Abstract
The heat transport properties of feldspar, one of the major minerals of the crust, are important for constraining the thermal state of the Earth’s crust. The thermal diffusivity (D) and thermal conductivity (κ) of two natural alkali feldspars, namely, perthite and albite (Ab), were simultaneously measured at high temperatures (300–873 K) and high pressures (0.8–3 GPa) using a transient plane-source method. The present results show that the D and κ of these alkali feldspars decreased with the increase in temperature, whereas the κ of perthite remained almost constant at above 450 K. The D and κ of these samples decreased by 24–35% and 8–21% when the temperature increased from 300 to 873 K, respectively, suggesting that phonon conduction may be the dominant mechanism. The D and κ of these samples also exhibited a positive pressure dependence as indicated by their positive pressure coefficients: 0.052–0.098 mm2s−1GPa−1 for D and 0.189–0.325 Wm−1K−1GPa−1 for κ. Combining previous data with the results of this study, the D and κ of an intermediate albite-orthoclase solid solution can be reasonably estimated by an empirical model. Furthermore, the average κ of the crust was recalculated to accurately constrain the thermal thickness and temperature of the lithosphere. The present estimate suggests that partial melting can occur at shallow depths of the middle and lower crust, which may provide a new understanding of the low-velocity and high-conductivity anomalies revealed by geophysical observations in the crust.













Similar content being viewed by others
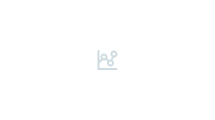
References
Akaogi M, Kamii N, Kishi A, Kojitani H (2004) Calorimetric study on high-pressure transitions in KAlSi3O8. Phys Chem Mineral 31:85–91
Anderson OL (1999) Mantle convection: a thermal balancing act. Science 283:1652–1653
Anderson OL, Isaak DG (1995) Elastic constants of mantle minerals at high temperature. Mineral Phys Crystallogr Handb Phys Constants 2:64–97
Benisek A, Dachs E, Kroll H (2009) Excess heat capacity and entropy of mixing in high structural state plagioclase. Am Mineral 94:1153–1161
Benusa MD, Angel RJ, Ross NL (2005) Compression of albite, NaAlSi3O8. Am Mineral 90:1115–1120
Branlund JM, Hofmeister AM (2012) Heat transfer in plagioclase feldspars. Am Mineral 97:1145–1154
Chapman DS (1986) Thermal gradients in the continental crust. Geol Soc Lond Spec Publ 24:63–70
Chapman DS, Furlong KP (1992) Thermal state of the continental crust. In: Fountain DM, Arculus R, Kay RW (eds) Continental lower crust. Elsevier, Amsterdam, pp 179–199
Dzhavadov LN (1975) Measurement of thermophysical properties of dielectrics under pressure. High Temp High Press 7:49–54
Fu HF, Zhang BH, Ge JH, Xiong ZL, Zhai SM, Shan SM, Li HP (2019) Thermal diffusivity and thermal conductivity of granitoids at 283–988 K and 0.3–1.5 GPa. Am Mineral 104:1533–1545
Furlong KP, Chapman DS (2013) Heat flow, heat generation, and the thermal state of the lithosphere. Annu Rev Earth Planet Sci 41:385–410
Ge JH, Zhang BH, Xiong ZL, He LF, Li HP (2021) Thermal properties of harzburgite and dunite at 0.8–3 GPa and 300–823 K and implications for the thermal evolution of Tibet. Geosci Front 12:947–956
Gibert B, Mainprice D (2009) Effect of crystal preferred orientations on the thermal diffusivity of quartz polycrystalline aggregates at high temperature. Tectonophysics 465:150–163
Hacker BR, Ritzwoller MH, Xie J (2014) Partially melted, mica-bearing crust in Central Tibet. Tectonics 33:1408–1424
Hasterok D, Chapman DS (2011) Heat production and geotherms for the continental lithosphere. Earth Planet Sci Lett 307:59–70
Höfer M, Schilling FR (2002) Heat transfer in quartz, orthoclase, and sanidine at elevated temperature. Phys Chem Mineral 29:571–584
Hofmeister AM (1999) Mantle values of thermal conductivity and the geotherm from phonon lifetimes. Science 283:1699–1706
Hofmeister AM (2007) Pressure dependence of thermal transport properties. Proc Natl Acad Sci USA 104:9192–9197
Hofmeister AM (2010) Thermal diffusivity of oxide perovskite compounds at elevated temperature. J Appl Phys 107:103532
Hofmeister AM, Branlund JM, Pertermann M (2007) Properties of rocks and minerals–thermal conductivity of the earth. Treatise Geophys 2:543–577
Hofmeister AM, Whittington AG, Pertermann M (2009) Transport properties of high albite crystals, near-endmember feldspar and pyroxene glasses, and their melts to high temperature. Contrib Mineral Petrol 158:381–400
Hofmeister AM, Dong J, Branlund JM (2014) Thermal diffusivity of electrical insulators at high temperatures: Evidence for diffusion of bulk phonon-polaritons at infrared frequencies augmenting phonon heat conduction. J Appl Phys 115:163517
Hofmeister AM, Carpenter PK (2015) Heat transport of micas. Can Mineral 53:557–570
Kanamori H, Fujii N, Mizutani H (1968) Thermal diffusivity measurement of rock-forming minerals from 300 to 1100 K. J Geophys Res 73:595–605
Lemenager A, O’Neill C, Zhang S, Evans M (2018) The effect of temperature-dependent thermal conductivity on the geothermal structure of the sydney basin. Geotherm Energy 6:1–27
Li S, Unsworth MJ, Booker JR, Wei W, Tan H, Jones AG (2003) Partial melt or aqueous fluid in the mid-crust of Southern Tibet? Constraints from INDEPTH magnetotelluric data. Geophys J Int 153:289–304
Linvill ML, Vandersande JW, Pohl RO (1984) Thermal conductivity of feldspars. Bull Mineral 107:521–527
Marquardt H, Ganschow S, Schilling FR (2009) Thermal diffusivity of natural and synthetic garnet solid solution series. Phys Chem Mineral 36:107–118
McKenzie D, Jackson J, Priestley K (2005) Thermal structure of oceanic and continental lithosphere. Earth Planet Sci Lett 233:337–349
Merriman JD, Whittington AG, Hofmeister AM, Nabelek PI, Benn K (2013) Thermal transport properties of major archean rock typesto high temperature and implications for cratonic geotherms. Precambrian Res 233:358–372
Miao S, Li H, Chen G (2014) The temperature dependence of thermal conductivity for lherzolites from the North China Craton and the associated constraints on the thermodynamic thickness of the lithosphere. Geophys J Inter 197:900–909
Michaut C, Jaupart C, Bell DR (2007) Transient geotherms in Archean continental lithosphere: new constraints on thickness and heat production of the subcontinental lithospheric mantle. J Geophys Res 112:1–17
Mottaghy D, Vosteen HD, Schellschmidt R (2008) Temperature dependence of the relationship of thermal diffusivity versus thermal conductivity for crystalline rocks. Int J Earth Sci 97:435–442
Nabelek PI, Hofmeister AM, Whittington AG (2012) The influence of temperature-dependent thermal diffusivity on the conductive cooling rates of plutons and temperature-time paths in contact aureoles. Earth Planet Sci Lett 317:157–164
Nelson KD, Zhao W, Brown LD, Kuo J, Che J, Liu X, Klemperer SL, Makovsky Y, Meissner R, Mechie J, Kind R, Wenzel F, Ni J, Nabelek J, Leshou C, Tan H, Wei W, Jones AG, Booker J, Unsworth M, Kidd W, Hauck M, Alsdorf D, Ross A, Cogan M, Wu C, Sandvol W, Edwards M (1996) Partially molten middle crust beneath southern Tibet: synthesis of project INDEPTH results. Science 274:1684–1688
Nestola F, Curetti N, Benna P, Ivaldi G, Angel RJ, Bruno E (2008) Compressibility and high-pressure behavior of Ab63Or27An10 anorthoclase. Can Mineral 46:1443–1454
Ohta K, Yagi T, Hirose K, Ohishi Y (2017) Thermal conductivity of ferropericlase in the Earth’s lower mantle. Earth Planet Sci Lett 465:29–37
Osako M, Ito E, Yoneda A (2004) Simultaneous measurements of thermal conductivity and thermal diffusivity for garnet and olivine under high pressure. Phys Earth Planet Inter 143:311–320
Osako M, Yoneda A, Ito E (2010) Thermal diffusivity, thermal conductivity, and heat capacity of serpentine (antigorite) under high pressure. Phys Earth Planet Inter 183:229–233
Padture NP, Klemens PG (1997) Low thermal conductivity in garnets. J Am Ceram Soc 80:1018–1020
Patiño Douce AE, Harris N (1998) Experimental constraints on Himalayan anatexis. J Petrol 39:689–710
Pertermann M, Hofmeister AM (2006) Thermal diffusivity of olivine-group minerals at high temperature. Am Mineral 91:1747–1760
Pertermann M, Whittington AG, Hofmeister AM, Spera FJ, Zayak J (2008) Transport properties of low-sanidine single-crystals, glasses and melts at high temperature. Contrib Mineral Petrol 155:689–702
Petitjean S, Rabinowicz M, Grégoire M, Chevrot S (2006) Differences between Archean and Proterozoic lithospheres: assessment of the possible major role of thermal conductivity. Geochem Geophys Geosyst 7:1–26
Rudnick RL, McDonough WF, O’Connell RJ (1998) Thermal structure, thickness and composition of continental lithosphere. Chem Geol 145:395–411
Smith JV, Brown WL (1988) Feldspar minerals: I crystal structures, physical, chemical and microtextural properties, 2nd edn. Springer, Berlin, pp 1–20
Smith DS, Fayette S, Grandjean S, Martin C, Telle R, Tonnessen T (2003) Thermal resistance of grain boundaries in alumina ceramics and refractories. J Am Ceram Soc 86:105–111
Stein CA, Stein SA (1992) A model for the global variation in oceanic depth and heat flow with lithospheric age. Nature 359:123–129
Thompson AB (1992) Water in the Earth’s upper mantle. Nature 358:295–302
Vosteen HD, Schellschmidt R (2003) Influence of temperature on thermal conductivity, thermal capacity and thermal diffusivity for different types of rock. Phys Chem Earth 28:499–509
Wang C, Yoneda A, Osako M, Ito E, Yoshino T, Jin Z (2014) Measurement of thermal conductivity of omphacite, jadeite, and diopside up to 14 GPa and 1000 K: Implication for the role of eclogite in subduction slab. J Geophys Res Solid Earth 119:6277–6287
Wei W, Unsworth M, Jones A, Booker J, Tan H, Nelson D, Chen L, Li S, Solon K, Bedrosian P, Jin S, Deng M, Ledo J, Kay D, Robert B (2001) Detection of widespread fluids in the Tibetan crust by magnetotelluric studies. Science 292:716–718
Whittington AG, Hofmeister AM, Nabelek PI (2009) Temperature-dependent thermal diffusivity of the Earth’s crust and implications for magmatism. Nature 458:319–321
Xiong ZL, Zhang BH (2019) Thermal properties of olivine, wadsleyite and ringwoodite—a review. Minerals 9:519
Yanagawa TKB, Nakada M, Yuen DA (2005) Influence of lattice thermal conductivity on thermal convection with strongly temperature-dependent viscosity. Earth Planet Space 57:15–28
Zhang BH, Ge JH, Xiong ZL, Zhai SM (2019a) Effect of water on the thermal properties of olivine with implications for lunar internal temperature. J Geophys Res Planet 124:3469–3481
Zhang Y, Yoshino T, Yoneda A, Osako M (2019b) Effect of iron content on thermal conductivity of olivine with implications for cooling history of rocky planets. Earth Planet Sci Lett 519:109–119
Zhang BH, Guo X, Yoshino T, Xia QK (2021) Electrical conductivity of melts: Implications for conductivity anomalies in the Earth’s mantle. Natl Sci Rev 8:nwab064. https://doi.org/10.1093/nsr/nwab064
Zheng YF, Chen YX (2016) Continental versus oceanic subduction zones. Natl Sci Rev 3:495–519
Acknowledgements
We thank the editor (H. Keppler) and two anonymous reviewers for their constructive comments that greatly improved the manuscript. This study was supported by Key Research Program of Frontier Sciences of CAS (ZDBS-LY-DQC015), CAS “Light of West China” program (Y9CR026 to X. G.), NSF of China (41773056, 41973056, 42072051). The authors declare no competing financial interests.
Author information
Authors and Affiliations
Corresponding authors
Additional information
Communicated by Hans Keppler.
Publisher's Note
Springer Nature remains neutral with regard to jurisdictional claims in published maps and institutional affiliations.
Supplementary Information
Below is the link to the electronic supplementary material.
Rights and permissions
About this article
Cite this article
Xiong, Z., Zhang, B., Ge, J. et al. Thermal diffusivity and thermal conductivity of alkali feldspar at 0.8–3 GPa and 300–873 K. Contrib Mineral Petrol 176, 42 (2021). https://doi.org/10.1007/s00410-021-01797-2
Received:
Accepted:
Published:
DOI: https://doi.org/10.1007/s00410-021-01797-2