by others
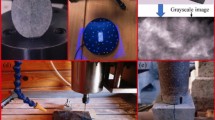
References
Abdulagatova Z, Abdulagatov IM, Emirov VN (2009) Effect of temperature and pressure on the thermal conductivity of sandstone. Int J Rock Mech Min 46:1055–1071
Albert K, Schulze M, Franz C, Koenigsdorff R, Zosseder K (2017) Thermal conductivity estimation model considering the effect of water saturation explaining the heterogeneity of rock thermal conductivity. Geothermics 66:1–12
Bahrami M, Culham JR, Yananovich MM, Schneider GE (2006) Review of thermal joint resistance models for nonconforming rough surfaces. Appl Mech Rev 59(1):411–431
Barbier E (2002) Geothermal energy technology and current status: an overview. Renew Sust Energ Rev 6(1):3–65
Burkhardt H, Honarmand H, Pribnow D (1995) Test measurements with a new thermal conductivity borehole tool. Tectonophysics 244(s 1–3):161–165
Cherubini C, Pastore N, Giasi CI, Allegretti NM (2017) Laboratory experimental investigation of heat transport in fractured media. Nonlin Process Geophys 24:23–42
Deere DU (1968) Geological consideration. In: Stagg KG, Zienkiewicz OC (eds) Rock mechanics in engineering practice. Wiley, New York, pp 1–20
Farid MM, Khudhair AM, Razack SAK, Hallaj SA (2004) A review on phase change energy storage: materials and applications. Energy Convers Manag 45(9):1597–1615
Garcia AV, Santamarina JC (2021) Heat flow in fractured rocks: stress and moisture-dependent thermal contact resistance. Geothermics 95:10213
Gens A, Sánchez M, Guimarães L, Do N, Alonso EE, Lloret A, Olivella S, Villar MV, Huertas F (2009) A full-scale in situ heating test for high-level nuclear waste disposal: observations, analysis and interpretation. Geotechnique 59:377–399
Guo Z (2018) Influence of thermal treatment on the thermal conductivity characteristics of Beishan granite. Msc thesis, Beijing Research Institute of Uranium Geology (in Chinese)
Jia GS, Tao ZY, Meng XZ, Ma CF, Chai JC, Jin LW (2019) Review of effective thermal conductivity models of rock-soil for geothermal energy applications. Geothermics 77:1–11
Jorand R, Vogt C, Marquart G, Clauser C (2013) Effective thermal conductivity of heterogeneous rocks from laboratory experiments and numerical modeling. J Geophys Res-Sol Earth 118(10):5225–5235
Lei G, Yang C, Wang G, Wei X, Chen SW, Ji GD, Huo L (2016) Geometric features of joints and quality evaluation of rock mass in Xinchang section. Beishan Chin J Rock Mech Eng 35(5):896–905 (in Chinese)
Li ZW, Feng XT, Zhang YJ, Xu TF (2018) Feasibility study of developing a geothermal heating system in naturally fractured formations: reservoir hydraulic properties determination and heat production forecast. Geothermics 73:1–15
Li ZW, Feng XT, Zhang YJ, Gong YH, Zhu GQ (2020a) Effect of mechanical damage on the thermal conductivity of granite. Rock Mech Rock Eng 53:1039–1051
Li ZW, Zhang YJ, Gong YH, Zhu GQ (2020b) Influences of mechanical damage and water saturation on the distributed thermal conductivity of granite. Geothermics 83:101736
Long JCS, Hestir K, Karasaki K, Davey A, Peterson J, Kemeny J, Landsfeld M (1991) Fluid flow in fractured rock: theory and application. In: Bear J, Corapcioglu MY (eds) Transport Processes in porous media. NATO ASI Series (Series E: Applied Sciences), vol 202. Springer, Dordrecht
Lu W, Xiang YY (2012) Experiments and sensitivity analyses for heat transfer in a meter-scale regularly fractured granite model with water flow. J Zhejiang Univ-Sci A (appl Phys & Eng) 13(12):958–968
Luo H, Wang J, Jiang S, Zhao HG, Jin YX (2019) Construction and application of three-dimensional geological model in Xinchang Block for high-level radioactive waste disposal. Geophys Geochem Explor 43(3):568–575 (in Chinese)
Mügler C, Filippi M, Montarnal P, Martinez JM, Wileveau Y (2006) Determination of the thermal conductivity of Opalinus Clay via simulations of experiments performed at the Mont Terri underground laboratory. J Appl Geophys 58:112–129
Nemoto K, Watanabe N, Hirano N, Tsuchiya N (2009) Direct measurement of contact area and stress dependence of anisotropic flow through rock fracture with heterogeneous aperture distribution. Earth Planet Sci Lett 281:81–87
Nguyen ST, Vu M-H, Vu MN, Tang AM (2017) Modeling of heat flow and effective thermal conductivity of fractured media: analytical and numerical methods. J Appl Geophys 140:117–122
Ou XG, Jin ZM, Wang L, Xu HJ, Jin SY (2004) Thermal conductivity and its anisotropy of rocks from the depth of 100–2000m mainhole of Chinese continental scientific drilling: revelations to the study on thermal structure of subduction zone. Acta Petrol Sin 20(1):109–118 (in Chinese)
Popov Y, Berezin V, Soloviov V, Romuschkevitch R, Korosteliov V, Kostiurin A, Kulikov A (1987) Thermal conductivity of minerals. Izv-Phys Solid Earth 23(3):245–253
Popov Y, Beardsmore G, Clauser C, Roy S (2016) ISRM suggested methods for determining thermal properties of rocks from laboratory tests at atmospheric pressure. Rock Mech Rock Eng 49(10):4179–4207
Pribnow DFC, Sass JH (1995) Determination of thermal conductivity for deep boreholes. J Geophys Res-Sol Earth 100(B6):9981–9994
Vosteen HD, Schellschmidt R (2003) Influence of temperature on thermal conductivity, thermal capacity and thermal diffusivity for different types of rock. Phys Chem Earth 28(9):499–509
Wang J, Chen L, Su R, Zhao XG (2018) The Beishan underground research laboratory for geological disposal of high-level radioactive waste in China: planning, site selection, site characterization and in situ tests. J Rock Mech Geotech 10:411–435
Williams CF, Anderson RN (1990) Thermophysical properties of the Earth’s crust: In situ measurements from continental and ocean drilling. J Geophys Res-Sol Ea 95(B6):9209–9236
Xie H, Pei J, Zuo J, Zhang R (2011) Investigation of mechanical properties of fractured marbles by uniaxial compression tests. J Rock Mech Geotech 4:302–313
Zhang GK, Xu WY (2008) Analysis of joint network simulation method and REV scale. Rock Soil Mech 29(6):1675–1680 (in Chinese)
Zhang C, Chen Q, Qin X, Hong B, Meng W, Zhang Q (2017a) In-situ stress and fracture characterization of a candidate repository for spent nuclear fuel in Gansu, northwestern China. Eng Geol 231:218–229
Zhang W, Zhao QH, Huang RQ, Ma DH, Chen JP, Xu PH, Que JS (2017b) Determination of representative volume element considering the probability that a sample can represent the investigated rock mass at Baihetan Dam site, China. Rock Mech Rock Eng 50:2817–2825
Zhang X, Wu Y, Zhai E, Ye P (2021) Coupling analysis of the heat-water dynamics and frozen depth in a seasonally frozen zone. J Hydrol 593:125603
Zhao XG, Zhao Z, Guo Z, Cai M, Wang J (2018) Influence of thermal treatment on the thermal conductivity of Beishan granite. Rock Mech Rock Eng 51(7):2055–2074
Acknowledgements
This study was supported by the National Natural Science Foundation of China (Grant no. 41702332), the Fundamental Research Funds for the Central Universities (Grant no. N2001017).
Author information
Authors and Affiliations
Corresponding author
Ethics declarations
Conflicts of interest
The authors wish to confirm that there are no known conflicts of interest associated with this publication and there has been no significant support for this work that could have influenced its outcome.
Additional information
Publisher's Note
Springer Nature remains neutral with regard to jurisdictional claims in published maps and institutional affiliations.
Rights and permissions
About this article
Cite this article
Li, ZW., Liu, Y., Mei, SM. et al. Effective Thermal Conductivity Estimation of Fractured Rock Masses. Rock Mech Rock Eng 54, 6191–6206 (2021). https://doi.org/10.1007/s00603-021-02599-5
Received:
Accepted:
Published:
Issue Date:
DOI: https://doi.org/10.1007/s00603-021-02599-5