Abstract
The realization of an ideal combination of mechanical and fatigue properties is prerequisites for practical application of titanium (Ti) microalloyed steel in automotive field. The fatigue behavior of four Ti microalloyed high-strength beam steels with different Ti contents was systematically studied. The results show that the content of microalloying element Ti has a significant effect on the fatigue properties, especially in the steel with a high Ti content. For the experimental Ti microalloyed steel, inclusion-induced crack initiation is the main fatigue failure mode. Different from general fatigue fracture mechanism in Ti-contained steel, no TiN, which is the most detrimental to fatigue behavior, was found in fatigue crack initiation area. However, the large-sized TiN and oxide complex inclusion with a core–shell structure is the dominant cause of fatigue fracture. Because of the intense-localized deformation at the interface between complex inclusion and matrix, the angular TiN in the outer shell has a serious deteriorating effect on the fatigue properties, which is consistent with the result of the Kernel average misorientation map. Besides, the modification effect of a small amount of MnS on large-sized inclusion is not obvious and has little effect on the fatigue behavior. For more practical guidance, the critical inclusion sizes of the experimental steels were also investigated by experimental extrapolation method. With the increasing tensile strength, the inclusion sensitivity of the experimental steels increases, leading to the small critical inclusion size.












Similar content being viewed by others
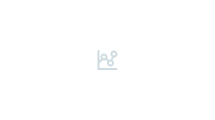
References
P. Wang, M. Ryberg, Y. Yang, Nat. Commun. 12 (2021) 2066.
P. Wolfram, S. Weber, K. Gillingham, Nat. Commun. 12 (2021) 7121.
R. Liu, P. Zhang, Z.J. Zhang, B. Wang, Z.F. Zhang, J. Mater. Sci. Technol. 70 (2021) 233–249.
R. Liu, P. Zhang, Z.J. Zhang, B. Wang, Z.F. Zhang, J. Mater. Sci. Technol. 70 (2021) 250–267.
S. Wang, Z. Gao, G. Wu, X. Mao, Int. J. Miner. Metall. Mater. 29 (2022) 645–661.
Y. Murakami, High and ultrahigh cycle fatigue, Elsevier, Oxford, UK, 2003.
S. Nishida, Failure analysis in engineering applications, Butterworth Heinemann, Oxford, UK, 1992.
U. Zerbst, M. Madia, C. Klinger, D. Bettge, Y. Murakami, Eng. Fail. Anal. 97 (2019) 777–792.
U. Zerbst, M. Madia, C. Klinger, D. Bettge, Y. Murakami, Eng. Fail. Anal. 98 (2019) 228–239.
M.L. Zhu, L. Jin, F.Z. Xuan, Acta Mater. 157 (2018) 259–275.
W. Hui, S. Chen, Y. Zhang, C. Shao, H. Dong, Mater. Des. 66 (2015) 227–234.
T.N. Baker, Ironmak. Steelmak. 43 (2016) 264–307.
P. Gong, X.G. Liu, A. Rijkenberg, W.M. Rainforth, Acta Mater. 161 (2018) 374–387.
Y. Furuya, S. Matsuoka, Metall. Mater. Trans. A 33 (2002) 3421–3431.
P. Wang, B. Wang, Y. Liu, P. Zhang, Y.K. Luan, D.Z. Li, Z.F. Zhang, Scripta Mater. 206 (2022) 114232.
Y. Murakami, Effects of small defects and nonmetallic inclusions, Elsevier, Oxford, UK, 2002.
Y. Murakami, Metal fatigue: effects of small defects and nonmetallic inclusions, Elsevier, Oxford, UK, 2019.
Y. Zhenguo, Acta Metall. Sin. 41 (2005) 1136–1142.
J. Zhang, S. Li, Z. Yang, G. Li, W. Hui, Y. Weng, Int. J. Fatigue 29 (2007) 765–771.
Z.G. Yang, G. Yao, G.Y. Li, S.X. Li, Z.M. Chu, W.J. Hui, H. Dong, Y.Q. Weng, Int. J. Fatigue 26 (2004) 959–966.
L. Tang, C. Wu, Z. Zhang, J. Shang, C. Yan, Metals 6 (2016) 280.
P. Zhao, G. Gao, R.D.K. Misra, B. Bai, Mater. Sci. Eng. A 630 (2015) 1–7.
Q. Zhang, Y. Zhu, X. Gao, Y. Wu, C. Hutchinson, Nat. Commun. 11 (2020) 5198.
G. Gao, Q. Xu, H. Guo, X. Gui, B. Zhang, B. Bai, Mater. Sci. Eng. A 739 (2019) 404–414.
M.D. Sangid, Int. J. Fatigue 57 (2013) 58–72.
C. Gu, W.Q. Liu, J.H. Lian, Y.P. Bao, Int. J. Miner. Metall. Mater. 28 (2021) 826–834.
X. Gui, G. Gao, B. An, R.D.K. Misra, B. Bai, Mater. Sci. Eng. A 803 (2021) 140692.
Z. Cao, Z. Shi, F. Yu, G. Wu, W. Cao, Y. Weng, Int. J. Fatigue 126 (2019) 1–5.
C. Gu, Y.P. Bao, P. Gan, M. Wang, J.S. He, Int. J. Miner. Metall. Mater. 25 (2018) 623–629.
J. Tan, X. Wu, E.H. Han, W. Ke, X. Liu, F. Meng, X. Xu, Corros. Sci. 88 (2014) 349–359.
T. Gao, Z. Sun, H. Xue, D. Retraint, Int. J. Fatigue 139 (2020) 105798.
C. Yang, P. Liu, Y. Luan, D. Li, Y. Li, Int. J. Fatigue 128 (2019) 105193.
J. Schumacher, B. Clausen, Steel Res. Int. 92 (2021) 2100252.
G. Gao, R. Liu, K. Wang, X. Gui, R.D.K. Misra, B. Bai, Scripta Mater. 184 (2020) 12–18.
L. Zhao, X. Qi, L. Xu, Y. Han, H. Jing, K. Song, Fatigue Fract. Eng. M 44 (2021) 533–550.
Acknowledgements
This work was financially supported by the National Natural Science Foundation of China (No. 52104369) and the China Postdoctoral Science Foundation (No. 2021M700374).
Author information
Authors and Affiliations
Corresponding authors
Ethics declarations
Conflict of interest
The authors declare that they have no known competing financial interests or personal relationships that could have appeared to influence the work reported in this paper.
Rights and permissions
Springer Nature or its licensor (e.g. a society or other partner) holds exclusive rights to this article under a publishing agreement with the author(s) or other rightsholder(s); author self-archiving of the accepted manuscript version of this article is solely governed by the terms of such publishing agreement and applicable law.
About this article
Cite this article
Gao, Zj., Pan, Gf., Song, Y. et al. High cycle fatigue behavior of titanium microalloyed high-strength beam steels. J. Iron Steel Res. Int. 30, 2267–2279 (2023). https://doi.org/10.1007/s42243-023-00963-z
Received:
Revised:
Accepted:
Published:
Issue Date:
DOI: https://doi.org/10.1007/s42243-023-00963-z