Abstract
Despite being a promising photoanode material for water splitting, WO3 has low conductivity, high onset potential, and sluggish water oxidation kinetics. In this study, we designed Ti-doped WO3 nanoplate arrays on fluoride-doped tin oxide by a seed-free hydrothermal method, and the effects of doping on the photoelectrochemical performance were investigated. The optimal Ti-doped WO3 electrode achieved a photocurrent density of 0.53 mA/cm2 at 0.6 V (vs Ag/AgCl), 110% higher than that of pure WO3 nanoplate arrays. Moreover, a significant cathodic shift in the onset potential was observed after doping. X-ray photoelectron spectroscopy valence band and ultraviolet — visible spectra revealed that the band positions of Ti-doped WO3 photoanodes moved upward, yielding a lower onset potential. Furthermore, electrochemical impedance spectroscopy measurements revealed that the conductivities of the WO3 photoanodes improved after doping, because of the rapid separation of photo-generated charge carriers. Thus, we report a new design route toward efficient and low-cost photoanodes for photoelectrochemical applications.
摘要
氧化钨(WO3)是一种重要的光电化学分解水半导体,具有可见光响应好、无毒、稳定性好等优 点,但低的电导率、高的起始电位以及缓慢的水氧化动力学等缺点也限制了其进一步应用。本文采用 无晶种水热法在导电玻璃(FTO)上设计了一种钛掺杂的WO3纳米片阵列,并研究了Ti 掺杂浓度对光电 化学分解水性能的影响。结果表明,在0.6 V(vs Ag/AgCl)的偏压下,Ti 掺杂WO3光电流密度最高,可 达0.53 mA/cm2,比纯WO3纳米片阵列的高110%。同时,Ti 掺杂WO3光电极的起始电位负移。X射线 光电子能谱价带谱(XPS-VB)和紫外-可见吸收(UV-Vis)光谱显示,Ti 掺杂WO3光阳极的能带位置向上移 动,导致了起始电位的负移。此外,电化学阻抗谱测试(EIS)表明,Ti 掺杂WO3光阳极的导电性显著增 强,引起光生载流子的快速分离。研究成果有望提供一种高效、低成本光阳极的设计路线。
Similar content being viewed by others
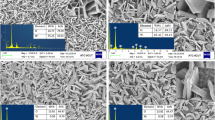
Data availability statements
The datasets generated during and/or analyzed during the current study are available from the corresponding author on reasonable request.
References
ZHOU Min, XU Yang, LEI Yong. Heterogeneous nanostructure array for electrochemical energy conversion and storage [J]. Nano Today, 2018, 20: 33–57. DOI: https://doi.org/10.1016/j.nantod.2018.04.002.
WANG Ya-bin, MA Hui, GUO Jing-jing, et al. TiO2−x nanoparticles dispersed in center-radial channels of dendritic mesoporous silica nanospheres (DMSNs) as novelly structured photocatalysts [J]. Journal of Materials Science, 2021, 56(26): 14659–14671. DOI: https://doi.org/10.1007/s10853-021-06049-z.
MONTOYA J H, SEITZ L C, CHAKTHRANONT P, et al. Materials for solar fuels and chemicals [J]. Nature Materials, 2017, 16(1): 70–81. DOI: https://doi.org/10.1038/nmat4778.
JIANG Chao-ran, MONIZ S J A, WANG Ai-qin, et al. Photoelectrochemical devices for solar water splitting-materials and challenges [J]. Chemical Society Reviews, 2017, 46(15): 4645–4660. DOI: https://doi.org/10.1039/c6cs00306k.
QIU Yong-cai, PAN Zheng-hui, CHEN Hai-ning, et al. Current progress in developing metal oxide nanoarrays-based photoanodes for photoelectrochemical water splitting [J]. Science Bulletin, 2019, 64(18): 1348–1380. DOI: https://doi.org/10.1016/j.scib.2019.07.017.
FUJISHIMA A, HONDA K. Electrochemical photolysis of water at a semiconductor electrode [J]. Nature, 1972, 238(5385): 37–38. https://doi.org/10.1038/238037a0.
CAO Da-peng, LUO Wen-jun, FENG Jian-yong, et al. Cathodic shift of onset potential for water oxidation on a Ti4+ doped Fe2O3 photoanode by suppressing the back reaction [J]. Energy Environ Sci, 2014, 7(2): 752–759. DOI: https://doi.org/10.1039/c3ee42722f.
KLAHR B, GIMENEZ S, FABREGAT-SANTIAGO F, et al. Photoelectrochemical and impedance spectroscopic investigation of water oxidation with “Co-Pi”-coated hematite electrodes [J]. Journal of the American Chemical Society, 2012, 134(40): 16693–16700. DOI: https://doi.org/10.1021/ja306427f.
MAZZARO R, BOSCOLO B S, NATALI M, et al. Hematite nanostructures: An old material for a new story. Simultaneous photoelectrochemical oxidation of benzylamine and hydrogen production through Ti doping [J]. Nano Energy, 2019, 61: 36–46. DOI: https://doi.org/10.1016/j.nanoen.2019.04.013.
CHEN Dong, LIU Zhi-feng, ZHANG Shao-ce. Enhanced PEC performance of hematite photoanode coupled with bimetallic oxyhydroxide NiFeOOH through a simple electroless method [J]. Applied Catalysis B: Environmental, 2020, 265: 118580. DOI: https://doi.org/10.1016/j.apcatb.2019.118580.
ZHONG D K, CHOI S, GAMELIN D R. Near-complete suppression of surface recombination in solar photoelectrolysis by “Co-Pi” catalyst-modified W: BiVO4 [J]. Journal of the American Chemical Society, 2011, 133(45): 18370–18377. DOI: https://doi.org/10.1021/ja207348x.
KIM T W, CHOI K S. Nanoporous BiVO4 photoanodes with dual-layer oxygen evolution catalysts for solar water splitting [J]. Science, 2014, 343(6174): 990–994. DOI: https://doi.org/10.1126/science.1246913.
SELIM S, PASTOR E, GARCÍA-TECEDOR M, et al. Impact of oxygen vacancy occupancy on charge carrier dynamics in BiVO4 photoanodes [J]. Journal of the American Chemical Society, 2019, 141(47): 18791–18798. DOI: https://doi.org/10.1021/jacs.9b09056.
LIU Jia-li, YANG Qiang, LIU Ji-kai, et al. Enhanced photoelectrochemical water oxidation of WO3/R-CoO and WO3/B-CoO photoanodes with a type II heterojunction [J]. Journal of Materials Science, 2021, 56(13): 8079–8090. DOI: https://doi.org/10.1007/s10853-020-05754-5.
WANG Yi-dan, TIAN Wei, CHEN Cheng, et al. Tungsten trioxide nanostructures for photoelectrochemical water splitting: Material engineering and charge carrier dynamic manipulation [J]. Advanced Functional Materials, 2019, 29(23): 1809036. DOI: https://doi.org/10.1002/adfm.201809036.
ZHANG Peng, WANG Tuo, GONG Jin-long. Passivation of surface states by ALD-grown TiO2 overlayers on Ta3N5 anodes for photoelectrochemical water oxidation [J]. Chemical Communications (Cambridge, England), 2016, 52(57): 8806–8809. DOI: https://doi.org/10.1039/c6cc03411j.
NARKEVICIUTE I, CHAKTHRANONT P, MACKUS A J M, et al. Tandem core-shell Si-Ta3N5 photoanodes for photoelectrochemical water splitting [J]. Nano Letters, 2016, 16(12): 7565–7572. DOI: https://doi.org/10.1021/acs.nanolett.6b03408.
SAHARA G, KUMAGAI H, MAEDA K, et al. Photoelectrochemical reduction of CO2 coupled to water oxidation using a photocathode with a Ru(II)-Re(I) complex photocatalyst and a CoOx/TaON photoanode [J]. Journal of the American Chemical Society, 2016, 138(42): 14152–14158. DOI: https://doi.org/10.1021/jacs.6b09212.
ZHAN Fa-qi, WANG Ke-ke, YANG Xue-tao, et al. Epitaxial growth of Heteropolyacid-WO3 vertical heterostructures with photo-induced charge modulation for enhanced water oxidation [J]. Electrochimica Acta, 2019, 306: 96–105. DOI: https://doi.org/10.1016/j.electacta.2019.03.113.
ZHANG Tao, WANG Lu, SU Jin-zhan, et al. Branched tungsten oxide nanorod arrays synthesized by controlled phase transformation for solar water oxidation [J]. Chem Cat Chem, 2016, 8(12): 2119–2127. DOI: https://doi.org/10.1002/cctc.201600267.
HILL J C, CHOI K S. Effect of electrolytes on the selectivity and stability of n-type WO3 photoelectrodes for use in solar water oxidation [J]. The Journal of Physical Chemistry C, 2012, 116(14): 7612–7620. DOI: https://doi.org/10.1021/jp209909b.
JIAO Zhi-hui, WANG Jin-min, KE Lin, et al. Morphology-tailored synthesis of tungsten trioxide (hydrate) thin films and their photocatalytic properties [J]. ACS Applied Materials & Interfaces, 2011, 3(2): 229–236. DOI: https://doi.org/10.1021/am100875z.
ZHANG Ning, LI Xi-yu, YE Hua-cheng, et al. Oxide defect engineering enables to couple solar energy into oxygen activation [J]. Journal of the American Chemical Society, 2016, 138(28): 8928–8935. DOI: https://doi.org/10.1021/jacs.6b04629.
HAO Zhi-chao, GUO Zhen-gang, RUAN Meng-nan, et al. Multifunctional WO3/NiCo2O4 heterojunction with extensively exposed bimetallic Ni/Co redox reaction sites for efficient photoelectrochemical water splitting [J]. Chem Cat Chem, 2021, 13(1): 271–280. DOI: https://doi.org/10.1002/cctc.202001298.
MA Ming, ZHANG Kan, LI Ping, et al. Dual oxygen and tungsten vacancies on a WO3 photoanode for enhanced water oxidation [J]. Angewandte Chemie International Edition, 2016, 55(39): 11819–11823. DOI: https://doi.org/10.1002/anie.201605247.
LIU Ya, ZHAO Liang, SU Jin-zhan, et al. Fabrication and properties of a branched (NH4)xWO3 nanowire array film and a porous WO3 nanorod array film [J]. ACS Applied Materials & Interfaces, 2015, 7(6): 3532–3538. DOI: https://doi.org/10.1021/am507230t.
JADWISZCZAK M, JAKUBOW-PIOTROWSKA K, KEDZIERZAWSKI P, et al. Highly efficient sunlight-driven seawater splitting in a photoelectrochemical cell with chlorine evolved at nanostructured WO3 photoanode and hydrogen stored as hydride within metallic cathode [J]. Advanced Energy Materials, 2020, 10(3): 1903213. DOI: https://doi.org/10.1002/aenm.201903213.
FAN Xiao-li, GAO Bin, WANG Tao, et al. Layered double hydroxide modified WO3 nanorod arrays for enhanced photoelectrochemical water splitting [J]. Applied Catalysis A: General, 2016, 528: 52–58. DOI: https://doi.org/10.1016/j.apcata.2016.09.014.
CAO Xiao-hu, XU Chun-jiang, MA Jia-rui, et al. Enhanced photoelectrochemical performance of \({\rm{WO}}_3^ - \) based composite photoanode coupled with carbon quantum dots and NiFe layered double hydroxide [J]. Chem Sus Chem, 2019, 12(20): 4685–4692. DOI: https://doi.org/10.1002/cssc.201901803.
ZHANG Lu, YANG Meng-fan, LUO Zhi-shan, et al. Photodeposited CoOx as highly active phases to boost water oxidation on BiVO4/WO3 photoanode [J]. International Journal of Hydrogen Energy, 2019, 44(47): 25652–25661. DOI: https://doi.org/10.1016/j.ijhydene.2019.08.040.
BAI Shou-li, YANG Xiao-jun, LIU Cheng-yao, et al. An integrating photoanode of WO3/Fe2O3 heterojunction decorated with NiFe-LDH to improve PEC water splitting efficiency [J]. ACS Sustainable Chemistry & Engineering, 2018, 6(10): 12906–12913. DOI: https://doi.org/10.1021/acssuschemeng.8b02267.
RETTIE A J E, KLAVETTER K C, LIN J F, et al. Improved visible light harvesting of WO3 by incorporation of sulfur or iodine: A tale of two impurities [J]. Chemistry of Materials, 2014, 26(4): 1670–1677. DOI: https://doi.org/10.1021/cm403969r.
LIU Yang, LI Jie, LI Wen-zhang, et al. Enhancement of the photoelectrochemical performance of WO3 vertical arrays film for solar water splitting by gadolinium doping [J]. The Journal of Physical Chemistry C, 2015, 119(27): 14834–14842. DOI: https://doi.org/10.1021/acs.jpcc.5b00966.
WANG Xiao-guang, SUN Ming-hui, MURUGANANTHAN M, et al. Electrochemically self-doped WO3/TiO2 nanotubes for photocatalytic degradation of volatile organic compounds [J]. Applied Catalysis B: Environmental, 2020, 260: 118205. DOI: https://doi.org/10.1016/j.apcatb.2019.118205.
SHIN S, HAN H S, KIM J S, et al. A tree-like nanoporous WO3 photoanode with enhanced charge transport efficiency for photoelectrochemical water oxidation [J]. Journal of Materials Chemistry A, 2015, 3(24): 12920–12926. DOI: https://doi.org/10.1039/c5ta00823a.
NAYAK A K, SOHN Y, PRADHAN D. Facile green synthesis of WO3·H2O nanoplates and WO3 nanowires with enhanced photoelectrochemical performance [J]. Crystal Growth & Design, 2017, 17(9): 4949–4957. DOI: https://doi.org/10.1021/acs.cgd.7b00886.
KALANUR S S, YOO I H, CHO I S, et al. Niobium incorporated WO3 nanotriangles: Band edge insights and improved photoelectrochemical water splitting activity [J]. Ceramics International, 2019, 45(7): 8157–8165. DOI: https://doi.org/10.1016/j.ceramint.2019.01.117.
ZHANG Teng, ZHU Zong-long, CHEN Hai-ning, et al. Iron-doping-enhanced photoelectrochemical water splitting performance of nanostructured WO3: A combined experimental and theoretical study [J]. Nanoscale, 2015, 7(7): 2933–2940. DOI: https://doi.org/10.1039/c4nr07024k.
KALANUR S S, YOO I H, EOM K, et al. Enhancement of photoelectrochemical water splitting response of WO3 by Means of Bi doping [J]. Journal of Catalysis, 2018, 357: 127–137. DOI: https://doi.org/10.1016/j.jcat.2017.11.012.
KAKO T, MENG Xian-guang, YE Jin-hua. Enhancement of photocatalytic activity for WO3 by simple NaOH loading [J]. Applied Catalysis A: General, 2014, 488: 183–188. DOI: https://doi.org/10.1016/j.apcata.2014.09.046.
WANG Feng-gong, di VALENTIN C, PACCHIONI G. Doping of WO3 for photocatalytic water splitting: Hints from density functional theory [J]. The Journal of Physical Chemistry C, 2012, 116(16): 8901–8909. DOI: https://doi.org/10.1021/jp300867j.
ZHANG Xue-liang, WANG Xin, WANG De-fa, et al. Conformal BiVO4-layer/WO3-nanoplate-array heterojunction photoanode modified with cobalt phosphate cocatalyst for significantly enhanced photoelectrochemical performances [J]. ACS Applied Materials & Interfaces, 2019, 11(6): 5623–5631. DOI: https://doi.org/10.1021/acsami.8b05477.
SU Jin-zhan, FENG Xin-jian, SLOPPY J D, et al. Vertically aligned WO3 nanowire arrays grown directly on transparent conducting oxide coated glass: Synthesis and photoelectrochemical properties [J]. Nano Letters, 2011, 11(1): 203–208. DOI: https://doi.org/10.1021/nl1034573.
YANG Jiao, LI Wen-zhang, LI Jie, et al. Hydrothermal synthesis and photoelectrochemical properties of vertically aligned tungsten trioxide (hydrate) plate-like arrays fabricated directly on FTO substrates [J]. Journal of Materials Chemistry, 2012, 22(34): 17744. DOI: https://doi.org/10.1039/c2jm33199c.
GAO Hong-qing, ZHANG Peng, HU Jun-hua, et al. One-dimensional Z-scheme TiO2/WO3/Pt heterostructures for enhanced hydrogen generation [J]. Applied Surface Science, 2017, 391: 211–217. DOI: https://doi.org/10.1016/j.apsusc.2016.06.170.
ZHAO Jiang-tao, ZHANG Peng, FAN Jia-jie, et al. Constructing 2D layered MoS2 nanosheets-modified Z-scheme TiO2/WO3 nanofibers ternary nanojunction with enhanced photocatalytic activity [J]. Applied Surface Science, 2018, 430: 466–474. DOI: https://doi.org/10.1016/j.apsusc.2017.06.308.
UPADHYAY S B, MISHRA R K, SAHAY P P. Structural and alcohol response characteristics of Sn-doped WO3 nanosheets [J]. Sensors and Actuators B: Chemical, 2014, 193: 19–27. DOI: https://doi.org/10.1016/j.snb.2013.11.049.
FENG Cheng-xin, WANG Shao-zhen, GENG Bao-you. Ti(IV) doped WO3 nanocuboids: Fabrication and enhanced visible-light-driven photocatalytic performance [J]. Nanoscale, 2011, 3(9): 3695–3699. DOI: https://doi.org/10.1039/c1nr10460h.
YEH T F, TENG C Y, CHEN S J, et al. Nitrogen-doped graphene oxide quantum dots as photocatalysts for overall water-splitting under visible light illumination [J]. Advanced Materials, 2014, 26(20): 3297–3303. DOI: https://doi.org/10.1002/adma.201305299.
SHEN Shao-hua, ZHOU Ji-gang, DONG Chung-li, et al. Surface engineered doping of hematite nanorod arrays for improved photoelectrochemical water splitting [J]. Scientific Reports, 2014, 4: 6627. DOI: https://doi.org/10.1038/srep06627.
Author information
Authors and Affiliations
Contributions
TANG Ya-qin conducted partial experiments, performed data analysis and edited the draft of the manuscript. JIANG Di, WANG Huan, ZHENG Hong-ye, REN Lu-jun conducted partial experiments. ZHANG Xue-liang provided the concept, conducted partial experiments and wrote the first draft of the manuscript. WEI Kui-xian, MA Wen-hui, LUO Da-jun and LIU Yi-ke performed data analysis and conducted the literature review.
Corresponding authors
Additional information
Conflict of interest
The authors declare that they have no known competing financial interests or personal relationship that could have appeared to influence the work reported in this paper.
Foundation item: Project(Qian Jiao He KY Zi [2021]257) supported provided by the Natural Science Research Project of Education Department of Guizhou Province, China; Project(GZSQCC2019003) supported by the High-level Innovative Talent Cultivation Project of Guizhou Province, China; Projects(GZLGXM-01, GZLGXM-08) supported by the Academic New Seedling Cultivation and Innovation Exploration Project of Guizhou Institute of Technology, China
Rights and permissions
About this article
Cite this article
Tang, Yq., Jiang, D., Wang, H. et al. Band gap modulation of nanostructured WO3 nanoplate film by Ti doping for enhanced photoelectrochemical performance. J. Cent. South Univ. 29, 2968–2979 (2022). https://doi.org/10.1007/s11771-022-5125-3
Received:
Accepted:
Published:
Issue Date:
DOI: https://doi.org/10.1007/s11771-022-5125-3