Abstract
The gas–water seepage parameters of coal reservoir have to do with the development effect of coalbed methane (CBM) wells, and determine the output characteristics of fluid in coal. The gas–water seepage parameters of coals in Jincheng coal mining area under the condition of gas-driven water was tested by using an unsteady-state method. The variation law of the relative permeability (Kr) of gas–water in coal with gas saturation is analyzed, and the gas–water seepage “triangle area” is proposed to clarify the seepage characteristics of coal reservoir. The impact of wettability and pore structure on fluid seepage and the output law of gas–water in coal during CBM drainage are revealed. It is indicated that the gas-phase permeability (Kg) of four specimens ranges from 0.021 × 10−3 to 0.068 × 10−3 μm2, and the water-phase permeability (Kw) ranges from 0.001 × 10−3 to 0.041 × 10−3 μm2. With increase in gas saturation (Sg), the relative permeability of the gas-phase (Krg) increases and that of the water-phase (Krw) decreases. The characteristic parameters of the gas–water seepage curve exhibit that Krg at the irreducible water is high, the relative permeability at the crossover point is low, Krw at the residual gas is high, the span of two-phase flow is narrow, and the saturation of residual gas is low. The high-rank coal is strong hydrophilic. With increase in vitrinite reflectance, the wetting angle increases linearly, and Krw increases. With increase in porosity, the saturation of irreducible water in two-phase flow decreases and the span of two-phase flow increases. The irreducible water saturation increases with increase in specific surface area; the span of two-phase flow is decreasing. Gas–water seepage in the dewatering and depressurization process of CBM wells will greatly reduce the drainage efficiency. Based on the current situation of CBM well drainage, the conditions and evaluation models of gas–water production are given, and the laws of gas–water seepage in CBM well production are revealed. After coal reservoir pressure drops to the critical desorption pressure, the water yield in CBM well goes down rapidly, while the gas yield goes up rapidly, and Kw drops continuously, which is caused by the gas–water seepage in coal.










Similar content being viewed by others
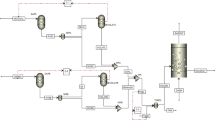
References
Brooks, R. H., & Corey, A. T. (1966). Properties of porous media affecting fluid flow. Journal of the Irrigation and Drainage Division, 92, 61–88.
Chen, D., Pan, Z., Liu, J., & Connell, L. D. (2013). An improved relative permeability model for coal reservoirs. International Journal of Coal Geology, 109, 45–57.
Clarkson, C. R., & Bustin, R. M. (2011). Coalbed methane: current field-based evaluation methods. SPE Reservoir Evaluation and Engineering, 14, 60–75.
Clarkson, C. R., Rahmanian, M., Kantzas, A., & Morad, K. (2011). Relative permeability of CBM reservoirs: Controls on curve shape. International Journal of Coal Geology, 88(4), 204–217.
Dabbous, M. K., Reznik, A. A., Taber, J. J., & Fulton, P. F. (1974). The permeability of coal to gas and water. Old SPE Journal, 14(6), 563–572.
Durucan, S., Ahsan, M., Shi, J. Q., Syed, A., & Korre, A. (2014). Two phase relative permeabilities for gas and water in selected European coals. Fuel, 134, 226–236.
Gray, I. (1987). Reservoir engineering in coal seams: Part 1-the physical process of gas storage and movement in coal seams. SPE Reservoir Engineering, 2, 28–34.
Ham, Y. S., & Kantzas, A. (2008). Measurement of relative permeability of coal: approaches and limitations. In Society of Petroleum Engineers Gas Technology Symposium 2008 Joint Conference, Alberta pp. 16–19.
Hassler, G. L. (1944). Method and apparatus for permeability measurements. US2345935,01-01.
Hotot, B. B. (1961). Coal and gas outburst (Song, S.Z., Wang, Y.A., Trans). China Coal Industry Publishing House.
Johnson, E. F., Bossler, D. P., & Naumann, V. O. (1959). Calculation of relative permeability from displacement experiments. Transactions of the AIME, 216, 370–372.
Jones, A. H., Bell, G. J., & Schraufnagel, R. A. (1988). A review of the physical and mechanical properties of coal with implications for coalbed methane well completion and production. 1988 Coalbed Methane, San Juan Basin, Rocky Mountain Association of Geologists. Wyoming, pp. 169–182.
Li, A. F. (2011). Fundaments of petrophysics- (3rd ed.). China University of Petroleum Press.
Li, Q. (1996). Evaluation of wettability of reservoir rock surface by relative permeability method. Petroleum Experimental Geology (in Chinese), 18(4), 454–458.
Liu, S. M., Wang, Y., & Harpalani, S. (2016). Anisotropy characteristics of coal shrinkage/swelling and its impact on coal permeability evolution with CO2 injection. Greenhouse Gases-Science and Technology, 6, 615–632.
Meaney, K., & Paterson, L. (1996). Relative permeability in coal. In Proceedings of the SPE Asia Pacific oil and gas conference, Adelaide, Australia, 28–31 October 1996; Society of Petroleum Engineers: Adelaide, Australia, p. 6.
Meng, Y., & Li, Z. P. (2018). Experimental comparisons of gas adsorption, sorption induced strain, diffusivity and permeability for low and high rank coals. Fuel, 234, 914–923.
Meng, Y., Li, Z. P., & Lai, F. P. (2015). Experimental study on porosity and permeability of anthracite coal under different stresses. Journal of Petroleum Science and Engineering, 133, 810–817.
Meng, Z. P., & Li, G. Q. (2013). Experimental research on the permeability of high-rank coal under a varying stress and its influencing factors. Engineering Geology, 162, 108–117.
Morse, R. A., Terwilliger, P. L., & Yuster, S. T. (1947). Relative permeability measurements on small core samples. The Procedures Monthly, 8, 19–25.
Paterson, L., Meaney, K., & Smyth, M. (1992). Measurements of relative permeability, absolute permeability and fracture geometry in coal. In 1992 Coalbed methane symposium. Townsville, pp. 19–21.
Peng, Z. Y., Li, X. F., & Sun, Z. (2020). A relative permeability model for CBM reservoir. Oil & Gas Science and Technology-Revue IFP Energies Nouvelles, 75(2), 1–9.
Rapoport, L. A., & Leas, W. J. (1951). Relative permeability to liquid in liquid–gas systems. Journal of Petroleum Technology, 3(3), 83–98.
Rice, D., & Flores, R. M. (1991). Controls of bacterial gas accumulations in thick Tertiary coal beds and adjacent channel sandstones, Powder River Basin Wyoming and Montana. AAPG Bulletin, 75, 661.
Schembre, J. M., & Kovscek, A. R. (2003). A technique for measuring two-phase relative permeability in porous media via X-ray CT measurements. Journal of Petroleum Science and Engineering, 39, 159–174.
Seidle, J. (2011). Fundamentals of coalbed methane reservoir engineering (pp. 166–172). PennWell Books.
Shaw, D., Mostaghimi, P., & Armstrong, R. (2019). The dynamic behaviour of coal relative permeability curves. Fuel, 253, 293–304.
Shen, J., Qin, Y., Wang, G. X., Fu, X., Wei, C., & Lei, B. (2011). Relative permeabilities of gas and water for different rank coals. International Journal of Coal Geology, 86, 266–275.
Shen, S. C., Fang, Z. M., & Li, X. C. (2020). Laboratory measurements of the relative permeability of coal: A review. Energies, 13(21), 5568.
Sun, X. X., Yao, Y. B., Ripepi, N., & Liu, D. M. (2018). A novel method for gas–water relative permeability measurement of coal using NMR relaxation. Transp Porous Med., 124, 73–90.
Tian, X. H. (2016). Research on tight gas reservoirs evaluation and classification of Benxi formation-Shanxi formation in eastern Ordos Basin. Master dissertation, Northwest University.
Wang, Z., Liu, S., & Qin, Y. (2021). Coal wettability in coalbed methane production: A critical review. Fuel, 303(1), 121277.
Xu, J. C., Guo, C. H., Jiang, R. Z., & Wei, M. Z. (2016). Study on relative permeability characteristics affected by displacement pressure gradient: Experimental study and numerical simulation. Fuel, 163, 314–323.
Yan, J. W., Meng, Z. P., Zhang, K., Yao, H. F., & Hao, H. J. (2020). Pore distribution characteristics of various rank coals matrix and their influences on gas adsorption. Journal of Petroleum Science and Engineering, 189, 107041.
Zhang, J. Y., Feng, Q. H., Zhang, X. M., & Wen, S. M. (2015). Relative permeability of coal: A review. Transport Porous Media, 106(3), 563–594.
Zhang, K., Meng, Z. P., Liu, S. M., & Hao, H. J. (2021). Laboratory investigation on pore characteristics of coals with consideration of various tectonic deformations. Journal of Natural Gas Science and Engineering, 91, 103960.
Zhao, T. T., Xu, H., Tang, D. Z., & Zong, P. (2021). A new relative permeability model of coal reservoir considering interface effect. Journal of Petroleum Science and Engineering, 207, 109082.
Zhao, W., Wang, K., Ju, Y., Fan, L., Cao, H., Yang, Y., Shu, L. Y., Feng, Z. K., Cui, R., Guo, X. F., & Wang, L. Y. (2023). Quantification of the asynchronous gas diffusivity in macro-/micropores using a Nelder–Mead simplex algorithm and its application on predicting desorption-based indexes. Fuel, 332, 126149.
Acknowledgments
This study was financially supported by the National Natural Science Foundation of China (Nos. 42002181, 42372192 and U1910205), Shanxi Province science and technology plan joint unveiling project (No. 20201101002). The authors also thank the reviewers and editors for their constructive comments and suggestions on improving this manuscript.
Author information
Authors and Affiliations
Corresponding author
Ethics declarations
Conflict of Interest
The authors have no competing interests to declare that are relevant to the content of this article.
Rights and permissions
Springer Nature or its licensor (e.g. a society or other partner) holds exclusive rights to this article under a publishing agreement with the author(s) or other rightsholder(s); author self-archiving of the accepted manuscript version of this article is solely governed by the terms of such publishing agreement and applicable law.
About this article
Cite this article
Meng, Y., Li, Z., Tang, S. et al. Experimental Study on Gas–Water Seepage in High-Rank Coal and Its Research Significance. Nat Resour Res 32, 2867–2881 (2023). https://doi.org/10.1007/s11053-023-10278-1
Received:
Accepted:
Published:
Issue Date:
DOI: https://doi.org/10.1007/s11053-023-10278-1