Abstract
The traditional homogeneous JC constitutive model can be used to perform the simulation of the mechanical properties effectively during the cutting process. However, polycrystalline material properties are not considered, and the effect of grain boundaries on the cutting process is ignored. In order to study the effect of grain boundary on micro-deformation during the cutting process of Inconel718, the grain size through the EBSD experiment was obtained firstly. A finite element simulation model of dynamic cutting of 2D polycrystal with grain boundaries was established using Python language and MATLAB software. A new material constitutive model with grain boundary effect is established by introducing the JC model into the geometrically necessary dislocation (GNDs) theory. By writing a VUMAT subroutine to achieve secondary development of ABAQUS, the established constitutive model is used for cutting simulation. Through the comparison of experimental results and simulation results, the model can accurately reflect the changes in cutting force, cutting temperature, and equivalent stress. On this basis, the influence of temperature on geometrically necessary dislocations, the influence of grain boundaries on the evolution of chip morphology, and the initiation and propagation of cracks are further analyzed.




























Similar content being viewed by others
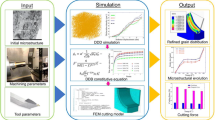
Data availability
All data generated or analyzed during this study are included in this published article.
Code availability
Not applicable.
References
Zhao R, Han JQ, Liu BB (2016) Interaction of forming temperature and grain size effect in micro/meso-scale plastic deformation of nickel-base superalloy. Mater Design 94:195–206. https://doi.org/10.1016/j.matdes.2016.01.022
Fang B, Yuan ZH, Li DP, Gao L (2021) Effect of ultrasonic vibration on finished quality in ultrasonic vibration assisted micromilling of Inconel718. Chinese J Aeronaut 34(6):209–219. https://doi.org/10.1016/j.cja.2020.09.021
An XL, Zhang B, Chu CL (2018) Evolution of microstructures and properties of the GH4169 superalloy during short-term and high-temperature processing. Mater Sci Eng A 744:255–266. https://doi.org/10.1016/j.msea.2018.12.019
Huang L, Cao Y, Zhang JH, Gao XS, Li GH, Wang YF (2021) Effect of heat treatment on the microstructure evolution and mechanical behaviour of a selective laser melted Inconel 718 alloy. J Alloy Compd: An Interdisc J Mater Sci and Solid-state Chem Phys 865(16):158613
Fan YH, Wang T, Hao ZP (2018) Research of plastic behavior in high-speed cutting Inconel718 based on multi-scale simulation. Int J Adv Manuf Tech 94:3731–3739. https://doi.org/10.1007/s00170-017-1121-4
Amrita M, Kamesh B (2021) Optimization of graphene based minimum quantity lubrication of Inconel718 turning with multiple machining performances. Mater Today: Proceed 39:1337–1344. https://doi.org/10.1016/j.matpr.2020.04.568
Ashtiani H, Shayanpoor AA (2021) New constitutive equation utilizing grain size for modeling of hot deformation behavior of AA1070 aluminum. T Nonferr Metal Soc 31(2):345–357. https://doi.org/10.1016/S1003-6326(21)65500-0
Li HT, Lai XM, Li CF, Feng J, Ni J (2007) Modelling and experimental analysis of the effects of tool wear, minimum chip thickness and micro tool geometry on the surface roughness in micro-end-milling. J Micromech Microeng 18:025006. https://doi.org/10.1088/0960-1317/18/2/025006
Kaira CS, Singh SS, Kirubanandham A et al (2016) Microscale deformation behavior of bicrystal boundaries in pure tin (Sn) using micropillar compression. Acta Mater 120:56–67. https://doi.org/10.1016/j.actamat.2016.08.030
Haque MA, Saif MTA (2002) Mechanical behavior of 30–50 nm thick aluminum films under uniaxial tension. Scripta Mater 47(12):863–867. https://doi.org/10.1016/S1359-6462(02)00306-8
AlMotasem AT, Posselt M, Bergström J (2018) Nanoindentation and nanoscratching of a ferrite/austenite iron bi-crystal: an atomistic study. Tribol Int 127:231–239. https://doi.org/10.1016/j.triboint.2018.06.017
Chang YH, Bai Y, Shimokawa T, Tsuji N, Murayama M (2021) A correlation between grain boundary character and deformation twin nucleation mechanism in coarse-grained high-Mn austenitic steel. Sci Rep 11:8468. https://doi.org/10.1038/S41598-021-87811-W
Sun H, Liang YL, Li G, Zhang XF, Wang S, Huang CW (2021) Dislocation hardening and phase transformation-induced high ductility in Ti-6Al-4V with a heterogeneous martensitic microstructure under tensile load. J Alloy Compd 868:159155. https://doi.org/10.1016/j.jallcom.2021.159155
Zhao J, Wang KH, Lv LX, Liu G (2019) Evolution and distribution of geometrically necessary dislocations for TA15 titanium alloy sheets during the hot tensile process. JOM 71:2303–2312. https://doi.org/10.1007/s11837-019-03490-z
Arsenlis A, Park DM, Becker R, Bulatov VV (2004) On the evolution of crystallographic dislocation density in non-homogeneously deforming crystals. J Mecha Phys Solid 52(6):1213–1246. https://doi.org/10.1016/j.jmps.2003.12.007
Evers LP, Brekelmans WAM, Geers MGD (2004) Non-local crystal plasticity model with intrinsic SSD and GND effects. J Mech Phys Solid 52(10):2379–2401. https://doi.org/10.1016/j.jmps.2004.03.007
Xue X, Tong DM, Shen G (2021) Micromechanical behavior simulation of dual-phase steel considering non-uniform distribution of geometric essential dislocation. J Mater Heat Treat 8:153–162. https://doi.org/10.13289/j.issn.1009-6264.2021-0086. (In Chinese)
Wang Z, Sun T, Zhang H (2019) The interaction between grain boundary and tool geometry in nanocutting of a bi-crystal copper. Int J Extreme Manuf 1(4):045001. https://doi.org/10.1088/2631-7990/ab4b68
Wang QL, Zhang CF, Wu MP, Chen JX (2019) Mechanism of subsurface damage in single point diamond cutting of nano polycrystalline copper. China Mech Eng 30(23):2790–2797. https://doi.org/10.3969/j.issn.1004-132X.2019.23.004. (In Chinese)
Bai J, Bai Q, Hu C (2016) Evolution of surface grain structure and mechanical properties in orthogonal cutting of titanium alloy. J Mater Res 31(24):3919–3929. https://doi.org/10.1557/jmr.2016.444
Bai J, Bai Q, Zhen T (2018) Theoretical model for subsurface microstructure prediction in micro-machining Ti-6Al-4V alloy - experimental validation. Int J Mech Sci 148:64–72. https://doi.org/10.1016/j.ijmecsci.2018.08.014
Ding H, Shen N, Shin YC (2011) Modeling of grain refinement in aluminum and copper subjected to cutting. Comp Mater Sci 50(10):3016–3025. https://doi.org/10.1016/j.commatsci.2011.05.020
Bla B, Song Z, Rha B (2020) Dislocation density and grain size evolution in hard machining of H13 steel: numerical and experimental investigation. J Mater Res Technol 9(3):4241–4254. https://doi.org/10.1016/j.jmrt.2020.02.051
Manual Editorial Board (2010) Materials data manual for aeroengine design. Aviation Industry Press (In Chinese)
Peng MW, Gu LY (2021) Finite element analysis of polycrystalline metal cutting based on Voronoi model. J Tool Technol 55:48–54. https://doi.org/10.3969/j.issn.1000-7008.2021.05.009. (In Chinese)
Fu HH, Benson DJ, Meyers MA (2001) Analytical and computational description of effect of grain size on yield stress of metals. Acta Mater 49(13):2567–2582. https://doi.org/10.1016/S1359-6454(01)00062-3
Johnson GR, Cook WH (1983) A constitutive model and data for metals subjected to large strains, high strain rates and high temperatures. Eng Fract Mech 21:541–548
Zhang H, Dong X, Du D (2013) A unified physically based crystal plasticity model for FCC metals over a wide range of temperatures and strain rate. Mater Sci Eng 564:431–441. https://doi.org/10.1016/j.msea.2012.12.001
Bardella L (2006) A deformation theory of strain gradient crystal plasticity that accounts for geometrically necessary dislocations. J Mech Phys Solids 54(1):128–160. https://doi.org/10.1016/j.jmps.2005.08.003
Taylor GI (1934) The mechanism of plastic deformation of crystals. Part I. Theoretical. P Roy Soc Lon 145(855):362–387. https://doi.org/10.2307/2935509
Ashby MF (1966) Work hardening of dispersion-hardened crystals. Phil Mag 14(132):1157–1178. https://doi.org/10.1080/14786436608224282
Argon A (2007) Strengthening mechanisms in crystal plasticity. Oxford University Press, London
Lu M, Zhou J, Zhu R (2009) Effects of strain gradient on the mechanical behaviors of nanocrystalline materials. Mater Sci Eng A 507(1–2):42–49. https://doi.org/10.1016/j.msea.2008.11.055
Huo RX, Zou JQ, Li XB (2010) Constitutive equation of nanocrystalline materials based on phase. J Nanjing Univer Technol: Nat Sci Ed 32(4):59–62. https://doi.org/10.3969/j.issn.1671-7627.2010.04.012. (In Chinese)
Erice B, Gálvez F (2014) A coupled elastoplastic-damage constitutive model with Lode angle dependent failure criterion. Int J Solids Struct 51(1):93–110. https://doi.org/10.1016/j.ijsolstr.2013.09.015
Zorev NN (1963) Inter-relationship between shear processes occurring along tool face and shear plane in metal cutting. Int Res Prod Eng 49:143–152
Pan XX (2005) Study on dynamic characteristics of FeCrNi alloy. Sichuan University. https://doi.org/10.7666/d.y779142 (In Chinese)
Liu P (2010) Dislocation dynamics analysis during plastic deformation. Hefei Polytechnic University. https://doi.org/10.7666/d.y1700721 (In Chinese)
Jin YH, Wang XJ, Li CH (2010) Microstructure evolution of friction stir welded joint of aluminum alloy 7050. Hot Work Process 39(15):122–124. https://doi.org/10.3969/j.issn.1001-3814.2010.15.039. (In Chinese)
Wang Y, Shao WZ, Zhen L, Yang L, Zhang X (2008) Flow behavior and microstructures of superalloy 718 during high temperature deformation. Mater Sci Eng A 497:479–486. https://doi.org/10.1016/j.msea.2008.07.046
Duan CZ, Zhang LC (2012) Adiabatic shear banding in AISI 1045 steel during high speed machining: mechanisms of microstructural evolution. Mater Sci Eng 532:111–119. https://doi.org/10.1016/j.msea.2011.10.071
Giovanola JH (1988) Adiabatic shear banding under pure shear loading Part II: fractographic and metallographic observations. Mech Mater 7(1):73–87. https://doi.org/10.1016/0167-6636(88)90007-5
Wang GM, Fan JM (1991) Dislocation theory and its application in metal cutting. J Kunming Inst Technol 16(5):5 (In Chinese)
Meyers MA, Pak HR (1986) Observation of an adiabatic shear band in titanium by high-voltage transmission electron microscopy. Acta Metall 34(12):2493–2499. https://doi.org/10.1016/0001-6160(86)90152-5
Funding
This work is supported by the National Natural Science Foundation of China (52275404), Natural Science Foundation of Jilin Province (YDZJ202301ZZYTS484), Project of Science and Technology Bureau of Changchun City, Jilin Province (21ZY40).
Author information
Authors and Affiliations
Contributions
Yihang Fan: conceptualization, methodology, and writing—review and editing.
Bing Wang: formal analysis, data curation, and writing—original draft.
LiJia Li: methodology.
LingHao Kong: data curation.
Zhaopeng Hao: conceptualization, methodology, project administration, funding acquisition, and resources.
Gangwei Cui: data curation.
Corresponding authors
Ethics declarations
Ethics approval
This article does not contain any studies with human participants or animals performed by any of the authors.
Consent to participate
The authors gave their approval in participating in this study.
Consent for publication
The authors approve of the publication of this study.
Conflict of interest
The authors declare no competing interests.
Additional information
Publisher's note
Springer Nature remains neutral with regard to jurisdictional claims in published maps and institutional affiliations.
Rights and permissions
Springer Nature or its licensor (e.g. a society or other partner) holds exclusive rights to this article under a publishing agreement with the author(s) or other rightsholder(s); author self-archiving of the accepted manuscript version of this article is solely governed by the terms of such publishing agreement and applicable law.
About this article
Cite this article
Fan, Y., Wang, B., Hao, Z. et al. A GND simulation model for micro-deformation mechanism analyses in high-speed cutting Inconel718. Int J Adv Manuf Technol 128, 2931–2952 (2023). https://doi.org/10.1007/s00170-023-12026-4
Received:
Accepted:
Published:
Issue Date:
DOI: https://doi.org/10.1007/s00170-023-12026-4