Abstract
Redox properties enable copper to perform its essential role in many biological processes, but they can also convert it into a potentially hazardous element. Its dyshomeostasis may have serious neurological consequences, and its possible involvement in Parkinson’s disease and other neurodegenerative disorders has been suggested. The in vitro and ex vivo ability of copper to increase oxidative stress has already been demonstrated, and the aim of the present study was to assess in vivo the capacity of copper to cause brain oxidative damage and its ability to increase the dopaminergic degeneration induced by 6-hydroxydopamine. We found that chronic copper administration (10 mg Cu2+/kg/day, IP) causes its accumulation in different brain areas (cortex, striatum, nigra) and was accompanied by an increase in TBARS levels and a decrease in protein free-thiol content in the cortex. A decrease in catalase activity and an increase in glutathione peroxidase activity were also observed in the cortex. The intrastriatal administration of Cu2+ caused an increase in some indices of oxidative stress (TBARS and protein free-thiol content) in striatum and nigra, but was unable to induce dopaminergic degeneration. However, when copper was intrastriatally coadministered with 6-hydroxydopamine, it increased dopaminergic degeneration, a fact that was also accompanied by an increase in the assayed indices of oxidative stress, a decrease in catalase activity, and an augmentation in glutathione activity. Evidently, copper cannot cause neurodegeneration per se, but may potentiate the action of other factors involved in the pathogenesis of Parkinson’s disease through oxidative stress.





Similar content being viewed by others
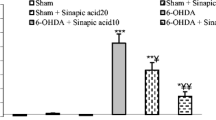
References
Gaier ED, Eipper BA, Mains RE (2013) Copper signaling in the mammalian nervous system: synaptic effects. J Neurosci Res 91:2–19. https://doi.org/10.1002/jnr.23143
Scheiber IF, Mercer JF, Dringen R (2014) Metabolism and functions of copper in brain. Prog Neurobiol 116:33–57. https://doi.org/10.1016/j.pneurobio.2014.01.002
Halliwell B, Gutteridge JM (1990) Role of free radicals and catalytic metal ions in human disease: an overview. Methods Enzymol 186:1–85
Youdim MB, Fridkin M, Zheng H (2005) Bifunctional drug derivatives of MAO-B inhibitor rasagiline and iron chelator VK-28 as a more effective approach to treatment of brain ageing and ageing neurodegenerative diseases. Mech Ageing Dev 126(2):317–326. https://doi.org/10.1016/j.mad.2004.08.023
Dringen R (2000) Glutathione metabolism and oxidative stress in neurodegeneration. Eur J Biochem 267:4903
Rossi L, Lombardo MF, Ciriolo MR, Rotilio G (2004) Mitochondrial dysfunction in neurodegenerative diseases associated with copper imbalance. Neurochem Res 29:493–504. https://doi.org/10.1023/B:NERE.0000014820.99232.8a
Halliwell B (2006) Oxidative stress and neurodegeneration: where are we now? J Neurochem 97:1634–1658. https://doi.org/10.1111/j.1471-4159.2006.03907.x
Wittung-Stafshede P (2015) Unresolved questions in human copper pump mechanisms. Q Rev Biophys 48:471–478. https://doi.org/10.1017/S0033583515000128
Choi B-S, Zheng W (2009) Copper transport to the brain by the blood-brain barrier and blood-CSF barrier. Brain Res 1248:14–21. https://doi.org/10.1016/j.brainres.2008.10.056
Arciello M, Capo CR, D’Annibale S, Cozzolino M, Ferri A, Carrì MT, Rossi L (2011) Copper depletion increases the mitochondrial-associated SOD1 in neuronal cells. Biometals 24:269–278. https://doi.org/10.1007/s10534-010-9392-3
Nevitt T, Ohrvik H, Thiele DJ (2012) Charting the travels of copper in eukaryotes from yeast to mammals. Biochim Biophys Acta 1823:1580–1593. https://doi.org/10.1016/j.bbamcr.2012.02.011
Rahil-Khazen R, Bolann BJ, Ulvik RJ (2002) Correlations of trace element levels within and between different normal autopsy tissues analyzed by inductively coupled plasma atomic emission spectrometry (ICP-AES). Biometals 15:87–98
Lech T, Sadlik JK (2007) Copper concentration in body tissues and fluids in normal subjects of southern Poland. Biol Trace Elem Res 118:10–15. https://doi.org/10.1007/s12011-007-0014-z
Davies KM, Bohic S, Carmona A, Ortega R, Cottam V, Hare DJ, Finberg JPM, Reyes S et al (2014) Copper pathology in vulnerable brain regions in Parkinson’s disease. Neurobiol Aging 35:858–866. https://doi.org/10.1016/j.neurobiolaging.2013.09.034
Davies KM, Hare DJ, Cottam V, Chen N, Hilgers L, Halliday G, Mercer JFB, Double KL (2013) Localization of copper and copper transporters in the human brain. Metallomics 5:43–51. https://doi.org/10.1039/C2MT20151H
Warren PJ, Earl CJ, Thompson RHS (1960) The distribution of copper in human brain. Brain 83:709–717. https://doi.org/10.1093/brain/83.4.709
Goldberg WJ, Allen N (1981) Determination of Cu, Mn, Fe, and Ca in six regions of normal human brain, by atomic absorption spectroscopy. Clin Chem 27:562–564
Popescu BFG, George MJ, Bergmann U et al (2009) Mapping metals in Parkinson’s and normal brain using rapid-scanning X-ray fluorescence. Phys Med Biol 54:651–663. https://doi.org/10.1088/0031-9155/54/3/012
Enochs WS, Sarna T, Zecca L, Riley PA, Swartz HM (1994) The roles of neuromelanin, binding of metal ions, and oxidative cytotoxicity in the pathogenesis of Parkinson’s disease: a hypothesis. J Neural Transm 7:83–100
Szerdahelyi P, Kasa P (1986) Histochemical demonstration of copper in normal rat brain and spinal cord. Evidence of localization in glial cells. Histochemistry 85:341–347
Kodama H (1993) Recent developments in Menkes disease. J Inherit Metab Dis 16:791–799. https://doi.org/10.1007/BF00711911
Becker JS, Salber D (2010) New mass spectrometric tools in brain research. Trends Anal Chem 29:966–979. https://doi.org/10.1016/j.trac.2010.06.009
Fahn S (2003) Description of Parkinson’s disease as a clinical syndrome. Ann N Y Acad Sci 991:1–14
de Lau LM, Breteler MM (2006) Epidemiology of Parkinson’s disease. Lancet Neurol 5:525–535. https://doi.org/10.1016/S1474-4422(06)70471-9
McNaught KS, Olanow CW (2006) Protein aggregation in the pathogenesis of familial and sporadic Parkinson’s disease. Neurobiol Aging 27:530–545. https://doi.org/10.1016/j.neurobiolaging.2005.08.012
Goedert M, Spillantini MG, Del Tredici K, Braak H (2013) 100 years of Lewy pathology. Nat Rev Neurol 9:13–24. https://doi.org/10.1038/nrneurol.2012.242
Sulzer D (2007) Multiple hit hypotheses for dopamine neuron loss in Parkinson’s disease. Trends Neurosci 30:244–250. https://doi.org/10.1016/j.tins.2007.03.009
Antony PM, Diederich NJ, Kruger R, Balling R (2013) The hallmarks of Parkinson’s disease. FEBS J 280:5981–5993. https://doi.org/10.1111/febs.12335
Pall HS, Williams AC, Blake DR, Lunec J, Gutteridge JM, Hall M, Taylor A (1987) Raised cerebrospinal-fluid copper concentration in Parkinson’s disease. Lancet 2(8553):238–241
Hozumi I, Hasegawa T, Honda A, Ozawa K, Hayashi Y, Hashimoto K, Yamada M, Koumura A et al (2011) Patterns of levels of biological metals in CSF differ among neurodegenerative diseases. J Neurol Sci 303:95–99. https://doi.org/10.1016/j.jns.2011.01.003
Taly AB, Meenakshi-Sundaram S, Sinha S, Swamy HS, Arunodaya GR (2007) Wilson disease: description of 282 patients evaluated over 3 decades. Medicine 86:112–121. https://doi.org/10.1097/MD.0b013e318045a00e
Paris I, Dagnino-Subiabre A, Marcelain K, Bennett LB, Caviedes P, Caviedes R, Azar CO, Segura-Aguilar J (2001) Copper neurotoxicity is dependent on dopamine-mediated copper uptake and one-electron reduction of aminochrome in a rat substantia nigra neuronal cell line. J Neurochem 77:519–529
Gorell JM, Peterson EL, Rybicki BA, Johnson CC (2004) Multiple risk factors for Parkinson’s disease. J Neurol Sci 217:169–174
Przedborski S, Levivier M, Jiang H et al (1995) Dose-dependent lesions of the dopaminergic nigrostriatal pathway induced by intrastriatal injection of 6-hydroxydopamine. Neuroscience 67:631–647
Soto-Otero R, Méndez-Álvarez E, Hermida-Ameijeiras A et al (2002) Effects of (-)-nicotine and (-)-cotinine on 6-hydroxydopamine-induced oxidative stress and neurotoxicity: relevance for Parkinson’s disease. Biochem Pharmacol 64:125–135
Paxinos G, Watson C (2007) The rat brain in stereotaxic coordinates, 6th edn. Academic, London
Sánchez-Iglesias S, Rey P, Méndez-Álvarez E, Labandeira-García JL, Soto-Otero R (2007) Time-course of brain oxidative damage caused by intrastriatal administration of 6-hydroxydopamine in a rat model of Parkinson’s disease. Neurochem Res 32:99–105. https://doi.org/10.1007/s11064-006-9232-6
Markwell MA, Haas SM, Bieber LL, Tolbert NE (1978) A modification of the Lowry procedure to simplify protein determination in membrane and lipoprotein samples. Anal Biochem 87:206–210
Hermida-Ameijeiras A, Méndez-Álvarez E, Sánchez-Iglesias S et al (2004) Autoxidation and MAO-mediated metabolism of dopamine as a potential cause of oxidative stress: role of ferrous and ferric ions. Neurochem Int 45:103–116. https://doi.org/10.1016/j.neuint.2003.11.018
Flohe L, Gunzler WA (1984) Assays of glutathione peroxidase. Methods Enzymol 105:114–121
Aebi H (1984) Catalase in vitro. Methods Enzymol 105:121–126
López-Real A, Rey P, Soto-Otero R et al (2005) Angiotensin-converting enzyme inhibition reduces oxidative stress and protects dopaminergic neurons in a 6-hydroxydopamine rat model of parkinsonism. J Neurosci Res 81:865–873. https://doi.org/10.1002/jnr.20598
Gundersen HJ, Bendtsen TF, Korbo L et al (1988) Some new, simple and efficient stereological methods and their use in pathological research and diagnosis. APMIS 96:379–394
Torres EM, Meldrum A, Kirik D, Dunnett SB (2006) An investigation of the problem of two-layered immunohistochemical staining in paraformaldehyde fixed sections. J Neurosci Methods 158:64–74. https://doi.org/10.1016/j.jneumeth.2006.05.016
Cruces-Sande A, Méndez-Álvarez E, Soto-Otero R (2017) Copper increases the ability of 6-hydroxydopamine to generate oxidative stress and the ability of ascorbate and glutathione to potentiate this effect: potential implications in Parkinson’s disease. J Neurochem 141:738–749. https://doi.org/10.1111/jnc.14019
Zheng W, Monnot AD (2012) Regulation of brain iron and copper homeostasis by brain barrier systems: implication in neurodegenerative diseases. Pharmacol Ther 133:177–188. https://doi.org/10.1016/j.pharmthera.2011.10.006
Lutsenko S, Barnes NL, Bartee MY, Dmitriev OY (2007) Function and regulation of human copper-transporting ATPases. Physiol Rev 87:1011–1046. https://doi.org/10.1152/physrev.00004.2006
Pal A, Prasad R (2016) Regional distribution of copper, zinc and iron in brain of Wistar rat model for non-Wilsonian brain copper toxicosis. Indian J Clin Biochem 31:93–98. https://doi.org/10.1007/s12291-015-0503-3
Sánchez-Iglesias S, Méndez-Álvarez E, Iglesias-González J, Muñoz-Patiño A, Sánchez-Sellero I, Labandeira-García JL, Soto-Otero R (2009) Brain oxidative stress and selective behaviour of aluminium in specific areas of rat brain: potential effects in a 6-OHDA-induced model of Parkinson’s disease. J Neurochem 109:879–888
Iglesias-González J, Sánchez-Iglesias S, Méndez-Álvarez E, Rose S, Hikima A, Jenner P, Soto-Otero R (2012) Differential toxicity of 6-hydroxydopamine in SH-SY5Y human neuroblastoma cells and rat brain mitochondria: protective role of catalase and superoxide dismutase. Neurochem Res 37:2150–2160. https://doi.org/10.1007/s11064-012-0838-6
Iglesias-González J, Sánchez-Iglesias S, Beiras-Iglesias A, Méndez-Álvarez E, Soto-Otero R (2017) Effects of aluminium on rat brain mitochondria bioenergetics: an in vitro and in vivo study. Mol Neurobiol 54:563–570. https://doi.org/10.1007/s12035-015-9650-z
Yu WR, Jiang H, Wang J, Xie JX (2008) Copper (Cu2+) induces degeneration of dopaminergic neurons in the nigrostriatal system of rats. Neurosci Bull 24:73–78. https://doi.org/10.1007/s12264-008-0073-y
Funding
This study was partially supported by the Galician Government (XUGA), Santiago de Compostela, Spain (No. 09CSA005298PR), the Spanish Ministry of Economy and Competitiveness (grant number BFU2015-70523), the Spanish Ministry of Health (CIBERNED), and FEDER (Regional European Development Fund).
Author information
Authors and Affiliations
Corresponding author
Ethics declarations
Conflict of Interest
The authors declare that they have no conflicts of interest.
Rights and permissions
About this article
Cite this article
Cruces-Sande, A., Rodríguez-Pérez, A.I., Herbello-Hermelo, P. et al. Copper Increases Brain Oxidative Stress and Enhances the Ability of 6-Hydroxydopamine to Cause Dopaminergic Degeneration in a Rat Model of Parkinson’s Disease. Mol Neurobiol 56, 2845–2854 (2019). https://doi.org/10.1007/s12035-018-1274-7
Received:
Accepted:
Published:
Issue Date:
DOI: https://doi.org/10.1007/s12035-018-1274-7