Abstract
Autophagy is responsible for degradation of non-essential or damaged cellular constituents and damaged organelles. The autophagy pathway maintains efficient cellular metabolism and reduces cellular stress by removing additional and pathogenic components. Dysfunctional autophagy underlies several diseases. Thus, several research groups have worked toward elucidating key steps in this pathway. Autophagy can be studied by animal modeling, chemical modulators, and in vitro disease modeling with induced pluripotent stem cells (iPSC) as a loss-of-function platform. The introduction of iPSC technology, which has the capability to maintain the genetic background, has facilitated in vitro modeling of some diseases. Furthermore, iPSC technology can be used as a platform to study defective cellular and molecular pathways during development and unravel novel steps in signaling pathways of health and disease. Different studies have used iPSC technology to explore the role of autophagy in disease pathogenesis which could not have been addressed by animal modeling or chemical inducers/inhibitors. In this review, we discuss iPSC models of autophagy-associated disorders where the disease is caused due to mutations in autophagy-related genes. We classified this group as “primary autophagy induced defects (PAID)”. There are iPSC models of diseases in which the primary cause is not dysfunctional autophagy, but autophagy is impaired secondary to disease phenotypes. We call this group “secondary autophagy induced defects (SAID)” and discuss them.

Graphical abstract




Similar content being viewed by others
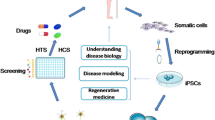
Abbreviations
- 5AdC:
-
5-aza-20-deoxycytidine
- 3-MA:
-
3-methyladenine
- AD:
-
Alzheimer’s disease
- ADPKD:
-
Autosomal dominant polycystic kidney disease
- ALP:
-
Autophagy-lysosome pathway
- ALS:
-
Amyotrophic lateral sclerosis
- ANP:
-
Atrial natriuretic peptide
- APP:
-
Amyloid-β precursor protein
- ASM:
-
Acid sphingomyelinase
- ATAD3A:
-
AAA-domain containing protein 3A
- AVs:
-
Autophagic vacuoles
- Aβ:
-
Amyloid beta
- BCD:
-
Bietti’s crystalline dystrophy
- BNP:
-
Brain natriuretic peptide
- BVVL:
-
Brown-Vialetto-Van Laere syndrome
- C9ORF72:
-
Chromosome 9 open reading frame 72
- CCCP:
-
Carbonyl cyanide m-chlorophenyl hydrazone
- CHMP2B:
-
Charged multivesicular body protein 2B
- CM:
-
Cardiomyocyte
- CMA:
-
Chaperone-mediated autophagy
- CMT:
-
Charcot-Marie-Tooth
- CYP4V2:
-
Cytochrome P450 family 4 subfamily V member 2
- DA:
-
Dopaminergic neurons
- DBA:
-
Diamond-Blackfan anemia
- DCM:
-
Dilated cardiomyopathy
- DHCR7:
-
7-dehydrocholesterol reductase
- DRP1:
-
Dynamin-related protein 1
- ER:
-
Endoplasmic reticulum
- FAD:
-
Familial AD
- FTD:
-
Frontotemporal dementia
- GAA:
-
Acid alpha-glucosidase
- GAGs:
-
Glycosaminoglycans
- GBA:
-
beta-Glucocerebrosidase
- GCase:
-
Glucocerebrosidase
- GSDs:
-
Glycogen storage diseases
- HPBCD:
-
2-hydroxypropyl-β-CD
- HPGCD:
-
2-hydroxypropyl-γ-CD
- Hsc70:
-
Heat shock cognate 71 kDa protein
- HSCs:
-
Hematopoietic stem cells
- HSP:
-
Hereditary spastic paraplegia
- hESC:
-
Human embryonic stem cell
- IDUA:
-
α-L-iduronidase
- IP3:
-
Inositol three phosphate
- JNCL:
-
Juvenile neuronal ceroid lipofuscinosis
- LAMP-2:
-
Lysosomal-associated membrane protein type 2
- LC3-II:
-
Light chain 3-II
- LIMP-II:
-
Lysosomal integral membrane protein-2
- LMNA:
-
Lamin A/C
- LRRK2:
-
Leucine-rich repeat kinase 2
- LSDs:
-
Lysosomal storage diseases
- MAPT:
-
Microtubule-associated protein tau
- MELAS:
-
Mitochondrial encephalomyopathy, lactic acidosis, and stroke-like episodes
- MIRO1:
-
Mitochondrial Rho GTPase 1
- MN:
-
Motor neuron
- MPS I:
-
Mucopolysaccharidoses type I
- MSA:
-
Multiple system atrophy
- mTOR:
-
Mammalian target of rapamycin
- MFN2:
-
Mitofusin 2
- MT-TL1:
-
Mitochondrial tRNALeu(UUR) gene
- NPC:
-
Niemann-Pick disease type C
- NPCs:
-
Neuronal precursor cells
- NSCs:
-
Neural stem cells
- NTG:
-
Normal tension glaucoma
- OPTN:
-
Optineurin
- PAID:
-
Primary autophagy induced defects
- PARK7:
-
Protein deglycase DJ-1
- PD:
-
Pompe disease
- PINK1:
-
PTEN induced putative kinase 1
- PKD:
-
Polycystin
- POAG:
-
Primary open angle glaucoma
- polyQ-AR:
-
Polyglutamine-expansion in androgen receptor
- PRKN:
-
Parkin RBR E3 ubiquitin protein ligase
- PSEN1:
-
Presenilin 1
- RAN:
-
Repeat-associated non-ATG
- rhGAA:
-
Recombinant human GAA
- ROS:
-
Reactive oxygen species
- RP:
-
Retinitis pigmentosa
- RPE:
-
Retinal pigment epithelium
- RyR2:
-
Ryanodine receptor type 2
- SAID:
-
Secondary autophagy induced defect
- SBMA:
-
Spinal and bulbar muscular atrophy
- SCA3:
-
Spinocerebellar ataxia-3
- SQSTM1:
-
Sequestosome 1
- SLOS:
-
Smith-Lemli-Opitz syndrome
- SMC:
-
Smooth muscle cell
- SNCA:
-
Synuclein alpha
- SOD1:
-
Superoxide dismutase
- SPG11:
-
Spastic paraplegia 11
- SQSTM1:
-
Sequestosome1
- TBK1:
-
TANK binding kinase 1
- TDP-43:
-
TAR DNA binding protein-43
- TFEB:
-
Transcription factor EB
- TPP1:
-
Tripeptidyl peptidase I
- TREM2:
-
Triggering receptor expressed on myeloid cells 2
- TRNT1:
-
tRNA nucleotidyl transferase, CCA-adding, 1
- TSC2:
-
Tuberous sclerosis complex 2
- UBQLN:
-
Ubiquilin
- VCP:
-
Valosin-containing protein
- VEGF:
-
Vascular endothelial growth factor
- VSD:
-
Ventricular septal defect
References
Bravo-San Pedro, J. M., Kroemer, G., & Galluzzi, L. (2017). Autophagy and mitophagy in cardiovascular disease. Circulation Research, 120(11), 1812–1824.
Orogo, A. M., & Gustafsson, Å. G. (2015). Therapeutic targeting of autophagy: potential and concerns in treating cardiovascular disease. Circulation Research, 116, 489–503.
Alessandri, C., Barbati, C., Vacirca, D., Piscopo, P., Confaloni, A., Sanchez, M., Maselli, A., Colasanti, T., Conti, F., & Truglia, S. (2012). T lymphocytes from patients with systemic lupus erythematosus are resistant to induction of autophagy. The FASEB Journal, 26(11), 4722–4732.
Weykopf, B., Haupt, S., Jungverdorben, J., Flitsch, L. J., Hebisch, M., Liu, G. H., Suzuki, K., Belmonte, J. C. I., Peitz, M., & Blaess, S. (2019). Induced pluripotent stem cell-based modeling of mutant LRRK 2‐associated Parkinson’s disease. European Journal of Neuroscience, 49(4), 561–589.
Zhang, J. (2013). Autophagy and mitophagy in cellular damage control. Redox Biology, 1(1), 19–23.
Shojaei, S., Suresh, M., Klionsky, D. J., Labouta, H. I., & Ghavami, S. (2020). Autophagy and SARS-CoV-2 infection: Apossible smart targeting of the autophagy pathway. Virulence, 11(1), 805–810. https://doi.org/10.1080/21505594.2020.1780088.
Brun, P., Tarricone, E., Di Stefano, A., Mattiuzzo, E., Mehrbod, P., Ghavami, S., & Leonardi, A. (2020). The regulatory activity of autophagy in conjunctival fibroblasts and its possible role in vernal keratoconjunctivitis. The Journal of Allergy and Clinical Immunology. https://doi.org/10.1016/j.jaci.2020.03.013
Klionsky, D. J., Abdalla, F. C., Abeliovich, H., Abraham, R. T., Acevedo-Arozena, A., Adeli, K., Agholme, L., Agnello, M., Agostinis, P., & Aguirre-Ghiso, J. A. (2012). Guidelines for the use and interpretation of assays for monitoring autophagy. Autophagy, 8(4), 445–544.
Mehrbod, P., Ande, S. R., Alizadeh, J., Rahimizadeh, S., Shariati, A., Malek, H., Hashemi, M., Glover, K. K. M., Sher, A. A., Coombs, K. M., & Ghavami, S. (2019). The roles of apoptosis, autophagy and unfolded protein response in arbovirus, influenza virus, and HIV infections. Virulence, 10(1), 376–413. https://doi.org/10.1080/21505594.2019.1605803.
Shojaei, S., Koleini, N., Samiei, E., Aghaei, M., Cole, L. K., Alizadeh, J., Islam, M. I., Vosoughi, A. R., Albokashy, M., Butterfield, Y., Marzban, H., Xu, F., Thliveris, J., Kardami, E., Hatch, G. M., Eftekharpour, E., Akbari, M., Hombach-Klonisch, S., Klonisch, T., & Ghavami, S. (2020). Simvastatin increases temozolomide-induced cell death by targeting the fusion of autophagosomes and lysosomes. The FEBS Journal, 287(5), 1005–1034. https://doi.org/10.1111/febs.15069
Mei, Y., Thompson, M. D., Cohen, R. A., & Tong, X. (2015) Autophagy and oxidative stress in cardiovascular diseases. Biochimica et Biophysica Acta (BBA)-Molecular Basis of Disease, 1852(2), 243–251.
Hamacher-Brady, A., Brady, N. R., & Gottlieb, R. A. (2006). Enhancing macroautophagy protects against ischemia/reperfusion injury in cardiac myocytes. Journal of Biological Chemistry, 281(40), 29776–29787.
Pattison, J. S., Osinska, H., & Robbins, J. (2011). Atg7 induces basal autophagy and rescues autophagic deficiency in CryABR120G cardiomyocytes. Circulation Research, 109(2), 151–160.
An, M., Ryu, D.-R., Won Park, J., Ha Choi, J., Park, E.-M., Eun Lee, K., Woo, M., & Kim, M. (2017). ULK1 prevents cardiac dysfunction in obesity through autophagy-meditated regulation of lipid metabolism. Cardiovascular Research, 113(10), 1137–1147.
Billia, F., Hauck, L., Konecny, F., Rao, V., Shen, J., & Mak, T. W. (2011). PTEN-inducible kinase 1 (PINK1)/Park6 is indispensable for normal heart function. Proceedings of the National Academy of Sciences, 108(23), 9572–9577.
Ghosh, R., & Pattison, J. S. (2018). Macroautophagy and chaperone-mediated autophagy in heart failure: The known and the unknown. Oxidative Medicine and Cellular Longevity, 2018, 8602041.
Sarkar, S. (2013). Regulation of autophagy by mTOR-dependent and mTOR-independent pathways: autophagy dysfunction in neurodegenerative diseases and therapeutic application of autophagy enhancers. London: Portland Press Ltd.
Mishra, P., Ammanathan, V., & Manjithaya, R. (2018). Chemical biology strategies to study autophagy. Frontiers in Cell and Developmental Biology, 6, 160.
McMullen, J. R., Sherwood, M. C., Tarnavski, O., Zhang, L., Dorfman, A. L., Shioi, T., & Izumo, S. (2004). Inhibition of mTOR signaling with rapamycin regresses established cardiac hypertrophy induced by pressure overload. Circulation, 109(24), 3050–3055.
Das, A., Salloum, F. N., Durrant, D., Ockaili, R., & Kukreja, R. C. (2012). Rapamycin protects against myocardial ischemia–reperfusion injury through JAK2–STAT3 signaling pathway. Journal of Molecular and Cellular Cardiology, 53(6), 858–869.
Das, A., Durrant, D., Koka, S., Salloum, F. N., Xi, L., & Kukreja, R. C. (2014). Mammalian target of rapamycin (mTOR) inhibition with rapamycin improves cardiac function in type 2 diabetic mice potential role of attenuated oxidative stress and altered contractile protein expression. Journal of Biological Chemistry, 289(7), 4145–4160.
Matsui, Y., Takagi, H., Qu, X., Abdellatif, M., Sakoda, H., Asano, T., Levine, B., & Sadoshima, J. (2007). Distinct roles of autophagy in the heart during ischemia and reperfusion: roles of AMP-activated protein kinase and Beclin 1 in mediating autophagy. Circulation research, 100(6), 914–922.
Lu, L., Wu, W., Yan, J., Li, X., Yu, H., & Yu, X. (2009). Adriamycin-induced autophagic cardiomyocyte death plays a pathogenic role in a rat model of heart failure. International Journal of Cardiology, 134(1), 82–90.
Piyush Mishra, V. A., & Manjithaya*, R. Chemical biology strategies to study autophagy. Frontiers in Cell and Developmental Biology 6, 160. https://doi.org/10.3389/fcell.2018.00160.
Sarkar, S. (2013). Regulation of autophagy by mTOR-dependent and mTOR-independent pathways: autophagy dysfunction in neurodegenerative diseases and therapeutic application of autophagy enhancers. Biochemical Society Transactions, 41, 1103–1130.
Kim, C. (2015). iPSC technology-Powerful hand for disease modeling and therapeutic screen. BMB Reports, 48(5), 256.
O’Prey, J., Sakamaki, J., Baudot, A., New, M., Van Acker, T., Tooze, S., Long, J., & Ryan, K. (2017). Application of CRISPR/Cas9 to autophagy research. In: Methods in Enzymology, (Vol. 588, pp. 79–108). Amsterdam: Elsevier.
Singh, R., Kaushik, S., Wang, Y., Xiang, Y., Novak, I., Komatsu, M., Tanaka, K., Cuervo, A. M., & Czaja, M. J. (2009). Autophagy regulates lipid metabolism. Nature, 458(7242), 1131.
Mathew, R., & White, E. (2011). Autophagy in tumorigenesis and energy metabolism: friend by day, foe by night. Current Opinion in Genetics & Development, 21(1), 113–119.
Ryter, S. W., Lee, S.-J., Smith, A., & Choi, A. M. (2010). Autophagy in vascular disease. Proceedings of the American Thoracic Society, 7(1), 40–47.
Nixon, R. A. (2013). The role of autophagy in neurodegenerative disease. Nature Medicine, 19(8), 983.
Bonaldo, P., & Sandri, M. (2013). Cellular and molecular mechanisms of muscle atrophy. Disease Models & Mechanisms, 6(1), 25–39.
Schneider, J. L., & Cuervo, A. M. (2014). Liver autophagy: much more than just taking out the trash. Nature Reviews Gastroenterology & Hepatology, 11(3), 187.
Murrow, L., & Debnath, J. (2013). Autophagy as a stress-response and quality-control mechanism: implications for cell injury and human disease. Annual Review of Pathology: Mechanisms of Disease, 8, 105–137.
Hata, M., Ikeda, H. O., Iwai, S., Iida, Y., Gotoh, N., Asaka, I., Ikeda, K., Isobe, Y., Hori, A., & Nakagawa, S. (2018). Reduction of lipid accumulation rescues Bietti’s crystalline dystrophy phenotypes. Proceedings of the National Academy of Sciences, 115(15), 3936–3941.
Siu, C.-W. Lee, Y.-K., Ho, J.C.-Y., Lai, W.-H., Chan, Y.-C., Ng, K.-M., Wong, L.-Y., Au, K.-W., Lau, Y.-M.,Zhang, J., Lay, K. W., Colman, A., & Tse, H.-F. (2012) Modeling of lamin A/C mutation premature cardiac aging using patient‐specific induced pluripotent stem cells. Aging 4(11), 803–822. https://doi.org/10.18632/aging.100503.
Singletary, K., & Milner, J. (2008). Diet, autophagy, and cancer: a review. Cancer Epidemiology and Prevention Biomarkers, 17(7), 1596–1610.
Rowland, T. J., Sweet, M. E., Mestroni, L., & Taylor, M. R. (2016). Danon disease–dysregulation of autophagy in a multisystem disorder with cardiomyopathy. Journal of Cell Science, 129(11), 2135–2143.
Ng, K.-M., Mok, P. Y., Butler, A. W., Ho, J. C., Choi, S.-W., Lee, Y.-K., Lai, W.-H., Au, K.-W., Lau, Y.-M., & Wong, L.-Y. (2016). Amelioration of X-linked related autophagy failure in Danon disease with DNA methylation inhibitor. Circulation, 134(18), 1373–1389.
Yoshida, S., Nakanishi, C., Okada, H., Mori, M., Yokawa, J., Yoshimuta, T., Ohta, K., Konno, T., Fujino, N., & Kawashiri, M. A. (2018). Characteristics of induced pluripotent stem cells from clinically divergent female monozygotic twins with Danon disease. Journal of Molecular and Cellular Cardiology, 114, 234–242.
Chong, C.-M., Ke, M., Tan, Y., Huang, Z., Zhang, K., Ai, N., Ge, W., Qin, D., Lu, J.-H., & Su, H. (2018). Presenilin 1 deficiency suppresses autophagy in human neural stem cells through reducing γ-secretase-independent ERK/CREB signaling. Cell Death & Disease, 9(9), 879.
Martín-Maestro, P., Gargini, R., Sproul, A., García, A., Antón, E., Noggle, L. C., Arancio, O., Avila, J., & García-Escudero, V. (2017). Mitophagy failure in fibroblasts and iPSC-derived neurons of Alzheimer’s disease-associated presenilin 1 mutation. Frontiers in Molecular Neuroscience, 10, 291.
Lee, J. K., Jin, H. K., Park, M. H., Kim, B., Lee, P. H., Nakauchi, H., Carter, J. E., He, X., Schuchman, E. H., & Bae, J. (2014). Acid sphingomyelinase modulates the autophagic process by controlling lysosomal biogenesis in Alzheimer’s disease. Journal of Experimental Medicine, 211(8), 1551–1570.
Li, L., Roh, J. H., Chang, E. H., Lee, Y., Lee, S., Kim, M., Koh, W., Chang, J. W., Kim, H. J., & Nakanishi, M. (2018). iPSC modeling of presenilin1 mutation in alzheimer’s disease with cerebellar ataxia. Experimental Neurobiology, 27(5), 350–364.
Reddy, K., Cusack, C. L., Nnah, I. C., Khayati, K., Saqcena, C., Huynh, T. B., Noggle, S. A., Ballabio, A., & Dobrowolski, R. (2016). Dysregulation of nutrient sensing and CLEARance in presenilin deficiency. Cell Reports, 14(9), 2166–2179.
Verheyen, A., Diels, A., Dijkmans, J., Oyelami, T., Meneghello, G., Mertens, L., Versweyveld, S., Borgers, M., Buist, A., & Peeters, P. (2015). Using human iPSC-derived neurons to model TAU aggregation. PLoS One, 10(12), e0146127.
Khayati, K., Antikainen, H., Bonder, E. M., Weber, G. F., Kruger, W. D., Jakubowski, H., & Dobrowolski, R. (2016). The amino acid metabolite homocysteine activates mTORC1 to inhibit autophagy and form abnormal proteins in human neurons and mice. The FASEB Journal, 31(2), 598–609.
Kim, H., Calatayud, C., Guha, S., Fernández-Carasa, I., Berkowitz, L., Carballo-Carbajal, I., Ezquerra, M., Fernández-Santiago, R., Kapahi, P., & Raya, Á. (2018). The small GTPase RAC1/CED-10 is essential in maintaining dopaminergic neuron function and survival against α-synuclein-induced toxicity. Molecular Neurobiology, 55(9), 7533–7552.
Sánchez-Danés, A., Richaud‐Patin, Y., Carballo‐Carbajal, I., Jiménez‐Delgado, S., Caig, C., Mora, S., Di Guglielmo, C., Ezquerra, M., Patel, B., & Giralt, A. (2012). Disease‐specific phenotypes in dopamine neurons from human iPS‐based models of genetic and sporadic Parkinson’s disease. EMBO Molecular Medicine, 4(5), 380–395.
Ohta, E., Nihira, T., Uchino, A., Imaizumi, Y., Okada, Y., Akamatsu, W., Takahashi, K., Hayakawa, H., Nagai, M., & Ohyama, M. (2015). I2020T mutant LRRK2 iPSC-derived neurons in the Sagamihara family exhibit increased Tau phosphorylation through the AKT/GSK-3β signaling pathway. Human Molecular Genetics, 24(17), 4879–4900.
Borgs, L., Peyre, E., Alix, P., Hanon, K., Grobarczyk, B., Godin, J. D., Purnelle, A., Krusy, N., Maquet, P., & Lefebvre, P. (2016). Dopaminergic neurons differentiating from LRRK2 G2019S induced pluripotent stem cells show early neuritic branching defects. Scientific Reports, 6, 33377.
Heman-Ackah, S. M., Manzano, R., Hoozemans, J. J., Scheper, W., Flynn, R., Haerty, W., Cowley, S. A., Bassett, A. R., & Wood, M. J. (2017). Alpha-synuclein induces the unfolded protein response in Parkinson’s disease SNCA triplication iPSC-derived neurons. Human Molecular Genetics, 26(22), 4441–4450.
Fernandes, H. J., Hartfield, E. M., Christian, H. C., Emmanoulidou, E., Zheng, Y., Booth, H., Bogetofte, H., Lang, C., Ryan, B. J., & Sardi, S. P. (2016). ER stress and autophagic perturbations lead to elevated extracellular α-synuclein in GBA-N370S Parkinson’s iPSC-derived dopamine neurons. Stem Cell Reports, 6(3), 342–356.
Schöndorf, D. C., Aureli, M., McAllister, F. E., Hindley, C. J., Mayer, F., Schmid, B., Sardi, S. P., Valsecchi, M., Hoffmann, S., & Schwarz, L. K. (2014). iPSC-derived neurons from GBA1-associated Parkinson’s disease patients show autophagic defects and impaired calcium homeostasis. Nature Communications, 5, 4028.
Osaki, T., Uzel, S. G., & Kamm, R. D. (2018). Microphysiological 3D model of amyotrophic lateral sclerosis (ALS) from human iPS-derived muscle cells and optogenetic motor neurons. Science Advances, 4(10), eaat5847.
Imamura, K., Izumi, Y., Watanabe, A., Tsukita, K., Woltjen, K., Yamamoto, T., Hotta, A., Kondo, T., Kitaoka, S., & Ohta, A. (2017). The Src/c-Abl pathway is a potential therapeutic target in amyotrophic lateral sclerosis. Science Translational Medicine, 9(391), eaaf3962.
Madill, M., McDonagh, K., Ma, J., Vajda, A., McLoughlin, P., O’Brien, T., Hardiman, O., & Shen, S. (2017). Amyotrophic lateral sclerosis patient iPSC-derived astrocytes impair autophagy via non-cell autonomous mechanisms. Molecular Brain, 10(1), 22.
Lara Marrone, I. P., Casci, I., Japtok, J., Reinhardt, P., Janosch, A., Andree, C., Hyun, O. L., C. M., Koerner, E., Reinhardt, L., Cicardi, M. E., Hackmann, K., Klink, B., A. P., Alberti, S., Bickle, M., Hermann, A., Pandey, U. B., Hyman, A. A., & aJLS*. (2018). Isogenic FUS-eGFP iPSC reporter lines enable quantification of FUS stress granule pathology that is rescued by drugs inducing autophagy. Stem Cell Reports, 10, 1–15.
Rusmini, P., Cortese, K., Crippa, V., Cristofani, R., Cicardi, M. E., Ferrari, V., Vezzoli, G., Tedesco, B., Meroni, M., & Messi, E. (2019). Trehalose induces autophagy via lysosomal-mediated TFEB activation in models of motoneuron degeneration. Autophagy, 15(4), 631–651.
Chandrachud, U., Walker, M. W., Simas, A. M., Heetveld, S., Petcherski, A., Klein, M., Oh, H., Wolf, P., Zhao, W.-N., & Norton, S. (2015). Unbiased cell-based screening in a neuronal cell model of Batten disease highlights an interaction between Ca2 + homeostasis, autophagy, and CLN3 protein function. Journal of Biological Chemistry, 290(23), 14361–14380.
Lojewski, X., Staropoli, J. F., Biswas-Legrand, S., Simas, A. M., Haliw, L., Selig, M. K., Coppel, S. H., Goss, K. A., Petcherski, A., & Chandrachud, U. (2013). Human iPSC models of neuronal ceroid lipofuscinosis capture distinct effects of TPP1 and CLN3 mutations on the endocytic pathway. Human Molecular Genetics, 23(8), 2005–2022.
Boutry, M., Branchu, J., Lustremant, C., Pujol, C., Pernelle, J., Matusiak, R., Seyer, A., Poirel, M., Chu-Van, E., & Pierga, A. (2018). Inhibition of lysosome membrane recycling causes accumulation of gangliosides that contribute to neurodegeneration. Cell Reports, 23(13), 3813–3826.
Minegishi, Y., Iejima, D., Kobayashi, H., Chi, Z.-L., Kawase, K., Yamamoto, T., Seki, T., Yuasa, S., Fukuda, K., & Iwata, T. (2013). Enhanced optineurin E50K–TBK1 interaction evokes protein insolubility and initiates familial primary open-angle glaucoma. Human Molecular Genetics, 22(17), 3559–3567.
Tucker, B. A., Solivan-Timpe, F., Roos, B. R., Anfinson, K. R., Robin, A. L., Wiley, L. A., Mullins, R. F., & Fingert, J. H. (2014). Duplication of TBK1 stimulates autophagy in iPSC-derived retinal cells from a patient with normal tension glaucoma. Journal of Stem Cell Research & Therapy, 3(5), 161.
Hashem, S. I., Perry, C. N., Bauer, M., Han, S., Clegg, S. D., Ouyang, K., Deacon, D. C., Spinharney, M., Panopoulos, A. D., & Izpisua Belmonte, J. C. (2015). Brief report: oxidative stress mediates cardiomyocyte apoptosis in a human model of D anon disease and heart failure. Stem Cells, 33(7), 2343–2350.
Hashem, S. I., Murphy, A. N., Divakaruni, A. S., Klos, M. L., Nelson, B. C., Gault, E. C., Rowland, T. J., Perry, C. N., Gu, Y., & Dalton, N. D. (2017). Impaired mitophagy facilitates mitochondrial damage in Danon disease. Journal of Molecular and Cellular Cardiology, 108, 86–94.
Scheetz, T. E., Roos, B. R., Solivan-Timpe, F., Miller, K., DeLuca, A. P., Stone, E. M., Kwon, Y. H., Alward, W. L., Wang, K., & Fingert, J. H. (2016). SQSTM1 mutations and glaucoma. PLoS One, 11(6), e0156001.
Stone, E. M., Fingert, J. H., Alward, W. L., Nguyen, T. D., Polansky, J. R., Sunden, S. L., Nishimura, D., Clark, A. F., Nystuen, A., & Nichols, B. E. (1997). Identification of a gene that causes primary open angle glaucoma. Science, 275(5300), 668–670.
Rezaie, T., Child, A., Hitchings, R., Brice, G., Miller, L., Coca-Prados, M., Héon, E., Krupin, T., Ritch, R., & Kreutzer, D. (2002). Adult-onset primary open-angle glaucoma caused by mutations in optineurin. Science, 295(5557), 1077–1079.
Fingert, J. H., Robin, A. L., Stone, J. L., Roos, B. R., Davis, L. K., Scheetz, T. E., Bennett, S. R., Wassink, T. H., Kwon, Y. H., & Alward, W. L. (2011). Copy number variations on chromosome 12q14 in patients with normal tension glaucoma. Human Molecular Genetics, 20(12), 2482–2494.
Minegishi, Y., Nakayama, M., Iejima, D., Kawase, K., & Iwata, T. (2016). Significance of optineurin mutations in glaucoma and other diseases. Progress in Retinal and Eye Research, 55, 149–181.
Richter, B., Sliter, D. A., Herhaus, L., Stolz, A., Wang, C., Beli, P., Zaffagnini, G., Wild, P., Martens, S., & Wagner, S. A. (2016). Phosphorylation of OPTN by TBK1 enhances its binding to Ub chains and promotes selective autophagy of damaged mitochondria. Proceedings of the National Academy of Sciences, 113(15), 4039–4044.
Coen, K., Flannagan, R. S., Baron, S., Carraro-Lacroix, L. R., Wang, D., Vermeire, W., Michiels, C., Munck, S., Baert, V., & Sugita, S. (2012). Lysosomal calcium homeostasis defects, not proton pump defects, cause endo-lysosomal dysfunction in PSEN-deficient cells. Journal of Cell Biology, 198(1), 23–35.
Settembre, C., & Medina, D. L. (2015). TFEB and the CLEAR network (pp. 45–62). Amsterdam: Elsevier.
Greenamyre, J. T., & Hastings, T. G. (2004). Parkinson’s–divergent causes, convergent mechanisms. Science, 304(5674), 1120–1122.
Vos, T., Barber, R. M., Bell, B., Bertozzi-Villa, A., Biryukov, S., Bolliger, I., Charlson, F., Davis, A., Degenhardt, L., & Dicker, D. (2015). Global, regional, and national incidence, prevalence, and years lived with disability for 301 acute and chronic diseases and injuries in 188 countries, 1990–2013: a systematic analysis for the Global Burden of Disease Study 2013. The Lancet, 386(9995), 743–800.
Jungverdorben, J., Till, A., & Brüstle, O. (2017). Induced pluripotent stem cell-based modeling of neurodegenerative diseases: a focus on autophagy. Journal of Molecular Medicine, 95(7), 705–718.
Beevers, J. E., Caffrey, T. M., & Wade-Martins, R. (2013). Induced pluripotent stem cell (iPSC)-derived dopaminergic models of Parkinson’s disease. London: Portland Press Limited.
Manzoni, C., Mamais, A., Roosen, D. A., Dihanich, S., Soutar, M. P., Plun-Favreau, H., Bandopadhyay, R., Hardy, J., Tooze, S. A., & Cookson, M. R. (2016). mTOR independent regulation of macroautophagy by Leucine Rich Repeat Kinase 2 via Beclin-1. Scientific Reports, 6, 35106.
Ho, D. H., Kim, H., Nam, D., Sim, H., Kim, J., Kim, H. G., Son, I., & Seol, W. (2018). LRRK2 impairs autophagy by mediating phosphorylation of leucyl-tRNA synthetase. Cell Biochemistry and Function, 36(8), 431–442.
Kaushik, S., & Cuervo, A. M. (2009). Methods to monitor chaperone-mediated autophagy. Methods in Enzymology, 452, 297–324.
Wang, X., Yan, M. H., Fujioka, H., Liu, J., Wilson-Delfosse, A., Chen, S. G., Perry, G., Casadesus, G., & Zhu, X. (2012). LRRK2 regulates mitochondrial dynamics and function through direct interaction with DLP1. Human Molecular Genetics, 21(9), 1931–1944.
Stafa, K., Tsika, E., Moser, R., Musso, A., Glauser, L., Jones, A., Biskup, S., Xiong, Y., Bandopadhyay, R., & Dawson, V. L. (2013). Functional interaction of Parkinson’s disease-associated LRRK2 with members of the dynamin GTPase superfamily. Human Molecular Genetics, 23(8), 2055–2077.
Hsieh, C.-H., Shaltouki, A., Gonzalez, A. E., da Cruz, A. B., Burbulla, L. F., Lawrence, E. S., Schüle, B., Krainc, D., Palmer, T. D., & Wang, X. (2016). Functional impairment in miro degradation and mitophagy is a shared feature in familial and sporadic Parkinson’s disease. Cell Stem Cell, 19(6), 709–724.
Glater, E. E., Megeath, L. J., Stowers, R. S., & Schwarz, T. L. (2006). Axonal transport of mitochondria requires milton to recruit kinesin heavy chain and is light chain independent. The Journal of Cell Biology, 173(4), 545–557.
Hardiman, O., Van Den Berg, L. H., & Kiernan, M. C. (2011). Clinical diagnosis and management of amyotrophic lateral sclerosis. Nature Reviews Neurology, 7(11), 639.
Dimos, J. T., Rodolfa, K. T., Niakan, K. K., Weisenthal, L. M., Mitsumoto, H., Chung, W., Croft, G. F., Saphier, G., Leibel, R., & Goland, R. (2008). Induced pluripotent stem cells generated from patients with ALS can be differentiated into motor neurons. Science, 321(5893), 1218–1221.
Bunton-Stasyshyn, R. K., Saccon, R. A., Fratta, P., & Fisher, E. M. (2015). SOD1 function and its implications for amyotrophic lateral sclerosis pathology: new and renascent themes. The Neuroscientist, 21(5), 519–529.
Farg, M. A., Sundaramoorthy, V., Sultana, J. M., Yang, S., Atkinson, R. A., Levina, V., Halloran, M. A., Gleeson, P. A., Blair, I. P., & Soo, K. Y. (2014). C9ORF72, implicated in amytrophic lateral sclerosis and frontotemporal dementia, regulates endosomal trafficking. Human Molecular Genetics, 23(13), 3579–3595.
Yang, M., Liang, C., Swaminathan, K., Herrlinger, S., Lai, F., Shiekhattar, R., & Chen, J.-F. (2016). A C9ORF72/SMCR8-containing complex regulates ULK1 and plays a dual role in autophagy. Science Advances, 2(9), e1601167.
Blokhuis, A. M., Groen, E. J., Koppers, M., van den Berg, L. H., & Pasterkamp, R. J. (2013). Protein aggregation in amyotrophic lateral sclerosis. Acta Neuropathologica, 125(6), 777–794.
Boxer, A. L., & Miller, B. L. (2005). Clinical features of frontotemporal dementia. Alzheimer Disease & Associated Disorders, 19, S3–S6.
Guerreiro, R., Brás, J., & Hardy, J. (2015). SnapShot: Genetics of ALS and FTD. Cell, 160(4), 798–798. e791.
Almeida, S., Gascon, E., Tran, H., Chou, H. J., Gendron, T. F., DeGroot, S., Tapper, A. R., Sellier, C., Charlet-Berguerand, N., & Karydas, A. (2013). Modeling key pathological features of frontotemporal dementia with C9ORF72 repeat expansion in iPSC-derived human neurons. Acta Neuropathologica, 126(3), 385–399.
Ju, J.-S., Fuentealba, R. A., Miller, S. E., Jackson, E., Piwnica-Worms, D., Baloh, R. H., & Weihl, C. C. (2009). Valosin-containing protein (VCP) is required for autophagy and is disrupted in VCP disease. The Journal of Cell Biology, 187(6), 875–888.
Lee, J.-A., Beigneux, A., Ahmad, S. T., Young, S. G., & Gao, F.-B. (2007). ESCRT-III dysfunction causes autophagosome accumulation and neurodegeneration. Current Biology, 17(18), 1561–1567.
Cortes, C. J., Miranda, H. C., Frankowski, H., Batlevi, Y., Young, J. E., Le, A., Ivanov, N., Sopher, B. L., Carromeu, C., & Muotri, A. R. (2014). Polyglutamine-expanded androgen receptor interferes with TFEB to elicit autophagy defects in SBMA. Nature Neuroscience, 17(9), 1180.
Ranganathan, S., Harmison, G. G., Meyertholen, K., Pennuto, M., Burnett, B. G., & Fischbeck, K. H. (2008). Mitochondrial abnormalities in spinal and bulbar muscular atrophy. Human Molecular Genetics, 18(1), 27–42.
Sardiello, M., Palmieri, M., di Ronza, A., Medina, D. L., Valenza, M., Gennarino, V. A., Di Malta, C., Donaudy, F., Embrione, V., & Polishchuk, R. S. (2009). A gene network regulating lysosomal biogenesis and function. Science, 325(5939), 473–477.
Cartoni, R., & Martinou, J.-C. (2009). Role of mitofusin 2 mutations in the physiopathology of Charcot–Marie–Tooth disease type 2A. Experimental Neurology, 218(2), 268–273.
Rizzo, F., Ronchi, D., Salani, S., Nizzardo, M., Fortunato, F., Bordoni, A., Stuppia, G., Del Bo, R., Piga, D., & Fato, R. (2016). Selective mitochondrial depletion, apoptosis resistance, and increased mitophagy in human Charcot-Marie-Tooth 2A motor neurons. Human Molecular Genetics, 25(19), 4266–4281.
Rizzo, F., Ramirez, A., Compagnucci, C., Salani, S., Melzi, V., Bordoni, A., Fortunato, F., Niceforo, A., Bresolin, N., & Comi, G. P. (2017). Genome-wide RNA-seq of iPSC-derived motor neurons indicates selective cytoskeletal perturbation in Brown–Vialetto disease that is partially rescued by riboflavin. Scientific Reports, 7, 46271.
Compagnoni, G. M., Kleiner, G., Samarani, M., Aureli, M., Faustini, G., Bellucci, A., Ronchi, D., Bordoni, A., Garbellini, M., & Salani, S. (2018). Mitochondrial dysregulation and impaired autophagy in iPSC-derived dopaminergic neurons of multiple system atrophy. Stem Cell Reports, 11(5), 1185–1198.
Ou, Z., Luo, M., Niu, X., Chen, Y., Xie, Y., He, W., Song, B., Xian, Y., Fan, D., & OuYang, S. (2016). Autophagy promoted the degradation of mutant ATXN3 in neurally differentiated spinocerebellar Ataxia-3 human induced pluripotent stem cells. BioMed Research International, 2016, 6701793.
Lin, D.-S., Huang, Y.-W., Ho, C.-S., Hung, P.-L., Hsu, M.-H., Wang, T.-J., Wu, T.-Y., Lee, T.-H., Huang, Z.-D., & Chang, P.-C. (2019). Oxidative insults and mitochondrial DNA mutation promote enhanced autophagy and mitophagy compromising cell viability in pluripotent cell model of mitochondrial disease. Cells, 8(1), 65.
Hämäläinen, R. H., Manninen, T., Koivumäki, H., Kislin, M., Otonkoski, T., & Suomalainen, A. (2013). Tissue-and cell-type–specific manifestations of heteroplasmic mtDNA 3243A > G mutation in human induced pluripotent stem cell-derived disease model. Proceedings of the National Academy of Sciences, 110(38), E3622–E3630.
Cooper, H. M., Yang, Y., Ylikallio, E., Khairullin, R., Woldegebriel, R., Lin, K.-L., Euro, L., Palin, E., Wolf, A., & Trokovic, R. (2017). ATPase-deficient mitochondrial inner membrane protein ATAD3A disturbs mitochondrial dynamics in dominant hereditary spastic paraplegia. Human Molecular Genetics, 26(8), 1432–1443.
Sato, Y., Kobayashi, H., Higuchi, T., Shimada, Y., Ida, H., & Ohashi, T. (2016). TFEB overexpression promotes glycogen clearance of Pompe disease iPSC-derived skeletal muscle. Molecular Therapy-Methods & Clinical Development, 3, 16054.
Raval, K. K., Tao, R., White, B. E., De Lange, W. J., Koonce, C. H., Yu, J., Kishnani, P. S., Thomson, J. A., Mosher, D. F., & Ralphe, J. C. (2015). Pompe disease results in a Golgi-based glycosylation deficit in human induced pluripotent stem cell-derived cardiomyocytes. Journal of Biological Chemistry, 290(5), 3121–3136.
Higuchi, T., Kawagoe, S., Otsu, M., Shimada, Y., Kobayashi, H., Hirayama, R., Eto, K., Ida, H., Ohashi, T., & Nakauchi, H. (2014). The generation of induced pluripotent stem cells (iPSCs) from patients with infantile and late-onset types of Pompe disease and the effects of treatment with acid-α-glucosidase in Pompe’s iPSCs. Molecular Genetics and Metabolism, 112(1), 44–48.
Swaroop, M., Brooks, M. J., Gieser, L., Swaroop, A., & Zheng, W. (2018). Patient iPSC-derived neural stem cells exhibit phenotypes in concordance with the clinical severity of mucopolysaccharidosis I. Human Molecular Genetics, 27(20), 3612–3626.
Wong, K., Sidransky, E., Verma, A., Mixon, T., Sandberg, G. D., Wakefield, L. K., Morrison, A., Lwin, A., Colegial, C., & Allman, J. M. (2004). Neuropathology provides clues to the pathophysiology of Gaucher disease. Molecular Genetics and Metabolism, 82(3), 192–207.
Maetzel, D., Sarkar, S., Wang, H., Abi-Mosleh, L., Xu, P., Cheng, A. W., Gao, Q., Mitalipova, M., & Jaenisch, R. (2014). Genetic and chemical correction of cholesterol accumulation and impaired autophagy in hepatic and neural cells derived from Niemann-Pick Type C patient-specific iPS cells. Stem Cell Reports, 2(6), 866–880.
Lee, H., Lee, J. K., Park, M. H., Hong, Y. R., Marti, H. H., Kim, H., Okada, Y., Otsu, M., Seo, E.-J., & Park, J.-H. (2014). Pathological roles of the VEGF/SphK pathway in Niemann–Pick type C neurons. Nature Communications, 5, 5514.
Soga, M., Ishitsuka, Y., Hamasaki, M., Yoneda, K., Furuya, H., Matsuo, M., Ihn, H., Fusaki, N., Nakamura, K., & Nakagata, N. (2015). HPGCD outperforms HPBCD as a potential treatment for Niemann-Pick disease type C during disease modeling with iPS cells. Stem Cells, 33(4), 1075–1088.
Sarkar, S., Carroll, B., Buganim, Y., Maetzel, D., Ng, A. H., Cassady, J. P., Cohen, M. A., Chakraborty, S., Wang, H., & Spooner, E. (2013). Impaired autophagy in the lipid-storage disorder Niemann-Pick type C1 disease. Cell Reports, 5(5), 1302–1315.
Yoshida, T., Ozawa, Y., Suzuki, K., Yuki, K., Ohyama, M., Akamatsu, W., Matsuzaki, Y., Shimmura, S., Mitani, K., & Tsubota, K. (2014). The use of induced pluripotent stem cells to reveal pathogenic gene mutations and explore treatments for retinitis pigmentosa. Molecular Brain, 7(1), 45.
Sharma, T. P., Wiley, L. A., Whitmore, S. S., Anfinson, K. R., Cranston, C. M., Oppedal, D. J., Daggett, H. T., Mullins, R. F., Tucker, B. A., & Stone, E. M. (2017). Patient-specific induced pluripotent stem cells to evaluate the pathophysiology of TRNT1-associated Retinitis pigmentosa. Stem Cell Research, 21, 58–70.
Ramachandra Rao, S., Pfeffer, B. A., Más Gómez, N., Skelton, L. A., Keiko, U., Sparrow, J. R., Rowsam, A. M., Mitchell, C. H., & Fliesler, S. J. (2018). Compromised phagosome maturation underlies RPE pathology in cell culture and whole animal models of Smith-Lemli-Opitz Syndrome. Autophagy, 14(10), 1796–1817.
Yinyin Cao, J. X. J. W., Xiaojing Ma, F., Liu, Y., Li, W., Chen, L., Sun, Y., Wu, Y., Li, S., Li, J., & Huang, G. (2018). Generation of a urine-derived ips cell line from a patient with a ventricular septal defect and heart failure and the robust differentiation of these cells to cardiomyocytes via small molecules. Cellular Physiology and Biochemistry, 50, 538–551. https://doi.org/10.1159/000494167
Julian, L. M., Delaney, S. P., Wang, Y., Goldberg, A. A., Doré, C., Yockell-Lelièvre, J., Tam, R. Y., Giannikou, K., McMurray, F., & Shoichet, M. S. (2017). Human pluripotent stem cell–derived TSC2-haploinsufficient smooth muscle cells recapitulate features of lymphangioleiomyomatosis. Cancer Research, 77(20), 5491–5502.
Doulatov, S., Vo, L. T., Macari, E. R., Wahlster, L., Kinney, M. A., Taylor, A. M., Barragan, J., Gupta, M., McGrath, K., & Lee, H.-Y. (2017). Drug discovery for Diamond-Blackfan anemia using reprogrammed hematopoietic progenitors. Science Translational Medicine, 9(376), eaah5645.
Llewellyn, K. J., Nalbandian, A., Weiss, L. N., Chang, I., Yu, H., Khatib, B., Tan, B., Scarfone, V., & Kimonis, V. E. (2017). Myogenic differentiation of VCP disease-induced pluripotent stem cells: A novel platform for drug discovery. PLoS One, 12(6), e0176919.
Lu, J., Boheler, K. R., Jiang, L., Chan, C. W., Tse, W. W., Keung, W., Poon, E. N., Li, R. A., & Yao, X. (2018). Polycystin-2 plays an essential role in glucose starvation‐induced autophagy in human embryonic stem cell‐derived cardiomyocytes. Stem Cells, 36(4), 501–513.
Goto, Y., Nonaka, I., & Horai, S. (1990). A mutation in the tRNALeu (UUR) gene associated with the MELAS subgroup of mitochondrial encephalomyopathies. Nature, 348(6302), 651.
Tesson, C., Koht, J., & Stevanin, G. (2015). Delving into the complexity of hereditary spastic paraplegias: how unexpected phenotypes and inheritance modes are revolutionizing their nosology. Human Genetics, 134(6), 511–538.
Malicdan, M. C. V., & Nishino, I. (2012). Autophagy in lysosomal myopathies. Brain Pathology, 22(1), 82–88.
Footitt, E., Karimova, A., Burch, M., Yayeh, T., Dupre, T., Vuillaumier-Barrot, S., Chantret, I., Moore, S., Seta, N., & Grunewald, S (2009). Cardiomyopathy in the congenital disorders of glycosylation (CDG): a case of late presentation and literature review. Journal of Inherited Metabolic Disease: Official Journal of the Society for the Study of Inborn Errors of Metabolism, 32, 313–319.
Raben, N., Schreiner, C., Baum, R., Takikita, S., Xu, S., Xie, T., Myerowitz, R., Komatsu, M., Van Der Meulen, J. H., & Nagaraju, K. (2010). Suppression of autophagy permits successful enzyme replacement therapy in a lysosomal storage disorder—murine Pompe disease. Autophagy, 6(8), 1078–1089.
Lim, J.-A., Li, L., Kakhlon, O., Myerowitz, R., & Raben, N. (2015). Defects in calcium homeostasis and mitochondria can be reversed in Pompe disease. Autophagy, 11(2), 385–402.
Nascimbeni, A. C., Fanin, M., Masiero, E., Angelini, C., & Sandri, M. (2012). The role of autophagy in the pathogenesis of glycogen storage disease type II (GSDII). Cell Death and Differentiation, 19(10), 1698.
Raben, N., Ralston, E., Chien, Y.-H., Baum, R., Schreiner, C., Hwu, W.-L., Zaal, K. J., & Plotz, P. H. (2010). Differences in the predominance of lysosomal and autophagic pathologies between infants and adults with Pompe disease: implications for therapy. Molecular Genetics and Metabolism, 101(4), 324–331.
Huang, H.-P., Chen, P.-H., Hwu, W.-L., Chuang, C.-Y., Chien, Y.-H., Stone, L., Chien, C.-L., Li, L.-T., Chiang, S.-C., & Chen, H.-F. (2011). Human Pompe disease-induced pluripotent stem cells for pathogenesis modeling, drug testing and disease marker identification. Human Molecular Genetics, 20(24), 4851–4864.
Fukuda, T., Ewan, L., Bauer, M., Mattaliano, R. J., Zaal, K., Ralston, E., Plotz, P. H., & Raben, N. (2006). Dysfunction of endocytic and autophagic pathways in a lysosomal storage disease. Annals of Neurology: Official Journal of the American Neurological Association and the Child Neurology Society, 59(4), 700–708.
Wraith, J., & Jones, S. (2014). Mucopolysaccharidosis type I. Pediatric endocrinology reviews. PER, 12, 102–106.
Bendikov-Bar, I., & Horowitz, M. (2012). Gaucher disease paradigm: from ERAD to comorbidity. Human Mutation, 33(10), 1398–1407.
Awad, O., Sarkar, C., Panicker, L. M., Miller, D., Zeng, X., Sgambato, J. A., Lipinski, M. M., & Feldman, R. A. (2015). Altered TFEB-mediated lysosomal biogenesis in Gaucher disease iPSC-derived neuronal cells. Human Molecular Genetics, 24(20), 5775–5788.
Bellettato, C. M., & Scarpa, M. (2010). Pathophysiology of neuropathic lysosomal storage disorders. Journal of Inherited Metabolic Disease, 33(4), 347–362.
Daiger, S., Sullivan, L., & Bowne, S. (2013). Genes and mutations causing retinitis pigmentosa. Clinical Genetics, 84(2), 132–141.
Mendes, H. F., van der Spuy, J., Chapple, J. P., & Cheetham, M. E. (2005). Mechanisms of cell death in rhodopsin retinitis pigmentosa: implications for therapy. Trends in Molecular Medicine, 11(4), 177–185.
Hartong, D. T., Berson, E. L., & Dryja, T. P. (2006). Retinitis pigmentosa. The Lancet, 368(9549), 1795–1809.
Bietti, G. (1937). Ueber familiares Vorkommen von” Retinitis punkutata albescens“(verbunden mit” Dystrophia marginalis cristallinea corneae”), Glitzern des Glaskorpers und anderen degenerativen Augenveranderungen. Klin Monatsbl Augenheilkd, 99, 737–756.
Li, A., Jiao, X., Munier, F. L., Schorderet, D. F., Yao, W., Iwata, F., Hayakawa, M., Kanai, A., Chen, M. S., & Lewis, R. A. (2004). Bietti crystalline corneoretinal dystrophy is caused by mutations in the novel gene CYP4V2. The American Journal of Human Genetics, 74(5), 817–826.
Lee, J., Jiao, X., Hejtmancik, J. F., Kaiser-Kupfer, M., Gahl, W. A., Markello, T. C., Guo, J., & Chader, G. J. (2001). The metabolism of fatty acids in human Bietti crystalline dystrophy. Investigative Ophthalmology & Visual Science, 42(8), 1707–1714.
Caldwell, R. B. M. B. Freeze-fracture study of filipin binding in photoreceptor outer segments and pigment epithelium of dystrophic and normal retinas. The Journal of Comparative Neurology, 236(4), 523–537. https://doi.org/10.1002/cne.902360408.
Carstea, E. D., Morris, J. A., Coleman, K. G., Loftus, S. K., Zhang, D., Cummings, C., Gu, J., Rosenfeld, M. A., Pavan, W. J., & Krizman, D. B. (1997). Niemann-Pick C1 disease gene: homology to mediators of cholesterol homeostasis. Science, 277(5323), 228–231.
Héron, B., Valayannopoulos, V., Baruteau, J., Chabrol, B., Ogier, H., Latour, P., Dobbelaere, D., Eyer, D., Labarthe, F., & Maurey, H. (2012). Miglustat therapy in the French cohort of paediatric patients with Niemann-Pick disease type C. Orphanet Journal of Rare Diseases, 7(1), 36.
Sanchez-Wandelmer, J., & Reggiori, F. (2013). Amphisomes: out of the autophagosome shadow? The EMBO Journal, 32(24), 3116–3118.
Lieberman, A. P., Puertollano, R., Raben, N., Slaugenhaupt, S., Walkley, S. U., & Ballabio, A. (2012). Autophagy in lysosomal storage disorders. Autophagy, 8(5), 719–730.
Criollo, A., Maiuri, M. C., Tasdemir, E., Vitale, I., Fiebig, A., Andrews, D., Molgó, J., Diaz, J., Lavandero, S., & Harper, F. (2007). Regulation of autophagy by the inositol trisphosphate receptor. Cell Death and Differentiation, 14(5), 1029.
Smith, D. W., Lemli, L., & Opitz, J. M. (1964). A newly recognized syndromeof multiple congenital anomalies. The Journal of Pediatrics, 64(2), 210–217.
Tricarico, P., Crovella, S., & Celsi, F. (2015). Mevalonate pathway blockade, mitochondrial dysfunction and autophagy: a possible link. International Journal of Molecular Sciences, 16(7), 16067–16084.
Tu, C., Li, J., Jiang, X., Sheflin, L. G., Pfeffer, B. A., Behringer, M., Fliesler, S. J., & Qu, J. (2013). Ion-current-based proteomic profiling of the retina in a rat model of Smith-Lemli-Opitz syndrome. Molecular & Cellular Proteomics, 12(12), 3583–3598.
Kellner, U., Kellner, S., & Weinitz, S. (2008). Chloroquine retinopathy: lipofuscin-and melanin-related fundus autofluorescence, optical coherence tomography and multifocal electroretinography. Documenta Ophthalmologica, 116(2), 119–127.
Moyzis, A. G., Sadoshima, J., & Gustafsson, ÅB. (2015). Mending a broken heart: the role of mitophagy in cardioprotection. American Journal of Physiology-Heart and Circulatory Physiology, 308(3), H183–H192.
Galluzzi, L., Pietrocola, F., Levine, B., & Kroemer, G. (2014). Metabolic control of autophagy. Cell, 159(6), 1263–1276.
Warr, M. R., Binnewies, M., Flach, J., Reynaud, D., Garg, T., Malhotra, R., Debnath, J., & Passegué, E. (2013). FOXO3A directs a protective autophagy program in haematopoietic stem cells. Nature, 494(7437), 323.
Mortensen, M., & Simon, A. K. (2010). Nonredundant role of Atg7 in mitochondrial clearance during erythroid development. Autophagy, 6(3), 423–425.
Narla, A., & Ebert, B. L. (2010). Ribosomopathies: human disorders of ribosome dysfunction. Blood, 115(16), 3196–3205.
Draptchinskaia, N., Gustavsson, P., Andersson, B., Pettersson, M., Willig, T.-N., Dianzani, I., Ball, S., Tchernia, G., Klar, J., & Matsson, H. (1999). The gene encoding ribosomal protein S19 is mutated in Diamond-Blackfan anaemia. Nature Genetics, 21(2), 169.
Dutt, S., Narla, A., Lin, K., Mullally, A., Abayasekara, N., Megerdichian, C., Wilson, F. H., Currie, T., Khanna-Gupta, A., & Berliner, N. (2011). Haploinsufficiency for ribosomal protein genes causes selective activation of p53 in human erythroid progenitor cells. Blood, 117(9), 2567–2576.
Heijnen, H. F., van Wijk, R., Pereboom, T. C., Goos, Y. J., Seinen, C. W., van Oirschot, B. A., van Dooren, R., Gastou, M., Giles, R. H., & van Solinge, W. (2014). Ribosomal protein mutations induce autophagy through S6 kinase inhibition of the insulin pathway. PLoS Genetics, 10(5), e1004371.
Kimonis, V. E., Fulchiero, E., Vesa, J., & Watts, G. (2008). VCP disease associated with myopathy, Paget disease of bone and frontotemporal dementia: review of a unique disorder. Biochimica et Biophysica Acta (BBA)-Molecular Basis of Disease, 1782(12), 744–748.
Badadani, M., Nalbandian, A., Watts, G. D., Vesa, J., Kitazawa, M., Su, H., Tanaja, J., Dec, E., Wallace, D. C., & Mukherjee, J. (2010). VCP associated inclusion body myopathy and paget disease of bone knock-in mouse model exhibits tissue pathology typical of human disease. PLoS One, 5(10), e13183.
Nalbandian, A., Llewellyn, K. J., Badadani, M., Yin, H. Z., Nguyen, C., Katheria, V., Watts, G., Mukherjee, J., Vesa, J., & Caiozzo, V. (2013). A progressive translational mouse model of human valosin-containing protein disease: The VCPR155H/+ mouse. Muscle & Nerve, 47(2), 260–270.
Vesa, J., Su, H., Watts, G. D., Krause, S., Walter, M. C., Martin, B., Smith, C., Wallace, D. C., & Kimonis, V. E. (2009). Valosin containing protein associated inclusion body myopathy: abnormal vacuolization, autophagy and cell fusion in myoblasts. Neuromuscular Disorders, 19(11), 766–772.
Rangan, G., Tchan, M., Tong, A., Wong, A., & Nankivell, B. (2016). Recent advances in autosomal-dominant polycystic kidney disease. Internal Medicine Journal, 46(8), 883–892.
Zhu, P., Sieben, C. J., Xu, X., Harris, P. C., & Lin, X. (2017). Autophagy activators suppress cystogenesis in an autosomal dominant polycystic kidney disease model. Human Molecular Genetics, 26(1), 158–172.
Rowe, I., Chiaravalli, M., Mannella, V., Ulisse, V., Quilici, G., Pema, M., Song, X. W., Xu, H., Mari, S., & Qian, F. (2013). Defective glucose metabolism in polycystic kidney disease identifies a new therapeutic strategy. Nature Medicine, 19(4), 488.
Chun, Y. S., Chaudhari, P., & Jang, Y.-Y. (2010). Applications of patient-specific induced pluripotent stem cells; focused on disease modeling, drug screening and therapeutic potentials for liver disease. International Journal of Biological Sciences, 6(7), 796.
Mathew, R., Karantza-Wadsworth, V., & White, E. (2007). Role of autophagy in cancer. Nature Reviews Cancer, 7(12), 961.
Acknowledgements
The author would like to thank Dr. Baharvand and Dr. Ghavami for their kind help and support. This study was supported by a grant from Royan Institute.
Author information
Authors and Affiliations
Corresponding authors
Ethics declarations
Conflicts of Interest
The authors declare that they have no conflicts of interest.
Additional information
Publisher’s Note
Springer Nature remains neutral with regard to jurisdictional claims in published maps and institutional affiliations.
Rights and permissions
About this article
Cite this article
Kolahdouzmohammadi, M., Totonchi, M. & Pahlavan, S. The Role of iPSC Modeling Toward Projection of Autophagy Pathway in Disease Pathogenesis: Leader or Follower. Stem Cell Rev and Rep 17, 539–561 (2021). https://doi.org/10.1007/s12015-020-10077-8
Accepted:
Published:
Issue Date:
DOI: https://doi.org/10.1007/s12015-020-10077-8