Abstract
Aims
The aim of the project was to determine the vertical variability of soil under the influence of deadwood (DWD) in a temperate forest ecosystem.
Methods
The laboratory analyses included soil layers of 0–5 cm, 5–10 cm, 10–20 cm and 20–40 cm, which were taken directly under the deadwood, as well as the forest litter layer and deadwood fragments. The control samples were taken 30 m away.
Results
The decomposition processes of deadwood are associated with a 55% increase in soil organic carbon (SOC) deposition to a depth of -40 cm and a 36% increase in total nitrogen (N) content compared to soils without deadwood. DWD significantly increases exchangeable cations, especially at a depth of -5 cm to -20 cm. Deadwood contains slightly more hydrogen (H+) and aluminum ions (Al3+) than forest litter, but soil acidification is related to pedogenic processes rather than decomposition of deadwood in hyperacid forest soils. The soil surface under deadwood with a high degree of decomposition is characterised by a lower bulk density (BD) value than the soil where only forest litter was present.
Conclusions
Our studies suggest that the physicochemical properties of forest soils change under the influence of deadwood such that the deeper layers beneath the logs take on propertie8s that make them similar to the shallower layers without deadwood. To summarise, leaving deadwood in the forest has a positive effect on soil fertility by enriching the soil with nutrients (Ca2+, K+, Na+, less Mg2+) and improving its physical properties.
Similar content being viewed by others
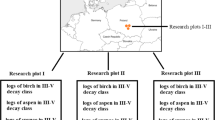
Avoid common mistakes on your manuscript.
Introduction
The moment a tree falls in the forest, the biochemical processes associated with its decomposition begin, which take place in the deadwood and in the soil. The decomposition of wood is a long-term process and takes between 10 and 100 years, depending on environmental conditions (Zielonka and Niklasson 2001), sometimes even over 300 years (Chen et al. 2005). The decomposition of deadwood is a key process in forest ecosystems that plays an important role in nutrient cycling (Cocciufa et al. 2014). Based on research and experiments, the factors causing coarse woody debris (CWD) decomposition have been described in detail. The decomposition of CWD has been shown to be important for habitat productivity, animal activity and tree seeding, and is a storehouse of carbon, water and nutrients (Harmon et al. 1986). Plant litters including dead leaves, stems, barks, flowers, fruits, and logs are the major sources of forest soil organic matter (SOM) (Osman 2013). The decomposition products of organic residues are absorbed into the litter and soil, they improve soil moisture in dry months and are resistant to destruction by fire (Page-Dumroese et al. 1991). It has been found that deadwood stores large amounts of water. Mosses play an important role in this process as they cover the dead stem and significantly increase its moisture content (Gutowski et al. 2004; Szukalska 2007). The rate of decomposition itself is the result of biotic factors and the locally created microclimate of the soil surface (Larsen et al. 1980; Swift and Boddy 1984; Breymeyer et al. 1998).
It is assumed that the most important abiotic factor influencing the rate of wood decomposition is moisture (Fravolini et al. 2016; Piaszczyk et al. 2022). Water determines the number of saprophytic fungi (Huang et al. 2022; Mayer et al. 2022) and is positively correlated with the number of decomposition taxa (Tarvainen et al. 2020; Hu et al. 2021). Among other abiotic factors related to the decomposition process of deadwood, its defragmentation by soil organisms is important. The fragmentation of the wood increases the availability of plant tissue for microorganisms and further biological decomposition is intensified (Harmon et al. 2000). Microorganisms are involved in the process of decomposition of deadwood and their activity determines the rate of decomposition. The most important biological role in wood decomposition is played by saprophytic fungi and bacteria, including cellulolytic and ligninolytic organisms, which determine the decomposition of lignin and cellulose by secreting enzymes (Wang et al. 2023). During the entire period of wood decomposition, white-rot fungi play the largest role, dominating the decomposition of hardwood and softwood (Arnstadt et al. 2016). Many factors have been found to influence the rate and intensity of development of the above groups, but the most important are pH and carbon and nitrogen content. Fungi grow much faster and more intensively than bacteria in soils with low pH values (Rousk and Bååth 2011). A high C/N ratio of the litter also favors fungi, as it increases the carbon content of the fungal hyphae colonizing the deadwood relative to the bacterial cells (Paustian and Schnurer 1987; Bakken 1985; Wallander et al. 2003). Organisms that decompose deadwood, such as fungi and bacteria, carry out wood decomposition processes and release organic carbon to the soil in the process (Bani et al. 2018; Piaszczyk et al. 2019). If little or no nitrogen is available, secondary metabolism occurs in conjunction with the degradation of lignin (Krajewski and Witomski 2005).
Some inferences may also come from research on nitrogen mineralization processes in litter. It has been found that even additional amounts of nitrogen (artificially or naturally introduced) do not cause significant and permanent changes in the chemical composition of litter, as they are likely to be leached out and mineralized very quickly (Frost and Hunter 2007; Sukovata et al. 2010). Long-term studies have shown that stem weight loss increases as soil nutrient content of the soil decreases. Fungi are responsible for this, which ensure the displacement of elements, especially between nutrient-poor soils and a rotting boot (Edelmann et al. 2023). Another important finding is that nutrient concentrations in decaying tree logs are sensitive to local microclimatic changes (Wang et al. 2022). Through leaching, the nutrients released during decomposition enter the soil with the rainwater. Three processes – defragmentation, decomposition and leaching – interact with each other and form a complex system for recycling CWD in forest ecosystems (Russell et al. 2015). The wood decomposition process shows considerable differences between tree species, especially between deciduous and coniferous species (Lasota et al. 2018).
The process of wood decomposition changes the physical, chemical and biochemical properties of forest soils (Błońska et al. 2017). During the decomposition process, 20 % of the carbon stored in deadwood is irretrievably lost in the form of CO2 released into the atmosphere, while 1/3 of the carbon stored in the wood is released into the soil (Lagomarsino et al. 2021). Piaszczyk et al. (2019) pointed out that the most decomposed deadwood significantly influences the composition of the SOM. Previous studies found significant differences in dissolved organic carbon (DOC) and nitrogen (N) concentrations in leachate from coniferous and deciduous trees. More DOC and N were released from decaying hornbeam and aspen wood, while the least DOC and TN were released into the soil from coniferous species, especially spruce (Błońska et al. 2019). SOC content, and thus the amount of organic matter, is a key factor influencing soil fertility in general (Zwydak et al. 2017; Ondrasek et al. 2019). However, current research on the effects of deadwood on soil does not explain the progression of physico-chemical transformations in soil from a vertical perspective. It is known that deadwood increases soil organic matter reserves in the upper soil layers (Wambsganss et al. 2017).
However, the extent to which deadwood alters the physicochemical properties of forest soils has not been determined. Previous research suggests that the effects of deadwood on soil decrease with depth (Nazari et al. 2023), but the limit of these effects has not been determined. The aim of our research was to determine the profound impact of deadwood on forest soil properties. We assume that the strongest impact of deadwood is in the superficial soil layers and has little impact at a depth of 20-40 cm. We assumed that deadwood leads to an increase in carbon accumulation in the near-surface layers of forest soils with a simultaneous increase in the content of basic cations.
Materials and methods
Study sites
The study plots were located in north-eastern Poland in the Masuria-Podlasie region, in the Białowieża Forest mesoregion (II.16) (Zielony and Kliczkowska 2012) (Table 1). The study area was formed during the Central Polish glaciation - the Warta Ice Age (170-120 thousand BP). Geologically, it is a poorly diversified, flat or slightly hilly plots covered with boulder clay, glacial fluvial sands and gravels, and outwash sands and gravels (Prusinkiewicz and Kowalkowski 1964; Fedorowicz et al. 1993). The study plots was dominated by podzols and brunic arenosols (IUSS working group WRB 2022). The analysed soils are characterised by a similar grain size, i.e. loamy sand. The climate of the Białowieża Forest is classified as a cool-temperate transitional climate. The average annual air temperature (1955-2001) was 6.8 °C. The average amount of precipitation is about 630 mm per year. The growing season is relatively short, lasting 205 days.
The investigation comprised 9 study plots. On each surface, a soil profile was dug directly at the location of the log. The most decomposed logs were included in the study (logs at the transition between the 4th and 5th degree of decomposition and logs in the 5th degree of decomposition). The degree of decomposition was determined according to the BioSoil methodology (2004): 4th degree - decomposed wood 26-75% of the wood was soft to very soft and 5th degree - very decomposed wood 76% - 100 % of the wood was soft. The sampling plan is shown in Figure 1. According to the BioSoil soil module methodology (Forest Focus Regulation (EC) N 2152/2003), mineral soil samples for laboratory analyzes were taken from the following layers: 0-5, 5-10, 10-20, 20-40 cm. The control soil samples were taken in the same way at 30 m distance from log. Deadwood samples (DWD) weighing approximately 0.5 kg were taken from the center of a decomposed log, and litter samples (O) were taken from control plots. Samples with intact structure were collected in 100 cm3 cylinders to determine bulk density. The deadwood surface variant is labeled with the DWD symbol, while the control surface variant is labeled with the no_DWD symbol.
Sampling scheme used in field surveys by BioSoil (2004): https://www.icp-forests.org/documentation/Surveys/BD/DWD.html
Soil physiochemical properties analysis
The laboratory tests included wood samples and soil samples from mineral layers up to a depth of -40 cm. A total of 90 samples were taken for laboratory analysis. In all samples were determined: bulk density by the gravimetric method, pH(CaCl2) (PN-ISO10390:1997) by SevenCompact Duo pH/Cond S213, Mettler-Toledo Company, total organic carbon [SOC] (PN-ISO10694:2002), total nitrogen content [N] (PN-ISO13878:2002) by Vario Max cube CN, Elementar Company, exchangeable acidity [Hex], exchangeable aluminum [Al3+], hydrogen ions [H+] (PB-12ed.3 01/07/2014) by Burette Dygital III 50ml, Brand Company, content of exchangeable cations (magnesium Mg2+, potassium K+, sodium Na+ and calcium Ca2+) (PB-05ed.3 01/07/2014) by ICP iCAP 7000 Series, Thermo Scientific Company. The content of total aluminum (Al), iron (Fe), magnesium (Mg), calcium (Ca), potassium (K), phosphorus (P), manganese (Mn) was determined using the extraction method according to the PN-ISO 11466:2002 standard and the inductively coupled plasma optical emission spectrometry (ICP-OES) method. Sum of exchangeable cations [EC] (Lambers and Barrow 2020) and C/N ratio was calculated on the basis of the results obtained.
Statistical analysis
The statistical analyzes were performed with the program R (R Core Team 2023). The significance of the differences in the analyzed variables between the DWD, O, 0-5, 5-10, 10-20, 20-40 cm layers were determined using the Friedman ANOVA and the Nemenyi post-hoc test from the "PMCMRplus" package (Pohlert 2022). The significance of the differences in the same layers between outcrops with and without deadwood was determined using the Wilcoxon test from the "stats" package (R Core Team 2023). The correlation between the analyzed variables in outcrops with and without deadwood was determined using Spearman's rank correlation and visualization of the results in the "corrplot" package (Wei and Simko 2021). The simultaneous effect of the presence of deadwood and soil layer on SOC, N and EC content was calculated using linear mixed models (LMM), which allow additional control of the influence of random effects. The "lmer" function from the "lme4" package was used (Bates et al. 2015). A normal distribution was achieved by logarithmic transformation of the underlying data. The formula used was: lmer(variable~DWD*shift+(1|profile ID)). The results were considered statistically significant at p<0.05. The diversity of soil layers was represented using NMDS analysis in the "vegan" package (Oksanen et al. 2022).
Results
The highest SOC and N contents were found in deadwood samples (Fig. 2). The SOC content in the analysed wood was 380 g C kg-1 ±28 and was higher than in the forest litter (300 g C kg-1 ±23), but significant differences were found at a depth of 0-5 cm, 5-10 cm and 10-20 cm below the wood (p<0.05). The highest N contents were found in the deadwood and in the organic horizon of the control plot. The nitrogen content was at a similar level in these layers. The SOC and N content decreased significantly with depth. A significantly higher SOC content in the upper layers leads to a broad C/N ratio throughout the profile, and at a depth of -10 cm these changes are significant (p< 0.05). Samples of deadwood and samples of organic horizons from control plots were characterized by the lowest bulk density. The BD of subsequent layers increased with depth, both in the plots with deadwood and in the control plots. The layer at a depth of 0-5 cm below the deadwood had a lower density than the corresponding layer in the control plot. A significantly lower density was found in the layers at a depth of 0-5 cm and 5-10 cm than in the deeper layers (p<0.05).
Vertical distribution of carbon and nitrogen content [g*kg-1],C/N ratio and BD in soils under the influence of deadwood [DWD] and without the influence of deadwood [no_DWD]. Different letters in the individual layers (a,b,c) indicate statistically significant differences based on Friedman's ANOVA and Nemenya's post-hoc test at p<0.05. Capital letters (A,B) - statistically significant differences in the same layers between DWD and no_DWD based on the Wilcoxon test (p<0.05)
Deadwood increases the carbon reserve up to a depth of -40 cm by 55 % compared to a soil that is not affected by deadwood. For nitrogen, a 36 % higher reserve was found in soils with deadwood compared to the control plots (Table 2). The pH values of the samples from the tested variants were found to be less variable with depth. Wood samples and the samples from the organic horizons had a higher pH value than the lower layers.
Up to a depth of -20 cm, the EC content under the influence of deadwood is about twice as high as in the samples without DWD, while the changes in soil acidity are not significant (Table 3). The total calcium content in the 0-5 cm thick layer is significantly higher in the soils under soil influence of deadwood than in the soils under the forest litter (Table 4). The other differences in cations content are statistically insignificant.
The analyzes confirmed the relationship between some of the properties of the studied soils (Fig. 3). A strong positive correlation was found between the carbon content and the total exchangeable bases. The bulk density was inversely related to the carbon and nitrogen content.
The NMDS analysis confirms significant changes in the properties of the soil layers under the influence of deadwood, in that the deeper layers under the log assume properties that make them similar to the higher layers in the variant without deadwood influence (Fig. 4).
The regression analysis confirmed that deadwood significantly influences all investigated layers and basic soil properties (Table 5). Both factors together, i.e. the presence of deadwood and the soil layer, have a significant effect on the nitrogen content and the sum of exchangeable cations. The variance of fixed and random effects (SD) for: SOC was 0.085 (0.291) and 0.099 (0.315); N: 0.09194 (0.303) and 0.064 (0.254); EC: 0.242 (0.492) and 0.119 (0.344)
Discussion
Our research shows that deadwood (DWD) significantly affects forest soil properties, especially soil organic carbon (SOC) resources, exchangeable cation (EC) content, soil acidity (Hex, Al3+) and bulk density (BD). The significant changes affect soil layers down to a depth of -20 cm.
Decaying soil organic matter (SOM) is important for habitat productivity (Grigal and Vance 2000; Thiffault et al. 2011), and the rate of its decomposition is the result of biotic factors and the locally created microclimate of the soil surface (Larsen et al. 1980; Swift and Boddy 1984; Edmonds 1991). Our research shows that this increase is 55% down to a depth of -40 cm. The explanation for this condition could be the high acidity of the analysed soils, which affects the carbon and nitrogen cycle. All soils in our studies were hyperacid (3.61< pH <4.41). High soil acidity can enhance the accumulation of organic materials in surface soils by decreasing the soil microbial activities for SOM decomposition (Funakawa et al. 2014). These researchers pointed out the downward movement of DOC under high acidity conditions, which increases the accumulation of SOM in the form of organo-mineral complexes. The inhibitory effect of soil acidification on decomposition processes and soil carbon dynamics was also confirmed by Růžek et al. (2021). With increasing soil acidification, the solubility of many of its components, especially organic carbon, increases (Christ and David 1996; Anderson et al. 2000; Remeš and Kulhavý 2009). Our study shows that the exchangeable acidity (Hex) in the 10-20 cm thick layer increased significantly due to DWD. This may indicate that soil acidification is related to pedogenic processes rather than wood decomposition. Some litter removal experiments, e.g. measurements within the DIRT (Detritus Input and Removal Treatments) project, have shown that soil pH decreases significantly in litter removal treatments compared to litter retention (Juhos et al. 2021). But in our studies, the presence of DWD was an additional natural factor. We found the greatest differences in pH of the soils studied in the O horizon, which is directly attributable to differences in the chemical composition of wood and litter (Kögel et al. 1988; Pettersen 1984). However, it should be noted that the pH differences in both the organic horizons and the mineral soil layers were not statistically significant. This is consistent with the results of studies on the decomposition properties of wood, where a constant decrease in pH from an initial 5.4 to 4.6 was observed in the final stage of decomposition (Kraigher et al. 2002).
Our studies also showed significant differences in the content of total N in soil layers down to a depth of -20 cm. Our research indicates an increase in the C/N ratio in the surface levels of the tested soils, which is the result of the impact of deadwood. However, using C/N as a general indicator of the nitrogen status of forests at the European scale, without explicit consideration of tree species, is too simplistic and may lead to misleading conclusions (Cools et al. 2014). Species composition influences the chemical, physical and biological properties of the soil in this influence is greatest in the topsoil (Augusto et al. 2002).
Our investigations also show that DWD, which is in an advanced stage of decomposition, contains slightly more H+ and Al3+ cations than forest litter, but the differences were not significant in contrast to the mineral layers. Soils in the pH range <4.5 generally have lower concentrations of adsorbed Al3+. This can be attributed to the lower availability of weather-resistant Al-containing minerals and the high proportion of weak organic acid (Rossa et al. 2008). The acidity of the soil and the Al3+ cations inhibit the activity of soil enzymes, which leads to an inhibition of nutrient circulation by microorganisms. Reduced SOM availability due to Al (and Fe) fixation can protect the SOC pool from microbial degradation in acidic forest soils (Kunito et al. 2016). It should be considered that fungi grow faster and more intensively in low pH soils compared to bacteria (Rousk and Bååth 2011). Fungi have been shown to alter their physical environment in acidic forest soils through the active translocation of metal cations from the mineral soil to the organic level (Clarholm and Skyllberg 2013). In our study, we found a significant difference in Al3+ content in the 10-20 cm thick layer, which may be related to increased Al3+ sorption by SOM. Our studies therefore confirm the observations of other researchers that deadwood is structurally and chemically different from litter and that its effects on the soil environment are different from those of leaves (Cotrufo et al. 2013). Previous work suggests a positive effect of litter on soil physicochemical properties, which may have implications for forest ecosystem resilience to climate change (Kuehne et al. 2008; Russell et al. 2015). Appropriate forestry practises, such as leaving deadwood in place, can help to maintain high carbon stocks in forest soils (Błońska et al. 2017; Piaszczyk et al. 2021). DWD is therefore a very important factor that slows down the carbon cycle in nature, especially in semi-natural forests. In the context of sustainable forest management, it is important to consider the role of deadwood in all forest ecosystems. In the face of climate change, maintaining the carbon balance in forest ecosystems is becoming crucial.
DWD is a source of nutrients released during decomposition processes (Stutz et al. 2017; Lasota et al. 2022; Piaszczyk et al. 2022). Jun-Hua et al. (2007) pointed out that soil moisture, organic matter content and exchangeable cations in the soil increase during forest succession and reach the highest value in the soil layer down to a depth of -15 cm (except for Na+). They also pointed out that the most important factor determining the content of exchangeable cations in the soil is SOM. Furthermore, it has been shown that the factor that correlates most strongly with SOM is exchangeable Ca2+ cations (Yao et al. 2022). Our research confirmed that DWD has a significant effect on the content and vertical distribution of exchangeable K+, Na+, Ca2+ and Mg2+ cations. The EC in forest litter and DWD was similar and was about 33 cmol(+)-kg-1. However, the processes accompanying the decomposition of wood and the accumulation of cations in mineral soil are different from those accompanying the decomposition of forest litter. Our studies indicate significant differences in EC in mineral soil, but their content was similar in litter and DWD. We observed significant changes down to a depth of -20 cm, and the largest EC differences between DWD and without DWD occurred in the 5-10 cm layer. At this point, it is important to address research findings that indicate that most exchangeable cations are located in deeper soil layers, below -1.00 m, and that the higher the SOC content in the soil, the lower the Ca2+ and Mg2+ content (James et al. 2016). Our results indicate the release of exchangeable cations as a result of the decomposition of DWD. The importance of these processes is low in the initial phase of decomposition, but as decomposition progresses, the leaching layer increases (Harmon et al. 1986). Studies by Fekete et al. (2020) show that higher Ca values were measured in drier oak forests with higher SOC content than in wetter forests with lower SOC content. Ca2+, Mg2+ and SOC also increased together in litter acidification experiments (Juhos et al. 2021; Tóth et al. 2011). Ca forms could therefore play an important role in SOC accumulation (Boiteau et al. 2020) and increase the stability of SOC compounds through several potential mechanisms (Rowley et al. 2018, 2021), however, these studies refer to alkaline soils. As previously mentioned, the soils we tested are hyperacid and prone to cation leaching and Al3+ activation. Therefore, the important role of soil SOM in buffering acids and retaining important cations in highly acidic forest soils is emphasised (Jiang et al. 2018). It should be noted that the quality of litter, which is influenced by species composition and ecosystem acidification, has little influence on the chemical composition of dissolved organic matter (DOM) (Ohno et al. 2007).
DWD also affects the physical properties of the soil and changes its structure. Our results showed that the soils under logs with heavily decomposed wood had a significant lower bulk density than the corresponding areas in control plots. The ability of the soil to store water (0-12 cm layer), which is correlated with the BD, depends on the decomposition rate of the organic residues (Ilek et al. 2017). Alexander (1980, 1988) and Huntington et al. (1989) indicated a strong correlation between SOM content and bulk density. This conclusion was confirmed in our study. In contrast to litter, DWD has a very specific surface area in relation to its volume (Stevens 1997). It therefore decomposes much more slowly than litter (Bujoczek 2012). Our research therefore indicates that leaving deadwood in the forest in the final phase of decomposition improves physical properties, decreasing BD. This in turn has a positive effect on the development of the new generation of the forest, especially on the growth parameters of seedlings of typical forest-forming species (Kormanek et al. 2015). The thickness of the litter layer can reduce the effects of extreme soil temperatures, which influences the microbial processing of litter and soil carbon (Fekete et al. 2016).
Conclusion
We show that DWD is a key component of biogeochemical cycles in forest ecosystems. DWD has a positive effect on the soil by increasing the accumulation of soil organic carbon and total nitrogen. The effect of strongly decaying wood is visible up to a depth of -20 cm under the log. Additionally, deadwood causes increases the content of exchangeable cations, especially in the upper layers of the soil. As a result of leaving the decomposing wood, changes in the physical properties of soils were noted, as evidenced by a reduction in bulk density. Our results indicate that leaving deadwood in forest ecosystems has a positive impact on soil condition, especially carbon sequestration.
Data availability
The datasets generated during the current study are available from the corresponding author (k.sokolowski@ibles.waw.pl) on reasonable request.
References
Alexander EB (1980) Bulk densities of California soils in relation to other soil properties. Soil Sci Soc Am J 44:689–692
Alexander EB (1988) Bulk density equations for southern Alaska soils. Can J Soil Sci 69:177–180
Anderson S, Nilsson SI, Saetre P (2000) Leaching of dissolved organic carbon (DOC) and dissolved organic nitrogen (DON) in mor humus as affected by temperature and pH. Soil Biol Biochem 32:1–10
Arnstadt T, Hoppe B, Kahl T, Kellner H, Krüger D, Bauhus J, Hofrichter M (2016) Dynamics of fungal community composition, decomposition and resulting deadwood properties in logs of Fagus sylvatica, Picea abies and Pinus sylvestris. Forest Ecol Manag 382:129–142
Augusto L, Ranger J, Binkley D, Rothe A (2002) Impact of several common tree species of European temperate forests on soil fertility. Ann For Sci 59(3):233–253
Bakken LR (1985) Separation and Purification of Bacteria from Soil. Appl Environ Microbiol 49(6):1482–1487
Bani A, Pioli S, Ventura M, Panzacchi P, Borruso L, Tognetti R, Tonon G, Brusetti L (2018) The role of microbial community in the decomposition of leaf litter and deadwood. Appl Soil Ecol 126:75–84
Bates D, Maechler M, Bolker B, Walker S (2015) Fitting Linear Mixed-Effects Models Using lme4. J Statis Softw 67(1):1–48. https://doi.org/10.18637/jss.v067.i01
Boiteau R, Kukkadapu R, Cliff J, Smallwood C (2020) Calcareous organic matter coatings sequester siderophores in alkaline soils. Sci Total Environ 724:138250
Błońska E, Kacprzyk M, Spólnik A (2017) Effect of deadwood of different tree species in various stages of decomposition on biochemical soil properties and carbon storage. Ecol Res 32:193–203
Błońska E, Lasota J, Piaszczyk W (2019) Dissolved carbon and nitrogen release from deadwood of different tree species in various stages of decomposition. Soil Sci Plant Nutr 65(1):100–107
Breymeyer A, Mroz G, Reed D, Degórski M (1998) Warunkowanie tempa dekompozycji ściółki sosnowej przez zmieniający się klimat i skład chemiczny substratu. Badania na transektach (w:) Breymeyer A., Roo-Zielińska E. (red.), Bory sosnowe w gradiencie kontynentalizmu i zanieczyszczeń w Europie Środkowej - badania geoekologiczne. Dokumentacja Geograficzna 13:187–205
Bujoczek L (2012) Dekompozycja obumarłych drzew w ekosystemach leśnych ze szczególnym uwzględnieniem świerka, buka i jodły. Sylwan 156(3):208–217
Chen X, Wei X, Scherer R (2005) Influence of wildfire and harvest on biomass, carbon pool, and decomposition of large woody debris in forested streams of southern interior British Columbia. For Ecol Manag 208:101–114
Christ MJ, David MB (1996) Dynamics of extractable organic carbon in spodosol forest floors. Soil Biol Biochem 28(9):1171–1179
Clarholm M, Skyllberg U (2013) Translocation of metals by trees and fungi regulates pH, soil organic matter turnover and nitrogen availability in acidic forest soil. Soil Biol Biochem 63:142–153
Cocciufa C, Gerth W, Luiselli L, Redolfi de Zan L, Cerretti P, Carpaneto GM (2014) Survey of saproxylic beetle assemblages at different forest plots in central Italy. Bull Insectol 67:295–306
Cools N, Vesterdal L, De Vos B, Vanguelova E, Hansen K (2014) Tree species is the major factor explaining C:N ratios in European forest soil. For Ecol Manag 311:3–16. https://doi.org/10.1016/j.foreco.2013.06.047
Cotrufo MF, Wallenstein MD, Boot CM, Denef K, Paul E (2013) The Microbial Efficiency-Matrix Stabilization (MEMS) framework integrates plant litter decomposition with soil organic matter stabilization: do labile plant inputs form stable soil organic matter? Glob Chang Biol 19:988–995
Edelmann P, Weisser WW, Ambarlı D, Bässler C, Buscot F, Hofrichter M, Hoppe B, Kellner H, Minnich C, Moll J, Persoh D, Seibold S, Seilwinder C, Schulze E-D, Wöllauer S, Borken W (2023) Regional variation in deadwood decay of 13 tree species: Effects of climate, soil and forest structure. For Ecol Manag 541:121094
Edmonds RL (1991) Organic matter decomposition in western United States forests (w:) Management and productivity of western montane forest soils. USDA Forest Service Gen Tech Rep INT-280:118–128
Fedorowicz S, Grzybowski K, Marks L (1993) Zlodowacenie Nadwarciańskie w rejonie Warszawy na podstawie najnowszych datowań termoluminescencyjnych. Geol Q 37:67–80 https://gq.pgi.gov.pl/article/view/8443/pdf_519
Fekete I, Varga C, Biró B, Tóth JA, Várbíró G, Lajtha K, Szabó G, Kotroczó Z (2016) The effects of litter production and litter depth on soil microclimate in a central european deciduous forest. Plant Soil 398:291–300
Fekete I, Berki I, Lajtha K, Trumbore S, Francioso O, Gioacchini P, Montecchio D, Várbíró G, Béni A, Makádi M, Demeter I, Madarász B, Juhos K, Kotroczó Z (2020) How will a drier climate change carbon sequestration in soils of the deciduous forests of Central Europe? Biogeochemistry 152:13–32
Fravolini G, Egli M, Derungs C, Cherubini P, Ascher-Jenull J, Gomez-Barandon M, Bardelli T, Tognetti R, Lombardi F, Marchetti M (2016) Soil attributes and microclimate are important drivers of initial deadwood decay in sub-alpine Norway spruce forests. Sci Total Environ 569-570:1064–1076
Frost CJ, Hunter MD (2007) Recycling of nitrogen in herbivore feces: plant recovery, herbivory assimilation, soil retention, and leaching losses. Oecologia. 151(1):42–53
Funakawa S, Fujii K, Kadono A, Watanabe T, Kosaki T (2014) Could Soil Acidity Enhance Sequestration of Organic Carbon in Soils? In: Soil Carbon. Springer, pp 209–216
Grigal DF, Vance ED (2000) Influence of soil organic matter on forest productivity. N Z J For Sci 30(1/2):169–205
Gutowski JM, Bobiec A, Pawlaczyk P, Zub K (2004) Drugie życie drzewa. WWF Polska
Harmon ME, Franklin JF, Swanson FJ, Sollins P, Gregory SV, Lattin JD, Anderson NH, Cline SP, Aumen NG, Sedell JR, Lienkaemper GW, Cromack K, Cummins KW (1986) Ecology of coarse woody debris in temperate ecosystems. Adv Ecol Res 15:133–302
Harmon ME, Krankina ON, Sexton J (2000) Decomposition vectors: a new approach to estimating wood detritus decomposition dynamics. Can J For Res 30:76–84
Hu Y, Yesilonis I, Szlavecz K (2021) Microbial and environmental controls on wood decomposition in deciduous forests of different ages. Appl Soil Ecol 166:103986
Huang C, Wu X, Liu X, Fang Y, Liu L, Wu C (2022) Functional fungal communities dominate wood decomposition and are modified by wood traits in a subtropical forest. Sci Total Environ 806:151377
Huntington TG, Johnson CE, Johnson AH, Siccama TG, Ryan DF (1989) Carbon, organic matter, and bulk density relationships in a forested spodosol. Soil Sci 148:380–386
Ilek A, Kucza J, Szostek M (2017) The effect of the bulk density and the decomposition index of organic matter on the water storage capacity of the surface layers of forest soils. Geoderma 285:27–34
IUSS Working Group WRB (2022) World Reference Base for Soil Resources. International soil classification system for naming soils and creating legends for soil maps, 4th edn. International Union of Soil Sciences (IUSS), Vienna, Austria
James J, Littke K, Bonassi T, Harrison R (2016) Exchangeable cations in deep forest soils: Separating climate and chemical controls on spatial and vertical distribution and cycling. Geoderma 279:109–121
Jiang J, Wang Y-P, Yu M, Cao N, Yan J (2018) Soil organic matter is important for acid buffering and reducing aluminum leaching from acidic forest soils. Chem Geol 501:86–94
Juhos K, Madarász B, Kotroczó Z, Béni Á, Makádi M, Fekete I (2021) Carbon sequestration of forest soils is reflected by changes in physicochemical soil indicators - A comprehensive discussion of a long-term experiment on a detritus manipulation. Geoderma 385:114918
Jun-Hua Y, Guo-Yi Z, De-Qiang Z, Guo-Wei C (2007) Changes of Soil Water, Organic Matter, and Exchangeable Cations Along a Forest Successional Gradient in Southern China. Pedosphere 17:397–405
Kormanek M, Banach J, Sowa P (2015) Effect of soil bulk density on forest tree seedlings. Int Agrophys 29:67–74
Kögel I, Hempfling R, Reinhold W, Hatcher PG, Schulten H-R (1988) Chemical composition of the organic matter in forest soils. Soil Sci 146:124–136
Krajewski A, Witomski P (2005) Ochrona drewna – surowca i materiału. SGGW, Warszawa
Kraigher H, Jurc D, Kalan P, Kutnar L, Levanič T, Rupel M, Smolej I (2002) Beech coarse woody debris characteristics in two virgin forest reserves in southern Slovenia. Zbornik gozdarstva in lesarstva 69:91–134
Kuehne C, Donath C, Műller-Using SI, Bartsch N (2008) Nutrient fluxes via leaching from coarse woody debris in a Fagus sylvatica forest in the Solling Mountains, Germany Canadian. J Forest Res 38:2405–2413
Kunito T, Isomura I, Sumi H, Park H-D, Toda H, Otsuka S, Nagaoka K, Saeki K, Senoo K (2016) Aluminum and acidity suppress microbial activity and biomass in acidic forest soils. Soil Biol Biochem 97:23–30
Lagomarsino A, De Meo I, Agnelli AE, Paletto A, Mazza G, Bianchetto E, Pastorelli R (2021) Decomposition of black pine (Pinus nigra J. F. Arnold) deadwood and its impact on forest soil components. Sci Total Environ 754:142039. https://doi.org/10.1016/j.scitotenv.2020.142039
Lambers H, Barrow NJ (2020) P2O5, K2O, CaO, MgO, and basic cations: pervasive use of references to molecules that do not exist in soil. Plant Soil 452:1–4
Larsen MJ, Harvey AE, Jurgensen MF (1980) Residue decay processes and associated environmental functions in northern Rocky Mountain Forests (w:) Environmental consequence of timber harvesting in Rocky Mountain Coniferous Forests. In: USDA Forest Service, Forest Products Laboratory INT-90. USDA Forest Service, Forest Products Laboratory, pp 157–194
Lasota J, Błońska E, Piaszczyk W, Wiecheć M (2018) How the deadwood of different tree species in various stages of decomposition affected nutrient dynamics? J Soils Sediments 18:2759–2769
Lasota J, Piaszczyk W, Błońska E (2022) Fine woody debris as a biogen reservoir in forest ecosystems. Acta Oecol 115:103822
Mayer M, Rosinger C, Gorfer M, Berger H, Deltedesco E, Bässler C, Müller J, Seifert L, Rewald B, Godbold DL (2022) Surviving trees and deadwood moderate changes in soil fungal communities and associated functioning after natural forest disturbance and salvage logging. Soil Biol Biochem 166:108558
Nazari M, Pausch J, Bickel S, Bilyera N, Rashtbari M, Razavi BS, Zamanian K, Sharififar A, Shi L, Dippold MA, Zarebanadkouki M (2023) Keeping thinning-derived deadwood logs on forest floor improves soil organic carbon, microbial biomass, and enzyme activity in a temperate spruce forest. Eur J For Res 142:287–300
Ohno T, Fernandez I, Hiradate S, Sherman J (2007) Effects of soil acidification and forest type on water soluble soil organic matter properties. Geoderma 140:176–187
Oksanen J, Simpson G, Blanchet F, Kindt R, Legendre P, Minchin P, O'Hara R, Solymos P, Stevens M, Szoecs E, Wagner H, Barbour M, Bedward M, Bolker B, Borcard D, Carvalho G, Chirico M, De Caceres M, Durand S, Evangelista H, FitzJohn R, Friendly M, Furneaux B, Hannigan G, Hill M, Lahti L, McGlinn D, Ouellette M, Ribeiro Cunha E, Smith T, Stier A, Ter Braak C, Weedon J (2022). vegan: Community Ecology Package. R package version 2.6-4, https://CRAN.R-project.org/package=vegan.
Ondrasek G, Bakić BH, Zovko M, Filipović L, Meriño-Gergichevich C, Savić R, Rengel Z (2019) Biogeochemistry of soil organic matter in agroecosystems & environmental implications. Sci Total Environ 658:1559–1573
Osman KT (2013) Organic Matter of Forest Soils. Forest Soil Book:63–76. https://doi.org/10.1007/978-3-319-02541-4_4
Page-Dumroese DS, Harvey AE, Jurgensen MF, Graham RT (1991) Organic matter function in the inland Northwest forest soil system (w:) Management and productivity of western montane forest soils. In: USDA Forest Service Gen Tech Rep INT- 280, pp 95–100
Paustian K, Schnurer J (1987) Fungal growth response to carbon and nitrogen limitation: Application of a model to laboratory and field data. Soil Biol Biochem 19:621–629
Pettersen RC (1984) The Chemical Composition of Wood. U.S. Department of Agriculture, Forest Service, Forest Products Laboratory, Madison, WI 53705
Piaszczyk W, Błońska E, Lasota J (2019) Soil biochemical properties and stabilization soil organic matter in relation to deadwood of different species. FEMS Microbiol Ecol 95(3). https://doi.org/10.1093/femsec/fiz011
Piaszczyk W, Lasota J, Gaura G, Błońska E (2021) Effect of deadwood decomposition on the restoration of soil cover in landslide areas of the Karpaty Mountains. Poland Forests 12:237
Piaszczyk W, Lasota J, Błońska E, Foremnik K (2022) How habitat moisture condition affects the decomposition of fine woody debris from different species. Catena. 208:105765
Pohlert T. 2022. PMCMRplus: Calculate Pairwise Multiple Comparisons of Mean Rank Sums Extended. R package version 1.9.6, https://CRAN.R-project.org/package=PMCMRplus.
R Core Team (2023) R: A Language and Environment for Statistical Computing. R Foundation for Statistical Computing, Vienna, Austria https://www.R-project.org
Prusinkiewicz Z, Kowalkowski A (1964) Studia gleboznawcze w Białowieskim Parku Narodowym. Soil Sci Annu 14:161–305 http://ssa.ptg.sggw.pl/artykul/482/pedological-studies-in-the-bialowieza-national-park
Remeš M, Kulhavý J (2009) Dissolved organic carbon concentrations under conditions of different forest composition. J For Sci 55:201–207
Rossa DS, Matschonat AG, Skyllberga BU (2008) Cation exchange in forest soils: the need for a new perspective. Eur J Soil Sci 59:1141–1159
Rousk J, Bååth E (2011) Growth of saprotrophic fungi and bacteria in soil. FEMS Microbiol Ecol 78(1):17–30
Rowley MC, Grand S, Verrecchia ÉP (2018) Calcium-mediated stabilisation of soil organic carbon. Biogeochemistry 137:27–49
Rowley MC, Grand S, Spangenber JE, Verrecchia EP (2021) Evidence linking calcium to increased organo-mineral association in soils. Biogeochemistry 153:223–241
Russell MB, Fraver S, Aakala T, Gove JH, Woodall CW, D’Amato AW, Ducey MJ (2015) Quantifying carbon stores and decomposition in dead wood: A review. For Ecol Manag 350:107–128
Růžek M, Tahovská K, Guggenberger G, Oulehle F (2021) Litter decomposition in European coniferous and broadleaf forests under experimentally elevated acidity and nitrogen addition. Plant Soil 463:471–485
Stevens V (1997) The Ecological Role of Coarse Woody Debris: an Overview of the Ecological Importance of CWD in BC Forests. In: Research Program, British Columbia. Ministry of Forests, Victoria, B.C.
Stutz KP, Dann D, Wambsganss J, Scherer-Lorenzen M, Lang F (2017) Phenolic matter from deadwood can impact forest soil properties. Geoderma 288:204–212
Sukovata L, Kolk A, Karolewski P, Smolewska M, Isidorov V (2010) Wpływ zerowania owadów liściożernych sosny na skład chemiczny igliwia, ściółki i gleby. Sylwan 154(9):639–648
Swift MJ, Boddy L (1984) Animal-microbial interactions in wood decomposition (w). In: Andersen JM, Raynerd ADM, Walton DWH (eds) Invertebrate-microbe Interactions. Cambridge University Press, Cambridge, pp 89–131
Szukalska D (2007) Plant communities on dead trees on forest of northern slopes of Babia Góra (Beskidy Mts.). Bogucki Wydawnictwo Naukowe, Poznań
Tarvainen O, Saravesi K, Pennanen T, Markkola AM, Suokas M, Fritze H (2020) Fungal communities in decomposing wood along an energy wood harvest gradient. Forest Ecol Manag 465:118070
Thiffault E, Hannam KD, Paré D, Titus BD, Hazlett PW, Maynard DG, Brais S (2011) Effects of forest biomass harvesting on soil productivity in boreal and temperate forests — A review. Environ Rev 19:278–309. https://doi.org/10.1139/a11-009
Tóth JA, Nagy PT, Krakomperger Z, Veres Z, Kotroczó Z, Kincses S, Fekete I, Papp M, Lajtha K (2011) Effect of litter fall on soil nutrient content and pH, and its consequences in view of climate change (Síkfőkút DIRT Project). Acta Silvatica et Lignaria Hungarica 7:75–86
Wallander H, Mahmood S, Hagerberg D, Johansson L, Pallon J (2003) Elemental composition of ectomycorrhizal mycelia identified by PCR-RFLP analysis and grown in contact with apatite or wood ash in forest soil. FEMS Microbiol Ecol 44(1):57–65
Wambsganss J, Stutz KP, Lang F (2017) European beech deadwood can increase soil organic carbon sequestration in forest topsoils. For Ecol Manag 405:200–209
Wang D, Abiodun OO, Xiao J, Zhao W (2023) Contrasting responses of microbial diversity and community structure in decaying root bark and xylem to N addition in an alpine shrubland. Soil Biol Biochem 178:108937
Wang Z, Yang W, Tan B, Wang Q, Wang L, Chang C, Cao R, Jiang Y, Muller J (2022) Effects of forest canopy density and epixylic vegetation on nutrient concentrations in decaying logs of a subalpine fir forest. Forest Ecosyst 9:100064
Wei T, Simko V 2021. R package 'corrplot': Visualization of a Correlation Matrix (Version 0.92), https://github.com/taiyun/corrplot.
Yao Y, Chen J, Li F, Sun M, Yang X, Wang G, Ma J, Sun W (2022) Exchangeable Ca2+ content and soil aggregate stability control the soil organic carbon content in degraded Horqin grassland. Ecol Indic 134:108507
Zielonka T, Niklasson M (2001) Dynamics of Dead Wood and Regeneration Pattern in Natural Spruce Forest in the Tatra Mountains, Poland. Ecol Bull 49:159–163
Zielony R, Kliczkowska M (2012) Regionalizacja przyrodniczo-geograficzna Polski 2010. Centrum Informacyjne Lasów Państwowych, Warszawa
Zwydak M, Błońska E, Lasota J (2017) Akumulacja węgla organicznego w glebach różnych typów siedlisk leśnych. Sylwan 161:62–70
Funding
The research was funded by the Ministry of Education and Science as part of the statutory activities of the Forest Research Institute (PL) under research topic no. 900801.
Author information
Authors and Affiliations
Contributions
The field work and soil samples were carried out by Karol Sokołowski. The laboratory analyses were carried out by Karol Sokołowski and the Natural Environmental Chemistry Laboratory, Forest Research Institute (PL). The first draft of the manuscript was written by Karol Sokołowski and Ewa Błońska. Statistical analyses were calculated by Radosław Gawryś. All authors edited earlier versions of the manuscript. All authors have read and approved the final version of the manuscript.
Corresponding author
Ethics declarations
Competing interest
All authors certify that they have no affiliations with or involvement in any organization or entity with any financial interest or non-financial interest in the subject matter or materials discussed in this manuscript.
Additional information
Responsible Editor: Ruzhen Wang.
Publisher’s note
Springer Nature remains neutral with regard to jurisdictional claims in published maps and institutional affiliations.
Rights and permissions
Open Access This article is licensed under a Creative Commons Attribution 4.0 International License, which permits use, sharing, adaptation, distribution and reproduction in any medium or format, as long as you give appropriate credit to the original author(s) and the source, provide a link to the Creative Commons licence, and indicate if changes were made. The images or other third party material in this article are included in the article's Creative Commons licence, unless indicated otherwise in a credit line to the material. If material is not included in the article's Creative Commons licence and your intended use is not permitted by statutory regulation or exceeds the permitted use, you will need to obtain permission directly from the copyright holder. To view a copy of this licence, visit http://creativecommons.org/licenses/by/4.0/.
About this article
Cite this article
Sokołowski, K., Gawryś, R. & Błońska, E. The falling of a tree in the forest is the beginning of significant changes in the soil. Plant Soil (2024). https://doi.org/10.1007/s11104-024-06737-0
Received:
Accepted:
Published:
DOI: https://doi.org/10.1007/s11104-024-06737-0