Abstract
The manganese silicate and calcium ferrite commonly generated during the treatment process of manganese ore results in difficult separation of manganese and iron. In this study, the migration behaviors of ferromanganese spinel and gangue compounds in a microwave field were explored by examining the corresponding phase and microstructural evolutions. Different from the traditional process, the interface reactions depend on the diffusion of Mn2+ and Fe2+ towards the surface of the gangue particles, owing to the microwave absorption ability of ferrite being better than that of calcium oxide. Compared with manganese, iron more easily combined with silicon oxide to form silicates. CaO induced the lattice transformation of the ferromanganese spinel to calcium manganese ferrite. The migration rate of manganese was faster than that of iron in the microwave field. The appropriate mass ratio (m(CaO)/m(SiO2)) reduced the negative impact of gangue components on the reduction process of ferromanganese.












Similar content being viewed by others
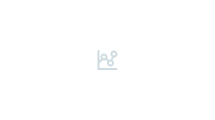
References
International Manganese Institute, 2008–2016. IMnI Annual Review. (2020) www.manganese.org, (Accessed 16 Nov 2022).
B. Liu, Y. Zhang, M. Lu, Z. Su, G. Li, and T. Jiang, Miner. Eng. 131, 286 https://doi.org/10.1016/j.mineng.2018.11.016 (2019).
T. Kodama, M. Ookubo, S. Miura, and Y. Kitayama, Mater. Res. Bull. 31, 1501 https://doi.org/10.1016/S0025-5408(96)00146-8 (1996).
V.A.M. Brabers, J. Phys. Chem. Solids. 32, 2181 https://doi.org/10.1016/S0022-3697(71)80396-7 (1971).
Z. Cheng, G. Zhu, and Y. Zhao, Hydrometallurgy 96, 176 https://doi.org/10.1016/j.hydromet.2008.08.004 (2009).
P. Ding, Q. Liu, and W. Pang, Appl. Mech. Mater. 280, 4431 https://doi.org/10.4028/www.scientific.net/AMM.380-384.4431 (2013).
Y. Gao, M. Olivas-Martinez, H.Y. Sohn, H.G. Kim, and C.W. Kim, Metall. Mater. Trans. B 43, 1465 https://doi.org/10.1007/s11663-012-9731-6 (2012).
H. Zhang, J. Li, A. Xu, Q. Yang, D. He, and N. Tian, J. Iron Steel Res. Int. 21, 427 https://doi.org/10.1016/S1006-706X(14)60066-2 (2014).
S.K. Tripathy, P.K. Banerjee, and N. Suresh, Int. J. Min. Met. Mater. 22, 661 https://doi.org/10.1007/s12613-015-1120-0 (2015).
G. Senanayake, Hydrometallurgy 73, 215 https://doi.org/10.1016/j.hydromet.2003.10.010 (2004).
B. Ghafarizadeh, F. Rashchi, and E. Vahidi, Miner. Eng. 24, 174 https://doi.org/10.1016/j.mineng.2010.11.003 (2011).
W. Zhang and C.Y. Cheng, Hydrometallurgy 89, 137 https://doi.org/10.1016/j.hydromet.2007.08.010 (2007).
C. Li, H. Zhong, S. Wang, J. Xue, F. Wu, and Z. Zhang, Trans. Nonferrous Met. Soc. China 25, 1677 https://doi.org/10.1016/s1003-6326(15)63772-4 (2015).
Q. Ye, Z. Peng, G. Li, Y. Liu, M. Liu, L. Ye, L. Wang, M. Rao, T. Jiang, and B. Zhao, Powder Technol. 377, 20–28 https://doi.org/10.1016/j.powtec.2020.08.070 (2021).
L. Gao, Z. Liu, M. Chu, R. Wang, Z. Wang, and C. Feng, Sep. Sci. Technol. 54, 195 https://doi.org/10.1080/01496395.2018.1504795 (2018).
Z. Cai, Y. Feng, H. Li, X. Liu, and Z. Yang, JOM 64, 1296 (2012).
W. Ye, Y. Li, L. Kong, M. Ren, and Q. Han, Trans. Nonferrous Met. Soc. China 23, 2083 https://doi.org/10.1016/s1003-6326(13)62838-1 (2013).
N.J. Welham, Int. J. Miner. Process. 67, 187 https://doi.org/10.1016/S0301-7516(02)00045-5 (2002).
B. Liu, Y. Zhang, Z. Su, M. Lu, Z. Peng, G. Li, and T. Jiang, Powder Technol. 313, 201–209 (2017).
R. Kononov, O. Ostrovski, and S. Ganguly, Metall. Mater. Trans. B 39, 662 https://doi.org/10.1007/s11663-008-9191-1 (2008).
X. Li, K. Tang, and M. Tangstad, Minerals 10, 97 https://doi.org/10.3390/min10020097 (2020).
G. Akdogan and R.H. Eric, Metall. Mater. Trans. B 26, 13 https://doi.org/10.1007/BF02648973 (1995).
J. Song, G. Zhu, Y. Zhao, and P. Zhang, Acta Metall. Sin. (English) 23, 223 (2010).
Y. Zhao, G. Zhu, and Z. Cheng, Hydrometallurgy 105, 96 https://doi.org/10.1016/j.hydromet.2010.08.004 (2010).
H. Zhang, G. Zhu, H. Yan, T. Li, and Y. Zhao, Metall. Mater. Trans. B 44, 889 https://doi.org/10.1007/s11663-013-9835-7 (2013).
Y. Zhang, M. Du, B. Liu, Z. Su, G. Li, and T. Jiang, Sep. Sci. Technol. 52, 1321 https://doi.org/10.1080/01496395.2017.1284864 (2017).
G. Chen, L. Li, C. Tao, Z. Liu, N. Chen, and J. Peng, J. Alloys Compd. 657, 515 https://doi.org/10.1016/j.jallcom.2015.10.147 (2016).
Q. Ye, Z. Peng, G. Li, J. Lee, Y. Liu, M. Liu, L. Wang, M. Rao, Y. Zhang, and T. Jiang, ACS Sustain. Chem. Eng. 7, 9515 https://doi.org/10.1021/acssuschemeng.9b00959 (2019).
J. Chen, P. Tian, X. Song, N. Li, and J. Zhou, J. Iron Steel Res. Int. 17, 13 https://doi.org/10.1016/S1006-706X(10)60066-0 (2010).
Q. Ye, Z. Peng, G. Li, Y. Liu, M. Liu, L. Ye, L. Wang, M. Rao, Y. Zhang, and T. Jiang, J. Clean. Prod. 286, 124919 https://doi.org/10.1016/j.jclepro.2020.124919 (2021).
Acknowledgements
This work was partially supported by the National Natural Science Foundation of China (No. 52204282), and the college student innovations special projects of Wuhan University of Science and Technology (No. 22z118).
Author information
Authors and Affiliations
Corresponding author
Ethics declarations
Conflict of interest
On behalf of all authors, the corresponding author states that there is no conflict of interest.
Additional information
Publisher's Note
Springer Nature remains neutral with regard to jurisdictional claims in published maps and institutional affiliations.
Rights and permissions
Springer Nature or its licensor (e.g. a society or other partner) holds exclusive rights to this article under a publishing agreement with the author(s) or other rightsholder(s); author self-archiving of the accepted manuscript version of this article is solely governed by the terms of such publishing agreement and applicable law.
About this article
Cite this article
Ye, Q., Jiang, Y., Lv, J. et al. Phase Evolution Between Ferromanganese Spinel and Gangue Components with Microwave Induction in Reducing Atmosphere. JOM 75, 4341–4349 (2023). https://doi.org/10.1007/s11837-023-06039-3
Received:
Accepted:
Published:
Issue Date:
DOI: https://doi.org/10.1007/s11837-023-06039-3