Abstract
The repetitive modelling required for traditional structural design work is a laborious and time-consuming task. Yet, the cost significantly increases due to the wide variety of profiles. It is suggested to use grouping technique along with algorithms to optimize structural elements in order to address these issues. In this paper, two meta-heuristic algorithms viz; Cuckoo Search algorithm and Thermal Exchange Optimization algorithm are employed for weight optimization of FPSO supporting structure. These algorithms identify the structure’s least weight for the offshore floating platform design. The current work suggests a method for FPSO structure design that is reliability-based and optimal. The current study discusses the optimized design of the topside FPSO structural assembly for various load conditions, such as production tank pressure loads, equipment loads, piping loads, electrical and instrumentation loads, girder deformation loads, wind load, and so on. The effects of hull flexibility, as well as wind and motion-induced accelerations, are considered for both Normal Operating and Extreme Operating conditions. The analysis and design optimization are carried out in the STAAD Pro and analytical tool by selecting the least weight as an objective function while adhering to the API design constraints on displacement and stress limits. Module primary structure design can be iteratively “optimized” using the algorithm and stress ratio as described above. By implication, the algorithms, FPSO supporting structure translates into optimal design, resulting in cost savings.













Similar content being viewed by others
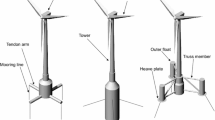
Data availability
The dataset generated and analyzed during the current study are available from the corresponding author on reasonable request.
References
0027/ND-Noble Denton Guidelines for Marine Lifting Operations, March 2010.
AISC-ASD. (2023). Specification for the Design, Fabrication and Erection of Structural Steel for Buildings—Allowable Stress Design, 9th Edition.
AlHamaydeh, M., Barakat, S., & Nasif, O. (2017). Optimization of support structures for offshore wind turbines using genetic algorithm with domain-trimming. Mathematical Problems in Engineering., 2017, 1–14.
API RP 2A-WSD. (2000). Recommended practice for planning, designing and Constructing Fixed Offshore Platforms—Working Stress Design, 21st Edition.
Aydın, Z. (2022). Size, layout and tendon profile optimization of prestressed steel trusses using Jaya algorithm. Structures, 40, 284–294.
Azizi, M., Ejlali, R. G., Mousavi Ghasemi, S. A., & Talatahari, S. (2019). Upgraded Whale Optimization Algorithm for fuzzy logic based vibration control of nonlinear steel structure. Engineering Structures, 192, 53–70.
Babaei, M., & Moharrami, H. (2022). Geometry and sizing optimization of steel gable frames with tapered members. Structures, 42, 575–585.
Benzo, P. G., Pereira, J. M., & Sena-Cruz, J. (2022). Optimization of steel web core sandwich panel with genetic algorithm. Engineering Structures, 253, 113805.
Chew, K., Tai, K., Ng, E., & Muskulus, M. (2015). Optimization of offshore wind turbine support structures using an analytical gradient-based method. Energy Procedia, 80, 100–107.
Cicconi, P., Germani, M., Bondi, S., Zuliani, A., & Cagnacci, E. (2016). A design methodology to support the optimization of steel structures. Procedia CIRP, 50, 58–64.
Fang, C., Ping, Y., Gao, Y., Zheng, Y., & Chen, Y. (2022). Machine learning-aided multi-objective optimization of structures with hybrid braces—framework and case study. Engineering Structures, 269, 114808.
Fang, H., Iqbal, N., Van Staen, G., & De Backer, H. (2021). Structural optimization of rib-to-crossbeam joint in orthotropic steel decks. Engineering Structures, 248, 113208.
FPSO Topside Structural Design Basis guidelines API standard 2000.
Fu, J., Wu, J., Dong, C., Xu, A., & Pi, Y. (2019). Optimization design of large span portal-rigid steel frame with tapered sections under wind-induced drift constraint. Engineering Structures, 194, 396–405.
Ghadimi, B., & Taghikhany, T. (2021). Dynamic response assessment of an offshore jacket platform with semi-active fuzzy-based controller: A case study. Ocean Engineering, 238, 109747.
Gholizadeh, S., Hasançebi, O., Eser, H., & Koçkaya, O. (2022). Seismic collapse safety based optimization of steel Moment-Resisting frames. Structures, 45, 329–342.
Gustafsson, G., Raudberget, D., & Ström, M. (2016). Unveiling fundamental relationships in industrial product development. Procedia CIRP, 50, 204–209.
Haji Mazdarani, M. J., Hoseini Vaez, S. R., Hosseini, P., & Fathali, M. A. (2023). Reliability-based layout optimization of concentrically braced in 3D steel frames. Structures, 47, 1094–1112.
Hassanzadeh, A., & Gholizadeh, S. (2019). Collapse-performance-aided design optimization of steel concentrically braced frames. Engineering Structures, 197, 109411.
Kaveh, A. (2014a). Advances in metaheuristic algorithms for optimal design of structures. Springer International Publishing. 978-3-319-46173-1.
Kaveh, A. (2014b). Optimal structural analysis. Wiley. ISBN: 978-0-470-03329-6.
Kaveh, A., Biabani Hamedani, K., Milad Hosseini, S., & Bakhshpoori, T. (2020). Optimal design of planar steel frame structures utilizing meta-heuristic optimization algorithms. Structures, 25, 335–346.
Kaveh, A., & Khayatazad, M. (2012). A new meta-heuristic method: Ray optimization. Computers & Structures, 112–113, 283–294.
Kaveh, A., & Zaerreza, A. (2022). Reliability-based design optimization of the frame structures using the force method and SORA-DM framework. Structures, 45, 814–827.
Kazemzadeh Azad, S., & Aminbakhsh, S. (2021). High-dimensional optimization of large-scale steel truss structures using guided stochastic search. Structures, 33, 1439–1456.
Kazmarec, S., & Hogreav, S. (2016). Improving design efforts and assembly efficency of rotor blade carries through modularization. Procedia CIRP, 50(2016), 76–81.
Lee, K., Chung, M., Kim, S., & Shin, D. H. (2021). Damage detection of catenary mooring line based on recurrent neural networks. Ocean Engineering, 227, 108898.
Natarajan, A., Stolpe, M., & Njomo Wandji, W. (2019). Structural optimization based design of jacket type sub-structures for 10 MW offshore wind turbines. Ocean Engineering, 172, 629–640.
ABS Rules for Building and Classing Floating Production Installations, 2013.
Shan, W., Ding, Y., & Zhou, J. (2022). Automatic structural optimization design using sensitivity-based method. Structures, 46, 99–108.
Tabeshpour, M. R., & Fatemi, M. (2020). Optimum arrangement of braces in jacket platform based on strength and ductility. Marine Structures, 71, 102734.
Tomei, V. (2023). The effect of joint stiffness on optimization design strategies for gridshells: The role of rigid, semi-rigid and hinged joints. Structures, 48, 147–158.
Tunca, O. (2022). Design of buckling restrained steel braces using application programming interface between simulation and discrete optimization. Structures, 43, 752–766.
Van Woudenberg, T. R., & van der Meer, F. P. (2021). A grouping method for optimization of steel skeletal structures by applying a combinatorial search algorithm based on a fully stressed design. Engineering Structures, 249, 113299.
Villalba, D., París, J., Couceiro, I., Colominas, I., & Navarrina, F. (2022). Topology optimization of structures considering minimum weight and stress constraints by using the Overweight Approach. Engineering Structures, 273, 115071.
Vishnuraj, K., Janardhanan, S., & Sandeep, T. N. (2018). Design optimization of water injection module of topside facility of an FPSO. International Journal of Structural Mechanics and Finite Elements, 112, 23–40.
Xu, A., Li, S., Fu, J., Misra, A., & Zhao, R. (2023). A hybrid method for optimization of frame structures with good constructability. Engineering Structures, 276, 115338.
Zhang, P., Li, J., Gan, Y., Zhang, J., Qi, X., Le, C., & Ding, H. (2020). Bearing capacity and load transfer of brace topological in offshore wind turbine jacket structure. Ocean Engineering, 199, 107037.
Zhao, S., Bi, C., & Sun, Z. (2021). Engineering analysis of the dynamic characteristics of an electrical jacket platform of an offshore wind farm under seismic loads. Applied Ocean Research, 112, 102692.
Funding
This research received no funding or specific grant from any funding agency in the public, commercial, or not-for-profit sectors.
Author information
Authors and Affiliations
Contributions
All authors reviewed the manuscript.
Corresponding author
Ethics declarations
Competing interests
The authors declare no competing interests.
Conflict of interest
We declare that the authors have no competing interests as defined by Springer, or other interests that might be perceived to influence the results and/or discussion reported in this paper.
Additional information
Publisher's Note
Springer Nature remains neutral with regard to jurisdictional claims in published maps and institutional affiliations.
Rights and permissions
Springer Nature or its licensor (e.g. a society or other partner) holds exclusive rights to this article under a publishing agreement with the author(s) or other rightsholder(s); author self-archiving of the accepted manuscript version of this article is solely governed by the terms of such publishing agreement and applicable law.
About this article
Cite this article
Yadhav, A., Kulkarni, M. Optimal design of FPSO topside module for in-place, lift and weighing conditions utilizing meta-heuristic optimization algorithms. Asian J Civ Eng 24, 3055–3070 (2023). https://doi.org/10.1007/s42107-023-00694-2
Received:
Accepted:
Published:
Issue Date:
DOI: https://doi.org/10.1007/s42107-023-00694-2