Abstract
Shrub willow biomass crops (SWBC) have been developed as a biomass feedstock for bioenergy, biofuels, and bioproducts in the northeastern and midwestern USA as well as in Europe. A previous life cycle analysis in North America showed that the SWBC production system is a low-carbon fuel source. However, this analysis is potentially inaccurate due to the limited belowground biomass data and the lack of aboveground stool biomass data. This study provides new information on the above- and belowground biomass, the carbon–nitrogen (C/N) ratio, and the root/shoot (R/S) ratio of willow biomass crops (Salix × dasyclados [SV1]), which have been in production from 5 to 19 years. The measured amounts of biomass were: 2.6 to 4.1 odt ha−1 for foliage, 4.9 to 10.9 odt ha−1 for aboveground stool (AGS), 2.9 to 5.7 odt ha−1 for coarse roots (CR), 3.1 to 10.2 odt ha−1 for belowground stool (BGS), and 5.6 to 9.9 odt ha−1 for standing fine root (FR). The stem biomass production ranged from 7.0 to 18.0 odt ha−1 year−1 for the 5- and 19-year-old willows, respectively. C/N ratios ranged from 23 for foliage to 209 for belowground stool. An average R/S ratio of 2.0, calculated as total belowground biomass (BGS, CR, and FR) plus AGS divided by annual stem biomass, can be applied to estimate the total belowground biomass production of a mature SWBC. Based on AGS, BGS, and CR and standing FR biomass data, SWBC showed a net GHG potential of −42.9 Mg CO2 eq ha−1 at the end of seven 3-year rotations.



Similar content being viewed by others
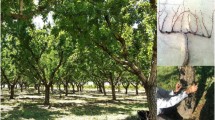
References
Heller MC, Keoleian GA, Volk TA (2003) Life cycle assessment of a willow bioenergy cropping system. Biomass Bioenergy 25:147–165
Keoleian GA, Volk TA (2005) Renewable energy from willow biomass crops: life cycle energy, environmental, and economic performance. Crit Rev Plant Sci 24:385–406
Kuzovkina YA, Volk TA (2009) The characterization of willow (Salix L.) varieties for use in ecological engineering applications: coordination of structure, function and autecology. Ecol Eng 35:1178–1189
Rowe RL, Street NR, Taylor G (2009) Identifying potential environmental impacts of large-scale deployment of dedicated bioenergy crops in the UK. Renew Sust Rev 13:271–290
Block RMA, Van Rees KCJ, Knight JD (2006) A review on fine root dynamics in Populus plantations. Agrofor Syst 67:73–84
Trumbore S, Da Costa ES, Nepstad DC, De Camargo PB, Martinelli LA, Ray D (2006) Dynamics of fine roots carbon in Amazonian tropical ecosystems and the contribution of roots to soil respiration. Glob Chang Biol 12:217–229
Adair EC, Reich PB, Hobbie SE, Knops JMH (2009) Interactive effects of time, CO2, N, and diversity on total belowground carbon allocation and ecosystem carbon storage in a grassland community. Ecosystems 12:1037–1052
Matthews RW (2001) Modeling of energy and carbon budgets of wood fuel coppice systems. Biomass Bioenergy 22:159–167
Volk TA (2002) Alternative methods of site preparations and coppice management during the establishment of short-rotation woody crops. Ph.D. dissertation, State University of New York—Environmental Science and Forestry, Syracuse
Grier CC, Vogt KA, Keyes MR, Edmonds RL (1981) Biomass distribution and above- and belowground yield in young and mature Abies amabilis zone ecosystem of the Washington Cascades. Can J For Res 11:155–167
Reyes MR, Grier CC (1981) Above and below-ground net yield in 40-year-old Douglas-fir stands on low and high productivity sites. Can J For Res 11:599–605
Yin X, Perry JA, Dixon RK (1989) Fine-root dynamics and biomass distribution in a Quercus ecosystem following harvesting. For Ecol Manag 27(3–4):159–177
Raich JW, Nadelhoffer KJ (1989) Belowground carbon allocation in forest ecosystems: global trends. Ecology 70:1346–1354
Canadell J, Roda F (1991) Root biomass of Quercus ilex in montane Mediterranean forest. Can J For Res 21:1771–1778
King JS, Albaugh TJ, Allen HL, Buford M, Strain BR, Dougherty P (2002) Below-ground carbon input to soil is controlled by nutrient availability and fine root dynamics in loblolly pine. New Phytol 154:389–398
Kopp RF, White EH, Abrahamson LP, Nowak CA, Burns KF (1993) Willow biomass trials in central New York State. Biomass Bioenergy 5:179–187
Kopp RF, Abrahamson LP, White EH, Burns KF, Nowak CA (1997) Cutting cycle and spacing effects on biomass production by a willow clone in New York. Biomass Bioenergy 12:313–319
Adegbidi HG, Volk TA, White EH, Abrahamson LP, Briggs RD, Beckelhaupt DH (2001) Biomass and nutrient removal by willow clones in experimental bioenergy plantations in New York State. Biomass Bioenergy 20:399–411
Tharakan PJ, Volk TA, Nowak CA, Abrahamson LP (2005) Morphological traits of 30 willow clones and their relationship to biomass production. Can J For Res 35:421–431
Tharakan PJ, Volk TA, Nowak CA, Ofezu G (2008) Canopy structure, light interception, and light-use efficiency in willow. Bioenergy Res 1:229–238
Volk TA, Abrahamson LP, et al (2011) Yields of willow biomass across a range of sites in North America. In: Booth E, Halford N, Shield I, Taylor G, Turley D, Voigt T (eds) Biomass and bioenergy crops IV: aspects of applied biology, 112. Association of Applied Biologist. pp 67–74
Hutton FZ, Rice CE (1977) Soil survey of Onondaga county, New York. USDA Conservation Service. In cooperation with Cornell University Agri. Exp. Stn., Ithaca, pp 233
Bickelhaupt DH, White EH, Schirmer C (2012) Laboratory manual for soil and plant tissue analysis. School of Forestry, State University of New York—College of Environmental Science and Forestry, Syracuse, New York 13210. pp 43–59
Rytter RM (1999) Fine-root production and turnover in a willow plantation estimated by different calculation methods. Scand J For Res 14:526–537
Rytter RM (2001) Biomass production and allocation, including fine-root turnover, and annual N uptake in lysimeter-grown basket willows. For Ecol Manag 140:177–192
Ulzen-Appiah F (2002) Soil organic matter in experimental short-rotation intensive culture (SRIC) systems: effects of cultural factors, season, and age. Ph.D. dissertation, State University of New York—Environmental Science and Forestry, Syracuse
Quaye AK, Volk TA (2011) Soil nutrient dynamics and biomass production in an organic and inorganic fertilized short rotation willow coppice system. In: Booth E, Halford N, Shield I, Taylor G, Turley D, Voigt T (eds) Biomass and bioenergy crops IV: aspects of applied biology, 112. "Association of Applied Biologist". pp 121–129
Quaye AK, Volk TA, Leopold DJ, Schirmer C (2011) Impacts of paper sludge and manure on soil and biomass production of willow. Biomass Bioenergy 35:2796–2806
Pacaldo RS, Volk TA, Briggs RD (2012) No significant differences in soil organic carbon contents along a chronosequence of shrub willow biomass crops. Biomass Bioenergy ("in review")
Pacaldo RS, Volk TA, Briggs RD (2011) Carbon balance in short rotation willow (Salix dasyclados) biomass crop across a 20-year chronosequence as affected by continuous production and tear-out treatments. In: Booth E, Halford N, Shield I, Taylor G, Turley D, Voigt T (eds) Biomass and bioenergy crops IV: aspects of applied biology, 112. "Association of Applied Biologist". pp 131–138
Sennerby-Forsse L, Ferm A, Kauppi A (1992) Coppicing ability and sustainability. In: Mitchell CP, Ford-Robertson JBF, Sennerby-Forsse L (eds) Ecophysiology of short rotation crops. Elsevier Applied Science, London, p 308
Goudriaan J, van Laar HH (1994) Modeling potential crop growth processes. Kluwer Academic, Dordrecht
Taylor JS, Blake TJ, Pharis RP (1982) The role of plant hormones and carbohydrates in the growth and survival of coppiced Eucalyptus seedlings. Physiol Plant 55:421–430
Schlesinger WH (1997) Biogeochemistry: an analysis of global change. Academic, New York, pp 127–164
Nadelhoffer KJ, Aber JD, Melillo JM (1985) Fine roots, net primary yield, and soil nitrogen availability: a new hypothesis. Ecology 66(4):1377–1390
Ares A, Peinemann N (1992) Fine-root distribution of coniferous plantations in relation to site in southern Buenos Aires, Argentina. Can J For Res 22(11):1575–1582
Zan CS, Fyles JW, Girouard P, Samson RA (2001) Carbon sequestration in perennial bioenergy, annual corn and uncultivated systems in southern Quebec. Agric Ecosyst Environ 86:135–144
Wilson JB (1988) A review of evidence on the control of shoot:root ratio, in relation to models. Ann Bot 61:433–449
Coder KD (1998) Control of shoot/root balance in trees. University of Georgia Cooperative Extension Service Forest Resources publication FOR98-3
Cannell MGR, Milne R, Sheppard LJ, Unsworth MH (1987) Radiation interception and productivity of willow. J Appl Ecol 24:261–278
Johansson T, Hjelm B (2012) Stump and root biomass in poplar stands. Forests 3:168–178
Rytter RM (1997) Fine-root production and carbon and nitrogen allocation in basket willows. Ph.D. thesis, Swedish University of Agricultural Sciences, Uppsala.
Vanninen P, Ylitalo H, Sievänen R, Mäkelä A (1996) Effects of age and site quality on the distribution of biomass in Scots pine (Pinus sylvestris L.). Trees-Struct Funct 10(4):231–238
Crow P, Houston TJ (2004) The influence of soil and coppice cycle on the rooting habit of short rotation poplar and willow coppice. Biomass Bioenergy 26:497–505
Dickmann D, Pregitzer KS (1992) The structure and dynamics of woody plant roots systems. In: Mitchell CP, Ford-Robertson JBF, Sennerby-Forsse L (eds) Ecophysiology of short rotation crops. Elsevier Applied Science, London, p 308
Faulkner HG (1976) Root distribution, amount, and development from 5-year-old Populus × euramericana (Dode) Guinier. "M.S.F. thesis, University of Toronto", pp 130
Rytter RM, Hansson AC (1996) Seasonal amount, growth and depth distribution of fine roots in an irrigated and fertilized Salix viminalis L. plantation. Biomass Bioenergy 11:129–137
Cavalier J (1992) Fine-root biomass and soil properties in semi-deciduous and a lower montane rain forest in Panama. Plant Soil 142(2):187–201
Gill R, Burke IC, Milchunas DG, Luenroth WK (1999) Relationship between root biomass and soil organic matter pools in the shortgrass steppe of Eastern Colorado. Ecosystems 2:226–236
Yang YS, Chen GS, Lin P, Xie JS, Guo JF (2004) Fine root distribution, seasonal pattern and yield in four plantations compared with a natural forest in subtropical China. Ann For Sci 61(7):617–627
Farrish KW (1991) Spatial and temporal fine-root distribution in three Louisiana forest soils. Soil Sci Soc Am J 55(6):1752–1757
Martin PJ, Stephens W (2006) Willow growth in response to nutrients and moisture on a clay landfill cap soil. I. Growth and biomass production. Bioresour Technol 97:437–448
Rytter RM, Rytter L (1998) Growth, decay, and turnover rates of fine roots of basket willow. Can J For Res 28:893–902
Proe MF, Griffiths JH, Craig J (2002) Effects of spacing, species and coppicing on leaf area, light interception and photosynthesis in short rotation forestry. Biomass Bioenergy 23:315–326
Dowell RC, Gibbins D, Rhoads JL, Pallardy SG (2009) Biomass production physiology and soil carbon dynamics in short-rotation-grown Populus deltoides and P. deltoides × P. nigra hybrids. For Ecol Manag 257:134–142
Cunnif J, Shield I, Barraclough T, Castle M, Hanley S, Andralojc J, et al (2011) BSBES-biomass-selecting traits to optimise biomass yield of SRC willow. In: Booth E, Halford N, Shield I, Taylor G, Turley D, Voigt T (eds) Biomass and bioenergy crops IV: aspects of applied biology, 112. "Association of Applied Biologists", pp 83–91
Prescott CE (2010) Litter decomposition: what controls it and how can we alter it to sequester more carbon in forest soils? Biogeochemistry 101:133–149
Zhang D, Hui D, Luo Y, Zhou G (2008) Rates of litter decomposition in terrestrial ecosystem: global patterns and controlling factors. J Plant Ecol 1:85–86
Brady NC, Weil RR (2008) The nature and properties of soils, 14th edn. "Prentice Hall", p 506
Grigal DF, Berguson WE (1998) Soil carbon changes associated with short rotation systems. Biomass Bioenergy 14:371–377
Acknowledgments
This research was carried out through the funding support from the United States Department of Agriculture—Cooperative State Research, Education, and Extension Service (USDA CSREES). Special thanks go to Rebecca Allmond, Eric Fabio, Philip Castellano, Ken Burns, Chuck Schirmer, Jacob Bakowski, Tyler Harvey, Gabe Kellman, Jason Maurer, Ryan Newby, and Devin Mc Bride for their valuable assistance in field and lab works.
Author information
Authors and Affiliations
Corresponding author
Rights and permissions
About this article
Cite this article
Pacaldo, R.S., Volk, T.A. & Briggs, R.D. Greenhouse Gas Potentials of Shrub Willow Biomass Crops Based on Below- and Aboveground Biomass Inventory Along a 19-Year Chronosequence. Bioenerg. Res. 6, 252–262 (2013). https://doi.org/10.1007/s12155-012-9250-y
Published:
Issue Date:
DOI: https://doi.org/10.1007/s12155-012-9250-y