Abstract
A macro-continuum model of the traffic flow is derived from a micro-car-following model that considers both the upslope and downslope by using the transformation relationship between macro- and micro-variables. The perturbation propagation characteristics and stability conditions of the macroscopic continuum equation are discussed. For uniform flow in the initial equilibrium state, the stability conditions reveal that as the slope angle increased under the action of a small disturbance, the upslope stability increases and downslope stability decreases. Moreover, under a large disturbance, the global stability analysis is carried out by using the wavefront expansion technique for uniform flow in the initial equilibrium state. For the initial nonuniform flow, nonlinear bifurcation analysis such as Hopf bifurcation and saddle–node bifurcation is carried out at the equilibrium point. Subcritical Hopf bifurcation exists when the traffic flow state changes; thus, the limit cycle formed by the Hopf bifurcation is unstable. And the existence condition of saddle-node bifurcation is obtained. Simulation results verify the stability conditions of the model and determine the critical density range. The numerical simulation results show the existence of Hopf bifurcation in phase space, and the spiral saddle point of saddle-node bifurcation varies with the slope angle. Furthermore, the impact of the angle of both the upslope and downslope on the evolution of density waves is investigated.










Similar content being viewed by others
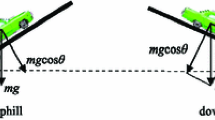
Data availability
The datasets/codes are available from the corresponding author on reasonable request.
References
Payne, H.J.: FREFLO: A macroscopic simulation model of freeway traffic. Transp. Res. Rec. 722, 68–77 (1979)
Nagatani, T.: The physics of traffic jams. Rep. Prog. Phys. 65, 1331–1386 (2002)
Zhang, H.M.: A theory of non-equilibrium traffic flow. Transp. Res. Part B 32, 485–498 (1998)
Nagel, K., Schreckenberg, M.: A cellular automaton model for freeway traffic. J. Phys. I(2), 2221–2229 (1992)
Mcdowell, M.: Kinetic theory of vehicular traffic. J. Oper. Res. Soc. 23(4), 599–600 (2017)
Pipes, L.A.: An operational analysis of traffic dynamics. J. Appl. Phys. 24, 274–281 (1953)
Newell, G.F.: Nonlinear effects in the dynamics of car following. Oper. Res. 9, 209–229 (1961)
Bando, M., Hasebe, K., Nakayama, A., et al.: Dynamical model of traffic congestion and numerical simulation. Phys. Rev. E 51, 1035 (1995)
Lighthill, M.J., Rs, F., Whitham, G.B.: On kinematic waves I. Flood movement in long rivers. Math. Phys. Sci. 229, 281–316 (1955)
Richards, P.I.: Shock waves on the highway. Oper. Res. 4, 42–51 (1956)
Payne, H.J.: Models of freeway traffic and control. Math. Models Public Syst. Simul. Council. 1, 51–61 (1971)
Papageorgiou, M.: A hierarchical control system for freeway traffic. Transp. Res. Part B Methodol. 17(3), 251–261 (1983)
Kühne, R.D.: Macroscopic freeway model for dense traffic: stop-start waves and incident detection. Int. Symp. Transp. Traffic Theory 9, 21–42 (1984)
Kerner, B.S., Konhäuser, P.: Structure and parameters of clusters in traffic flow. Phys. Rev. E 50(1), 54–83 (1994)
Lee, H.Y., Lee, H.W., Kim, D.: Dynamic states of a continuum traffic equation with on-ramp. Phys. Rev. E 59(5), 5101–5111 (1999)
Daganzo, C.F.: Requiem for second-order fluid approximations of traffic flow. Transp. Res. Part B: Methodol. 29(4), 277–286 (1995)
Kaur, R., Sharma, S.: Analysis of driver’s characteristics on a curved road in a lattice model. Phys. A 471, 59–67 (2017)
Redhu, P., Gupta, A.K.: Effect of forward looking sites on a multi-phase lattice hydrodynamic model. Phys. A 445, 150–160 (2016)
Sharma, S.: Lattice hydrodynamic modeling of two-lane traffic flow with timid and aggressive driving behavior. Phys. A 421, 401–411 (2015)
Redhu, P., Gupta, A.K.: Delayed-feedback control in a lattice hydrodynamic model. Commun. Nonlinear Sci. Numer. Simulat. 27, 263–270 (2015)
Redhu, P., Gupta, A.K.: Jamming transitions and the effect of interruption probability in a lattice traffic flow model with passing. Phys. A 421, 249–260 (2015)
Gupta, A.K., Sharma, S., Redhu, P.: Effect of multi-phase optimal velocity function on jamming transition in a lattice hydrodynamic model with passing. Nonlinear Dyn. 80, 1091–1108 (2015)
Gupta, A.K., Redhu, P.: Analyses of the driver’s anticipation effect in a new lattice hydrodynamic traffic flow model with passing. Nonlinear Dyn. 76(2), 1001–1011 (2014)
Gupta, A.K., Redhu, P.: Analyses of driver’s anticipation effect in sensing relative flux in a new lattice model for two-lane traffic system. Phys. A 392, 5622–5632 (2013)
Kuang, H., et al.: An extended car-following model accounting for the honk effect and numerical tests. Nonlinear Dyn. 87, 149–157 (2017)
Qiang, X.H., Huang, L.: Traffic flow modeling in fog with cellular automata model. Modern Phys. Lett. B. 35(11), 2150180(1)-2150180(14) (2021)
Xue, Y., Zhang, Y.C., et al.: An extended macroscopic model for traffic flow on curved road and its numerical simulation. Nonlinear Dyn. 95(4), 3295–3307 (2019)
Kerner, B.S., Rehborn, H.: Experimental properties of phase transitions in traffic flow. Phys. Rev. Lett. 79, 4030–4033 (1997)
Helbing, D., Treiber, M.: Gas-kinetic-based traffic model explaining observed hysteretic phase transition. Phys. Rev. Lett. 81, 3042–3045 (1998)
Lee, H.Y., Lee, H.W., Kim, D.: Traffic states of a model highway with on-ramp. Phys. A 281, 78–86 (2008)
Komada, K., Masukura, S., Nagatani, T.: Effect of gravitational force upon traffic flow with gradients. Phys. A 388, 2880–2894 (2009)
Zhu, W.X., Yu, R.L.: Nonlinear analysis of traffic flow on a gradient highway. Phys. A 391, 954–965 (2012)
Wu, C.X., Zhang, P., Wong, S.C., Choi, K.: Steady-state traffic flow on a ring road with up- and down-slopes. Phys. A 403, 85–93 (2014)
Gupta, A.K., Sharma, S., Redhu, P.: Analyses of lattice traffic flow model on a gradient highway. Commun. Theor. Phys. 62(3), 393–404 (2014)
Kaur, R., Sharma, S.: Modeling and simulation of driver’s anticipation effect in a two lane system on curved road with slope. Phys. A 499, 110–120 (2018)
Li, X.L., Song, T., Kuang, H., Dai, S.Q., et al.: Phase transition on speed limit traffic with slope. Chin. Phys. B 17(8), 3014–3020 (2008)
Yu, R.L., Zhang, C.H.: Slope effect in traffic flow with a speed difference. Appl. Mech. Mater. 361–363, 2297–2303 (2013)
Chen, J.Z., Peng, Z.Y., et al.: An extended lattice model for two-lane traffic flow with consideration of the slope effect. Mod. Phys. Lett. B 29(05), 1550017–1550022 (2015)
Tan, J.H., Gong, L., Qin, X.Q.: An extended car-following model considering the low visibility in fog on a highway with slopes. Int. J. Mod. Phys. C 30(11), 1950090–1950096 (2019)
Zhou, J., Shi, Z.K., Cao, J.L.: An extended traffic flow model on a gradient highway with the consideration of the relative velocity. Nonlinear Dyn. 78(3), 1765–1779 (2014)
Zhang, X.D., Xu, J.L., Liang, Q.Q., et al.: Modeling impacts of speed reduction on traffic efficiency on expressway up slope sections. Sustainability 12(2), 587 (2020)
Choi, S., Suh, J., Yeo, H.: Microscopic analysis of climbing lane performance at freeway up slope section. Transp. Res. Proc. 21, 98–109 (2017)
He, H.D., Lu, W.Z., Xue, Y.: Dynamic characteristics and simulation of traffic flow with slope. Chin. Phys. B 18(7), 2703–2708 (2009)
Wang, Q.Y., Cheng, R.J., Ge, H.X.: A new lattice hydrodynamic model accounting for the traffic interruption probability on a gradient highway. Phys. Lett. A 16, 1879–1887 (2019)
Kuang, H., et al.: An extended car-following model incorporating the effects of driver’s memory and mean expected velocity field in ITS environment. Int. J. Mod. Phys. C 32, 2150095 (2021)
Kuang, H., et al.: An extended car-following model considering multi-anticipative average velocity effect under V2V environment. Phys. A 527, 121268 (2019)
Kuang, H., et al.: An extended car-following model accounting for the average headway effect in intelligent transportation system. Phys. A 471, 778–787 (2017)
Berg, P., Mason, A., Woods, A.: Continuum approach to car-following models. Phys. Rev. E 61, 1056–1066 (2000)
Helbing, D.: Derivation of non-local macroscopic traffic equations and consistent traffic pressures from microscopic car-following models. Eur. Phys. J. B 69(4), 539–548 (2009)
Gupta, A.K., Katiyar, V.K.: A new anisotropic continuum model for traffic flow. Phys. A 368(2), 551–559 (2006)
Gupta, A.K., Katiyar, V.K.: Analyses of shock waves and jams in traffic flow. J. Phys. A: Math. Gen. 38, 4069–4083 (2005)
Yi, J.G., Lin, H., Alvarez, L., Horowitz, R.: Stability of macroscopic traffic flow modeling through wavefront expansion. Transp. Res. Part B 37, 661–679 (2003)
Ou, Z.H., Dai, S.Q., Zhang, P., Dong, L.Y.: Nonlinear analysis in the Aw-Rascle anticipation model of traffic flow. SIAM J. Appl. Math. 67(3), 605–618 (2007)
Gupta, A.K., Sharma, S.: Nonlinear analysis of traffic jams in an anisotropic continuum model. Chin. Phys. B 11, 160–168 (2010)
Carrillo, F.A., Delgado, J., et al.: Traveling waves, catastrophes and bifurcations in a generic second order traffic flow model. Int. J. Bifurcat. Chaos. 23, 1350191–1350207 (2013)
Delgado, J., Saavedra, P.: Global bifurcation diagram for the Kerner-Konhauser traffic flow model. Int. J. Bifurcat. Chaos. 25, 1550064–1550075 (2015)
Gasser, I., Sirito, G., Werner, B.: Bifurcation analysis of a class of ‘car following’ traffic models. Phys. D 197, 222–241 (2004)
Orosz, G.: Hopf bifurcation calculations in delayed systems. Period. Polytech. 48, 189–200 (2004)
Orosz, G., Stepan, G.: Hopf bifurcation calculations in delayed systems with translational symmetry. J. Nonlin. Sci. 14, 505–528 (2004)
Orosz, G., Stepan, G.: Subcritical Hopf bifurcations in a car-following model with reaction-time delay. Proc. R. Soc. A 462, 2643–2670 (2006)
Ngoduy, D., Li, T.: Hopf bifurcation structure of a generic car-following model with multiple time delays. Transp. A: Transp. Sci. 17, 878–896 (2020)
Ai, W.H., Shi, Z.K., Liu, D.W.: Bifurcation analysis of a speed gradient continuum traffic flow model. Phys. A 437, 418–429 (2015)
Miura, Y., Sugiyama, Y.: Coarse analysis of collective behaviors: Bifurcation analysis of the optimal velocity model for traffic jam formation. Phys. Lett. A 381, 3983–3988 (2017)
Ren, W.L., Cheng, R.J., Ge, H.X.: Bifurcation analysis of a heterogeneous continuum traffic flow model. Appl. Math. Model. 94, 369–387 (2021)
Kerner, B.S., Konhäuser, P.: Cluster effect in initially homogeneous traffic flow. Phys. Rev. E 48(4), R2335–R2338 (1993)
Cao, J.F., Han, C.Z., Fang, Y.W.: Nonlinear Systems Theory and Application. Xi’an Jiao Tong University Press, Xi’an (2006)
Herrmann, M., Kerner, B.S.: Local cluster effect in difference traffic flow models. Phys. A 255, 163–188 (1998)
Igarashi, Y., Itoh, K., Nakanishi, K., et al.: Bifurcation phenomena in optimal velocity model for traffic flows. Phys. Rev. E 64, 047102 (2001)
Acknowledgements
This work is supported by the National Natural Science Foundation of China (Grant No. 11962002 & 11902083 & 12072195), the Natural Science Foundation of Guangxi, China (Grant No. 2018GXNSFAA138205) and Innovation Project of Guangxi Graduate Education (YCBZ2021021).
Author information
Authors and Affiliations
Corresponding author
Ethics declarations
Conflict of interest
The authors declare that they have no known competing financial interests or personal relationships that could have appeared to influence the work reported in this paper.
Additional information
Publisher's Note
Springer Nature remains neutral with regard to jurisdictional claims in published maps and institutional affiliations.
Rights and permissions
Springer Nature or its licensor (e.g. a society or other partner) holds exclusive rights to this article under a publishing agreement with the author(s) or other rightsholder(s); author self-archiving of the accepted manuscript version of this article is solely governed by the terms of such publishing agreement and applicable law.
About this article
Cite this article
Cen, BL., Xue, Y., Qiao, YF. et al. Global stability and bifurcation of macroscopic traffic flow models for upslope and downslope. Nonlinear Dyn 111, 3725–3742 (2023). https://doi.org/10.1007/s11071-022-08032-y
Received:
Accepted:
Published:
Issue Date:
DOI: https://doi.org/10.1007/s11071-022-08032-y