Abstract
In this article, we focus on using the multiexciton generation (MEG) effect of quantum dot (QD) to realize quantum cutting of high-energy photons which will give rise to a remarkable increase of total photon number. To avoid the complicated solving of Schrödinger equation, we take approximations and develop a method for fast evaluating the quantum efficiency of MEG process. On this basis, we calculate the detailed balance limit of efficiency of Si single-junction solar cell with Si QD-doped glass placed on top of it as a spectral converter layer. It shows that the efficiency will reach 36 % which is 6 % higher than that without the converter layer. We have also explored the influence of QD radius, QD-doping density, QD, and host material, device working temperature on the efficiency.





Similar content being viewed by others
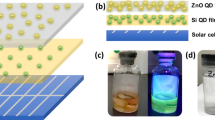
References
Wei G, Forrest SR (2007) Intermediate-band solar cells employing quantum dots embedded in an energy fence barrier. Nano Lett 7:218–222
Marti A, Lopez N, Antolin E et al (2007) Emitter degradation in quantum dot intermediate band solar cells. Appl Phys Lett 90:233510
Shen YJ, Lee YL, Kobayashi J et al (2008) Assembly of CdS quantum dots onto mesoscopic TiO2 films for quantum dot-sensitized solar cell applications. Nanotechnology 19:045602
Loef R, Houtepen AJ, Talgorn E et al (2009) Study of electronic defects in CdSe quantum dots and their involvement in quantum dot solar cells. Nano Lett 9:856–859
Dayal S, Kopidakis N, Olson DC et al (2010) Photovoltaic devices with a low band gap polymer and CdSe nanostructures exceeding 3% efficiency. Nano Lett 10:239
Nozik AJ (2002) Quantum dot solar cells. Phys E 14:115–120
Trupke T, Green MA, Wurfel PJ (2002) Improving solar cell efficiencies by down-conversion of high-energy photons. J Appl Phys 92:1668–1674
Schaller RD, Klimov VI (2004) High efficiency carrier multiplication in PbSe nanocrystals: implications for solar energy conversion. Phys Rev Lett 92:186601
Klimov VI (2006) Mechanisms for photogeneration and recombination of multiexcitons in semiconductor nanocrystals: implications for lasing and solar energy conversion. J Phys Chem B 110:16827–16845
Schaller RD, Sykora M, Pietryga JM et al (2006) Seven excitons at a cost of one: redefining the limits for conversion efficiency of photons into charge carriers. Nano Lett 6:424–429
Ellingson RJ, Beard MC, Johnson JC et al (2005) Highly efficient multiple exciton generation in colloidal PbSe and PbS quantum dots. Nano Lett 5:865–871
McGuire JA, Sykora M, Joo J et al (2010) Apparent versus true carrier multiplication yields in semiconductor nanocrystals. Nano Lett 10:2049–2057
Beard MC, Midgett AG, Hanna MC et al (2010) Comparing multiple exciton generation in quantum dots to impact ionization in bulk semiconductors: implications for enhancement of solar energy conversion. Nano Lett 10:3019–3027
Midgett AG, Hillhouse HW, Hughes BK et al (2010) Flowing versus static conditions for measuring multiple exciton generation in PbSe quantum dots. J Phys Chem C 114:17486–17500
Witzel WM, Shabaev A, Hellberg CS et al (2010) Quantum simulation of multiple-exciton generation in a nanocrystal by a single photon. Phys Rev Lett 105:137401
Rabani E, Baer R (2010) Theory of multiexciton generation in semiconductor nanocrystals. Chem Phys Lett 496:227–235
Lin Z, Franceschetti A, Lusk MT (2011) Size dependence of the multiple exciton generation rate in CdSe quantum dots. ACS Nano 5:2503–2511
Timmerman D, Izeddin I, Stallinga P et al (2008) Space-separated quantum cutting with silicon nanocrystals for photovoltaic applications. Nat Photon 2:105–109
Brus LE (1984) Electron–electron and electron–hole interactions in small semiconductor crystallites: the size dependence of the lowest excited electronic state. J Chem Phys 80:4403–4409
Kayanuma Y (1988) Quantum-size effects of interacting electrons and holes in semiconductor microcrystals with spherical shape. Phys Rev B 38:9797–9805
Schaller RD, Agranovich VM, Klimov VI (2005) High-efficiency carrier multiplication through direct photogeneration of multi-excitons via virtual single-exciton states. Nat Phys 1:189–194
Griffths DJ (2004) Introduction to quantum mechanics, 2nd edn. Prentice Hall, London
Shockley W, Queisser HJ (1961) Detailed balance limit of efficiency of p–n junction solar cells. J Appl Phys 32:510–519
Shpaisman H, Niitsoo O, Lubomirsky I et al (2008) Can up- and down-conversion and multi-exciton generation improve photovoltaics? Sol Energy Mater Sol Cells 92:1541–1546
Klimov VI (2006) Detailed-balance power conversion limits of nanocrystal-quantum-dot solar cells in the presence of carrier multiplication. Appl Phys Lett 89:23118
Hanna MC, Nozik AJ (2006) Solar conversion efficiency of photovoltaic and photoelectrolysis cells with carrier multiplication absorbers. J Appl Phys 100:074510
Beard MC, Knutsen KP, Yu P et al (2007) Multiple exciton generation in colloidal silicon nanocrystals. Nano Lett 7:2506–2512
Klimov VI (2007) Spectral and dynamical properties of multiexcitons in semiconductor nanocrystals. Annu Rev Phys Chem 58:635–673
Trinh MT, Limpens R, De Boer WDAM et al (2012) Direct generation of multiple excitons in adjacent silicon nanocrystals revealed by induced absorption. Nat Photon 6:316–321
Nozik AJ, Beard MC, Luther JM et al (2010) Semiconductor quantum dots and quantum dot arrays and applications of multiple exciton generation to third-generation photovoltaic solar cells. Chem Rev 110:6873–6890
De Vos A, Desoete B (1998) On the ideal performance of solar cells with larger-than-unity quantum efficiency. Sol Energy Mater Sol Cells 51:413
Landsberg PT, Badescu V (2002) Solar cell thermodynamics including multiple impact ionization and concentration of radiation. J Phys D 35:1236
Acknowledgments
This study was supported by the National Natural Science Foundation of China (YG 61177056).
Author information
Authors and Affiliations
Corresponding author
About this article
Cite this article
Sun, L., Jiang, C. Semiconductor quantum dot-doped glass as spectral converter for photovoltaic application. Chin. Sci. Bull. 59, 16–22 (2014). https://doi.org/10.1007/s11434-013-0017-4
Received:
Accepted:
Published:
Issue Date:
DOI: https://doi.org/10.1007/s11434-013-0017-4