Abstract
Growing interest in possible global climate change has underlined the need for better information concerning the way in which carbon partitioning between ecosystem components is influenced by constraints on nutrient availability. Micro-organisms play a fundamental role in the cycling of carbon and nutrients in all ecosystems but the role of fungi in particular is pivotal in boreal forest ecosystems. Traditional models of nutrient cycling are based on methods and concepts developed in agricultural systems where microorganisms are considered primarily as nutrient processors providing plants with inorganic nutrients. The filamentous nature of fungi, their ability to translocate carbon and nutrients between different substrates and the capacity of ectomycorrhizal fungi to utilise organic nutrients have all been largely ignored. In this article, a new model is suggested which emphasises competition for organic nutrients between decomposer organisms and plants, with the plants depending on their associated mycorrhizal fungi for nutrient acquisition. Antagonistic interactions involving nutrient transfer between decomposer and mycorrhizal fungi are proposed as important pathways in nutrient cycling. Due to the nutrient conservative features of decomposer fungi, inorganic nutrients are considered less important for plant nutrition. The implications of the new nutrient cycling model on the carbon balance of boreal forests are discussed.
Similar content being viewed by others
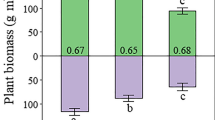
References
Aber J D and Melillo J M 1980 Litter decomposition: Measuring relative contributions of organic matter and nitrogen to forest soils. Can. J. Bot. 58, 416-421.
Aber J D and Melillo J M 1991 Terrestrial ecosystems. Saunders College Publishing, New York.
Abuzinadah R A, Finlay R D and Read D J 1986 The role of proteins in the nitrogen nutrition of ectomycorrhizal plants: II. Utilization of protein by mycorrhizal plants of Pinus contorta. New Phytol. 103, 495-506.
Abuzinadah R A and Read D J 1986 The role of proteins in the nitrogen nutrition of ectomycorrhizal plants: III. Protein utilization by Betula, Picea and Pinus in mycorrhizal association with Hebeloma crustuliniforme. New Phytol. 103, 507-514.
Abuzinadah R A and Read D J 1989 The role of proteins in the nitrogen nutrition of ectomycorrhizal plants: IV. The utilization of peptides by birch (Betula pendula L.) infected with different mycorrhizal fungi. New Phytol. 112, 55-66.
Bending G D and Read D J 1995a The structure and function of the vegetative mycelium of ectomycorrhizal plants: VI. Activities of nutrient mobilizing enzymes in birch litter colonized by Paxillus involutus (Fr.) Fr. New Phytol. 130, 411-417.
Bending G D and Read D J 1995b The structure and function of the vegetative mycelium of ectomycorrhizal plants: V. Foraging behaviour and translocation of nutrients from exploited litter. New Phytol. 130, 401-409.
Bending G D and Read D J 1996 Nitrogen mobilization from protein-polyphenol complex by ericoid and ectomycorrhizal fungi. Soil Biol. Biochem. 28, 1603-1612.
Bending G D and Read D J 1997 Lignin and soluble phenolic degradation by ectomycorrhizal and ericoid mycorrhizal fungi. Mycol. Res. 101, 1348-1354.
Berg B 2000 Litter decomposition and organic matter turnover in northern forest soils. For. Ecol. Manage. 133, 13-22.
Berg B, Ekbohm G, Johansson M B, McClaugherty C, Rutigliano F and Virzo De Santo A 1996 Maximum decomposition limits of forest litter types: A synthesis. Can. J. Bot. 74, 659-672.
Berg B, Hannus K, Popoff T and Theander O 1982 Changes in organic chemical components of needle litter during decomposition. Long-term decomposition in a Scots pine forest I. Can. J. Bot. 60, 1310-1319.
Berg B, Staaf H and Wessen B 1987 Decomposition and nutrient release in needle litter from nitrogen-fertilized Scots pine (Pinus sylvestris) stands. Scand. J. For. Res. 2, 399-415.
Berg B and Theander O 1984 Dynamics of some nitrogen fractions in decomposing Scots pine needle litter. Pedobiologia 27, 261-267.
Bergh J, Linder S, Lundmark T and Elfving B 1999 The effect of water and nutrient availability on the productivity of Norway spruce in northern and southern Sweden. For. Ecol. Manage. 119, 51-62.
Boddy L 1999 Saprotrophic cord-forming fungi: Meeting the challenge of heterogeneous environments. Mycologia 91, 13-32.
Boddy L and Watkinson S C 1995 Wood decomposition, higher fungi, and their role in nutrient redistribution. Can. J. Bot. 73, suppl. 1, sect. E-H, 1377-1383.
Bååth E and Söderström B 1979 Fungal biomass and fungal immobilization of plant nutrients in Swedish coniferous forest soils. Rev. Ecol. Biol. Sol 16, 477-489.
Cairney J W G 1992 Translocation of solutes in ectomycorrhizal and saprotrophic rhizomorphs. Mycol. Res. 96, 135-141.
Cairney J W G and Burke R M 1994 Fungal enzymes degrading plant cell walls: Their possible significance in the ectomycorrhizal symbiosis. Mycol. Res. 98, 1345-1356.
Cairney J W G and Burke R M 1998 Do ecto-and ericoid mycorrhizal fungi produce peroxidase activity? Mycorrhiza 8, 61-65.
Chambers S M, Burke R M, Brooks P R and Cainey J W G 1999 Molecular and biochemical evidence for manganese-dependent peroxidase activity in Tylospora fibrillosa. Mycol. Res. 103, 1098-1102.
Colpaert J V, Van Laere A and Van Assche J A 1996 Carbon and nitrogen allocation in ectomycorrhizal and non-mycorrhizal Pinus sylvestris L. seedlings. Tree Physiol. 16, 787-793.
Colpaert J V and van Tichelen K K 1996 Decomposition, nitrogen and phosphorus mineralization from beech leaf litter colonized by ectomycorrhizal or litter decomposing basidiomycetes. New Phytol. 134, 123-132.
Colpaert J V and Verstuyft I 1999 The Ingestad concept in ectomycorrhizal research: Possibilities and limitations. Physiol. Plant. 105, 233-238.
Cotrufo M F and Ineson P 1996 Elevated CO-2 reduces field decomposition rates of Betula pendula (Roth.) leaf litter. Oecologia 106, 525-530.
Cotrufo M F, Ineson P and Roberts J D 1995 Decomposition of birch leaf litters with varying C-to-N ratios. Soil Biol. Biochem. 27, 1219-1221.
DeLuca T H, Keeney D R and McCarty G W 1992 Effect of freeze-thaw events on mineralization of soil nitrogen. Biol. Fert. Soils 14, 116-120.
Dyck W J, Mees C A and Hodgkiss P D 1987 Nitrogen availability and comparison to uptake in two New Zealand Pinus radiata forests. N. Z. J. For. Sci. 17, 338-352.
Fahey T J 1983 Nutrient dynamics of aboveground detritus in lodgepole pine (Pinus contorta ssp.latifolia) ecosystems, southeastern Wyoming. Ecol. Monogr. 53, 51-72.
Fahey T J, Yavitt J B, Pearson J A and Knight D H 1985 The nitrogen cycle in lodgepole pine forests, southeastern Wyoming. Biogeochemistry 1, 257-275.
Finlay R and Söderström B 1992 Mycorrhiza and carbon flow to the soil. In Mycorrhizal Functioning. Ed. M F Allen. pp 134-160. Chapman & Hall, New York.
Finlay R D, Frostegård Å and Sonnerfeldt A-M 1992 Utilization of organic and inorganic nitrogen sources by ectomycorrhizal fungi in pure culture and in symbiosis with Pinus contorta Dougl. ex Loud. New Phytol. 120, 105-115.
Fitter A H, Heinemeyer A and Staddon P L 2000 The impact of elevated CO2 and global climate change on arbuscular mycorrhizas: A mycocentric approach. New Phytol. 147, 179-187.
Frostegård Å and Bååth E 1996 The use of phospholipid fatty acid analysis to estimate bacterial and fungal biomass in soil. Biol. Fert. Soils 22, 59-65.
Gadgil R L and Gadgil P D 1971 Mycorrhiza and litter decomposition. Nature 233, 133.
Giltrap N J 1982 Production of polyphenol oxidases by ectomycorrhizal fungi with special reference to Lactarius spp. Trans. Br. Mycol. Soc. 78, 75-81.
Gorissen A and Kuyper T H W 2000 Fungal species-specific responses of ectomycorrhizal Scots pine (Pinus sylvestris) to elevated [CO2]. New Phytol. 146, 163-168.
Goulden M L, Wofsy S C, Harden J W, Trumbore S E, Crill P M, Gower S T, Fries T, Daube B C, Fan S M, Sutton D J, Bazzaz A and Munger J W 1998 Sensitivity of boreal forest carbon balance to soil thaw. Science 279, 214-217.
Handley W R C 1961 Further evidence for the importance of residual leaf protein complexes in litter decomposition and the supply of nitrogen for plant growth. Plant Soil 15, 37-73.
Hart S C and Firestone M K 1991 Forest floor-mineral soil interactions in the internal nitrogen cycle of an old-growth forest. Biogeochemistry 12, 103-128.
Haselwandter K, Bobleter O and Read D J 1990 Degradation of 14C-labelled lignin and dehydropolymer of coniferyl alcohol by ericoid and ectomycorrhizal fungi. Arch. Microbiol. 153, 352-354.
Hodge A, Alexander I J and Gooday G W 1995 Chitinolytic enzymes of pathogenic and ectomycorrhizal fungi. Mycol. Res. 99, 935-941.
Hodge A, Robinson D and Fitter A H 2000 An arbuscular mycorrhizal inoculum enhances root proliferation in, but not nitrogen capture from, nutrient-rich patches in soil. New Phytol. 145, 575-584.
Hutchison L J 1990 Studies on the systematics of ectomycorrhizal fungi in axenic culture: III. Patterns of polyphenol oxidase activity. Mycologia 82, 424-435.
Jalal M A F, Read D J and Haslam E 1982 Phenolic composition and its seasonal variation in Calluna vulagris. Phytochemistry 21, 1397-1401.
Jonasson S, Michelsen A, Schmidt I K, Nielsen E V and Callaghan T V 1996 Microbial biomass C, N and P in two arctic soils and responses to addition of NPK fertilizer and sugar: Implications for plant nutrient uptake. Oecologia 106, 507-515.
Kaye J P and Hart S C 1997 Competition for nitrogen between plants and soil micro-organisms. Trends Ecol. Evol. 12, 139-143.
Kelley K R and Stevenson F J 1996 Organic forms of N in soil. In Humic Substances in Terrestrial Ecosystems. Ed. A Piccolo. pp 407-427. Elsevier Science, Amsterdam.
Kerley S J and Read D J 1995 The biology of mycorrhiza in the Ericaceae: XVIII. Chitin degradation by Hymenoscyphus ericae and transfer of chitin-nitrogen to the host plant. New Phytol. 131, 369-375.
Kerley S J and Read D J 1997 The biology of mycorrhiza in the Ericaceae: XIX. Fungal mycelium as a nitrogen source for the ericoid mycorrhizal fungus Hymenoscyphus ericae and its host plants. New Phytol. 136, 691-701.
Kerley S J and Read D J 1998 The biology of mycorrhiza in the Ericaceae: XX. Plant and mycorrhizal necromass as nitrogenous substrates for the ericoid mycorrhizal fungus Hymenoscyphus ericae and its host. New Phytol. 139, 353-360.
Keyser P, Kirk T K and Zeikus I G 1978 Ligninolytic enzyme of Phanerochaete chrysosporium: Synthesized in the absence of lignin in response to nitrogen starvation. J. Bacteriol. 135, 790-797.
Knight D H, Yavitt J B and Joyce G D 1991 Water and nitrogen out-flow from lodgepole pine forest after two levels of tree mortality. For. Ecol. Manage. 46, 215-226.
Kope H H and Fortin J A 1989 Inhibition of phytopathogenic fungi in vitro by cell free culture media of ectomycorrhizal fungi. New Phytol. 113, 57-63.
Laiho R and Prescott C E 1999 The contribution of coarse woody debris to carbon, nitrogen and phosphorus cycles in three Rocky Mountain coniferous forests. Can. J. Forest Res. 29, 1592-1603.
Leake J R and Read D J 1997 Mycorrhizal fungi in terrestrial habitats. In The Mycota IV: Environmental and Microbial Relationships. Eds. D T Wicklow and B Söderström. pp 281-301. Springer-Verlag, Berlin.
Lindahl B, Stenlid J, Olsson S and Finlay R 1999 Translocation of 32P between interacting mycelia of a wood decomposing fungus and ectomycorrhizal fungi in microcosm systems. New Phytol. 144, 183-193.
Lindahl B, Stenlid J, Olsson S and Finlay R 2001 Effects of resource availability on mycelial interactions and 32P transfer between a saprotrophic and an ectomycorrhizal fungus in soil microcosms. FEMS Microbiol. Ecol. 38, 43-52.
Lund V and Goksøyr J 1980 Effects of water fluctuations on microbial biomass in soil. Microbiol. Ecol. 6, 115-123.
Majdi H and Kangas P 1997 Demography of fine roots in response to nutrient applications in a Norway spruce stand in southwestern Sweden. Ecoscience 4, 199-205.
Markkola A M, Ohtonen R, Tarvainen O and Ahonen-Jonnarth U 1995 Estimates of fungal biomass in scots pine stands on an urban pollution gradient. New Phytol. 131, 139-147.
Marumoto T, Anderson J P E and Domsch K H 1982 Mineralization of nutrients from soil microbial biomass. Soil Biol. Biochem. 14, 469-475.
Merrill W and Cowling E B 1966 Role of nitrogen in wood deterioration. IV. Relationship of natural variation in nitrogen content of wood to its susceptibility to decay. Phytopathology 56, 1324-1325.
Moore T R 1996 The carbon budget of boreal forests: Reducing the uncertainty. In Global Chance: Effects on Coniferous Forest and Grasslands. Eds. A I Breymeyer, D O Hall, J M Melillo and G I Ågren. pp 17-40. John Wiley & Sons Ltd., Chichester.
Nadelhoffer K J, Downs M R and Fry B 1999 Sinks for 15N-enriched additions to an oak forest and a red pine plantation. Ecol. Appl. 9, 72-86.
Northup R R, Yu Z, Dahlgren R A and Vogt K A 1995 Polyphenol control of nitrogen release from pine litter. Nature 377, 227-229.
Näsholm T, Ekblad A, Nordin A, Giesler R, Högberg M and Högberg P 1998 Boreal forest plants take up organic nitrogen. Nature 392, 914-916.
Olsson S 1995 Mycelial density profiles of fungi on heterogenous media and their interpretation in terms of nutrient reallocation patterns. Mycol. Res. 99, 143-153.
Pastor J, Aber J D, McClaugherty C A and Melillo J M 1984 Aboveground production and N and P cycling along a nitrogen mineralization gradient on Blackhawk Island, Wisconsin. Ecology 65, 256-268.
Perez-Moreno J and Read D J 2000 Mobilization and transfer of nutrients from litter to tree seedlings via the vegetative mycelium of ectomycorrhizal plants. New Phytol. 145, 301-309.
Persson T 1989 Role of soil animals in C and N mineralisation. Plant Soil 115, 241-245.
Persson T, Van Oene H, Harrison A F, Karlsson P, Bauer G, Cerny J, Coûteaux M-M, Dambrine E, Högberg P, Kjøller A, Matteucci G, Rudebeck A, Schulze E-D and Paces T 2000 Experimental sites in the NIPHYS/CANIF project. In Carbon and Nitrogen Cycling in European forest ecosystems. Ecological Studies Series. Ed. E-D Schulze. Vol. 142, pp 14-46. Springer Verlag, Heidelberg.
Preston C M and Mead D J 1994 Growth response and recovery of 15N-fertilizer one and eight growing seasons after application to lodgepole pine in British Columbia. For. Ecol. Manage. 65, 219-229.
Pulleman M and Tietema A 1999 Microbial C and N transformations during drying and rewetting of coniferous forest floor material. Soil Biol. Biochem. 31, 275-285.
Qualls R G and Haines B L 1991 Fluxes of dissolved organic nutrients and humic substances in a deciduous forest. Ecology 72, 254-266.
Rayner A D M and Boddy L 1988 Fungal decomposition of wood, its biology and ecology. John Wiley & Sons Ltd., Chichester.
Read D J 1984 The structure and function of the vegetative mycelium of mycorrhizal roots. In The Ecology and Physiology of the Fungal Mycelium. Symposium of the British Mycological Society. Eds. D H Jennings and A D M Rayner. pp 215-240. Cambridge University Press, Cambridge.
Read D J 1991 Mycorrhizas in ecosystems. Experientia 47, 376-391.
Rouhier H and Read D J 1998 Plant and fungal responses to elevated atmospheric carbon dioxide in mycorrhizal seedlings of Pinus sylvestris. Environ. Exp. Bot. 40, 237-246.
Rouhier H and Read D J 1999 Plant and fungal responses to elevated atmospheric CO2 in mycorrhizal seedlings of Betula pendula. Environ. Exp. Bot. 42, 231-241.
Rygiewicz P T and Andersen C P 1994 Mycorrhizae alter quality and quantity of carbon allocated below ground. Nature 369, 58-60.
Schleppi P, Bucher-Wallin L, Siegwolf R, Saurer M, Muller N and Bucher J B 1999 Simulation of increased nitrogen deposition to a montane forest ecosystem: Partitioning of the added 15N. Wat. Air Soil Pollut. 116, 129-134.
Smith S E and Read D J 1997 Mycorrhizal symbiosis. Academic Press Inc., San Diego.
Staaf H and Berg B 1982 Accumulation and release of plant nutrients in decomposing Scots pine needle litter. Long term decomposition in a Scots pine forest II. Can. J. Bot. 60, 1561-1568.
Stanford G and Smith S J 1972 Nitrogen mineralization potentials of soils. Soil Sci. Soc. Am. Proc. 36, 465-472.
Swift M J, Heal O W and Anderson M 1979 Decomposition in terrestrial ecosystem. Blackwell Scientific Publications, London.
Tanesaka E, Masuda H and Kinugawa K 1993 Wood degrading ability of basidiomycetes that are wood decomposers, litter decomposers, or mycorrhizal symbionts. Mycologia 85, 347-354.
Taylor A F S, Högbom L, Högberg M, Lyon A J E, Näsholm T and Högberg P 1997 Natural 15N abundance in fruit bodies of ectomycorrhizal fungi from boreal forests. New Phytol. 136, 713-720.
Taylor A F S, Martin F and Read D J 2000 Fungal diversity in ectomycorrhizal communities of Norway spruce (Picea abies (L.) Karst.) and Beech (Fagus sylvatica L.) along north-south transects in Europe. In Carbon and Nitrogen Cycling in European Forest Ecosystems. Ecological Studies Series. Ed. E-D Schulze. Vol. 142, pp 343-365. Springer Verlag, Heidelberg.
Tietema A, Emmet B A, Gundersen P, Kjønaas O J and Koopmans C J 1998 The fate of 15N-labelled nitrogen deposition in coniferous forest ecosystems. For. Ecol. Manage. 101, 19-27.
Trojanowski J, Haider H and Hüttermann A 1984 Decomposition of 14C-labelled lignin, holocellulose and lignocellulose by mycorrhizal fungi. Arch. Microbiol. 139, 202-206.
Van Breemen N, Finlay R, Lundström U, Jongmans A G, Giesler R and Olsson M 2000 Mycorrhizal weathering: A true case of mineral plant nutrition ? Biogeochemistry 49, 53-67.
Vitousek P M and Matson P A 1984 Mechanisms of nitrogen retention in forest ecosystems: A field experiment. Science 225, 51-52.
Vogt K A and Edmonds R L 1980 Patterns of nutrient concentration in basidiocarps in western Washington. Can. J. Bot. 58, 694-698.
Wells J, Harris M and Boddy L 1998 Temporary phosphorus partitioning in mycelial systems of the cord-forming basidiomycete Phanerochaete velutina. New Phytol. 140, 283-293.
Wells J M and Boddy L 1995 Phosphorus translocation by saprotrophic basidiomycete mycelial cord systems on the floor of a mixed deciduous woodland. Mycol. Res. 99, 977-980.
Wells J M, Hughes C and Boddy L 1990 The fate of soil-derived phosphorus in mycelial cord systems of Phanerochaete velutina and Phallus impudicus. New Phytol. 114, 595-606.
Yavitt J B and Fahey T J 1984 An experimental analysis of soil solution chemistry in a lodgepole pine forest floor. Oikos 43, 222-234.
Yavitt J B and Fahey T J 1986 Litter decay and leaching from the forest floor in Pinus contorta (Lodgepole pine) ecosystems. J. Ecol. 74, 525-545.
Author information
Authors and Affiliations
Corresponding author
Rights and permissions
About this article
Cite this article
Lindahl, B.O., Taylor, A.F.S. & Finlay, R.D. Defining nutritional constraints on carbon cycling in boreal forests – towards a less `phytocentric' perspective. Plant and Soil 242, 123–135 (2002). https://doi.org/10.1023/A:1019650226585
Issue Date:
DOI: https://doi.org/10.1023/A:1019650226585