Abstract
The recent effects of climate change and rising global warming levels have increased the need to transition towards clean energy. The use of multi-energy systems is one of the potential solutions to these issues, as validated in the literature. The production of hydrogen from cleaner sources has an integral role in decarbonizing the industrial, building, and transportation sectors. Hence, this study proposes novel multi-energy systems that can produce hydrogen from wind resources. The study is novel as it developed an innovative multi-energy system configuration and also considers hydrogen production as a means to utilize excess wind power production. The intermittency of wind resources has been a major drawback in using this renewable energy as the singular source for multi-generation systems. The multi-energy configuration developed and analyzed in this study proposed a solution for this by integrating a regenerative reheat biomass integrated power cycle as an auxiliary system for the multi-generation system (Wind-Bio-MGS). This system is modeled to produce electricity, heating, hot water, and hydrogen. The energy, exergy, and exergoeconomic approach is adopted in this study to evaluate the steady-state performance of the system, while the levelized cost of electricity (LCOE), levelized cost of heating (LCOh), and levelized cost of Hydrogen (LCOH) are also computed. A multi-objective optimization of the overall exergy efficiency and total product unit cost is presented. The parametric analysis of the energy systems is included to show the sensitivity of different parameters to changes and validate the robustness of the modeled system. The results show that the integration of hydrogen with wind-based multi-generation systems is a viable means of reducing carbon emissions and global warming. The overall energy and exergy efficiencies of the Wind-Bio-MGS system are 69.13% and 31.16%, respectively. The LCOE, LCOh, and LCOH for the system, respectively, are 0.02828 $ kWh−1, 0.004038 $ kWh−1, and 1.311 $ kg−1 s−1.
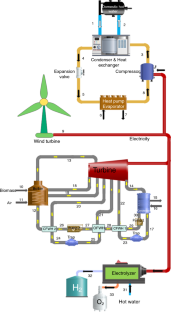



Similar content being viewed by others
Abbreviations
- AWE:
-
Alkaline water electrolyzer
- BC:
-
Brayton cycle
- \(\dot{{\text{C}}}\) :
-
Specific cost
- CFWH:
-
Closed feedwater heater
- CO2 :
-
Carbon dioxide
- COP:
-
Coefficient of performance
- \({\text{ex}}\) :
-
Specific exergy
- EES:
-
Engineering equation solver
- GHG:
-
Greenhouse gas
- GW:
-
Gigawatts
- h:
-
Specific enthalpy
- H2 :
-
Hydrogen
- HP:
-
Heat pump
- HWH:
-
Hot water heater
- LCOh:
-
Levelized cost of heating
- LCOE:
-
Levelized cost of electricity
- LCOH:
-
Levelized cost of hydrogen
- LCOW:
-
Levelized cost of water
- LFC:
-
Linear Fresnel concentrator
- kg:
-
Kilogram
- kg m−3 :
-
Kilogram per cubic meter
- kg s−1 :
-
Kilogram per second
- kPa:
-
Kilopascal
- kW:
-
Kilowatts
- \(\dot{m}\) :
-
Mass flow rate (kg s−1)
- m2 :
-
Meters squared
- m/s:
-
Meters per second
- MED:
-
Multi-effect desalination
- MGES:
-
Multi-generation energy system
- MSW:
-
Municipal solid waste
- MW:
-
Megawatts
- OFWH:
-
Open feedwater heater
- ORC:
-
Organic Rankine cycle
- PEM:
-
Proton exchange membrane
- PTC:
-
Parabolic trough collectors
- LHV:
-
Lower heating value
- \(\dot{Q}\) :
-
Heat transfer rate (kW)
- RC:
-
Rankine cycle
- RES:
-
Renewable energy sources
- s:
-
Specific entropy
- SOFC:
-
Solid-oxide fuel cell
- SRC:
-
Steam Rankine cycle
- TS/MGS:
-
Two-source multi-generation system
- \(\dot{W}\) :
-
Work done/power
- \(\dot{Z}\) :
-
Exergy unit cost
- \(\eta\) :
-
Efficiency
- Ѱ :
-
Exergy efficiency
- $ kg−1 :
-
Dollar per kilogram
- $ kWh−1 :
-
Dollar per kilowatts hour
- kg h−1 :
-
Kilogram per hour
- $ h−1 :
-
Dollar per hour
- Cond:
-
Condenser
- comp:
-
Compressor
- Dest:
-
Destruction
- Elect:
-
Electricity
- Evap:
-
Evaporator
- HWH:
-
Hot water heater
- HEX:
-
Heat exchanger
- P.E.M:
-
Proton exchange membrane
- Turb:
-
Turbine
References
IPCC. Climate change 2021: the physical science basis. Cambridge: Cambridge University Press, 2021. https://doi.org/10.1017/9781009157896.
IEA. World energy outlook 2020. IEA (2020) https://www.iea.org/reports/world-energy-outlook-2020. Accessed 05, March, 2024
IEA. Renewables 2020. IEA, 2020. Available: https://www.iea.org/reports/renewables-2020. Accessed 08 Nov 2023
UNFCC. The Paris agreement. Paris, 2016. https://unfccc.int/sites/default/files/english_paris_agreement.pdf. Accessed 05 March 2024.
IRENA. World energy transitions outlook: 1.5 °C pathway. In: International renewable energy agency, Abu Dhabi, 2021. www.irena.org/publications. Accessed 05 March 2024.
IRENA. Renewable capacity statistics 2021. In International renewable energy agency, Abu Dhabi, 2021, www.irena.org/publications. Accessed: 05 March 2024
IEA. Achieving net zero heavy industry sectors in G7 members. IEA, Paris, 2022. https://www.iea.org/reports/achieving-net-zero-heavy-industry-sectors-in-g7-members. Accessed 05 March 2024
IEA. Energy technology perspectives 2020. IEA, Paris, 2020. Available: https://www.iea.org/reports/energy-technology-perspectives-2020. Accessed 05 March 2024
Hassan Q, Sameen AZ, Salman HM, Jaszczur M, Al-Jiboory AK. Hydrogen energy future: advancements in storage technologies and implications for sustainability. J Energy Storage. 2023;72:108404. https://doi.org/10.1016/j.est.2023.108404.
Ferrada F, Babonneau F, Homem-de-Mello T, Jalil-Vega F. The role of hydrogen for deep decarbonization of energy systems: a Chilean case study. Energy Policy. 2023;177:113536. https://doi.org/10.1016/j.enpol.2023.113536.
Rasul MG, Hazrat MA, Sattar MA, Jahirul MI, Shearer MJ. The future of hydrogen: challenges on production, storage and applications. Energy Convers Manag. 2022;272:116326. https://doi.org/10.1016/j.enconman.2022.116326.
Ishaq H, Dincer I, Crawford C. A review on hydrogen production and utilization: challenges and opportunities. Int J Hydrogen Energy. 2022;47(62):26238–64. https://doi.org/10.1016/j.ijhydene.2021.11.149.
Bamisile O, Cai D, et al. An innovative approach for geothermal-wind hybrid comprehensive energy system and hydrogen production modelling/process analysis. International Journal of Hydrogen Energy. 2022;47:27, p13261–13288. https://doi.org/10.1016/j.ijhydene.2022.02.084
Coelho A, Neyestani N, Soares F, Lopes JP. Wind variability mitigation using multi-energy systems. Int J Electr Power Energy Syst. 2020;118:105755. https://doi.org/10.1016/j.ijepes.2019.105755.
Mahdavi M, Jurado F, Ramos RAV, Awaafo A. Hybrid biomass, solar and wind electricity generation in rural areas of Fez–Meknes region in Morocco considering water consumption of animals and anaerobic digester. Appl Energy. 2023;343:121253. https://doi.org/10.1016/j.apenergy.2023.121253.
Lak Kamari M, Maleki A, Daneshpour R, Rosen MA, Pourfayaz F, Alhuyi Nazari M. Exergy, energy and environmental evaluation of a biomass-assisted integrated plant for multigeneration fed by various biomass sources. Energy. 2023;263:125649. https://doi.org/10.1016/j.energy.2022.125649.
Lykas P, Bellos E, Kitsopoulou A, Tzivanidis C. Dynamic analysis of a solar-biomass-driven multigeneration system based on s-CO2 Brayton cycle. Int J Hydrogen Energy. 2024;59:1268–86. https://doi.org/10.1016/j.ijhydene.2024.02.093.
Sharifishourabi M, Dincer I, Mohany A. Performance assessment of a novel solar heliostat and digester-based multigeneration system with hydrogen production. Appl Therm Eng. 2023;232:121094. https://doi.org/10.1016/j.applthermaleng.2023.121094.
Tian L, Zhang Z, Salah B, Marefati M. Multi-variable assessment/optimization of a new two-source multigeneration system integrated with a solid oxide fuel cell. Process Saf Environ Prot. 2023;179:754–73. https://doi.org/10.1016/j.psep.2023.08.003.
Ghasemi A, Nikafshan Rad H, Akrami M, Marefati M. Exergoeconomic and exergoenvironmental analyzes of a new biomass/solar-driven multigeneration energy system: an effort to maximum utilization of the waste heat of gasification process. Therm Sci Eng Prog. 2024;48:102407. https://doi.org/10.1016/j.tsep.2024.102407.
Nasrabadi AM, Korpeh M. Techno-economic analysis and optimization of a proposed solar-wind-driven multigeneration system; case study of Iran. Int J Hydrogen Energy. 2023;48(36):13343–61. https://doi.org/10.1016/j.ijhydene.2022.12.283.
Mohammadi K, McGowan JG, Powell K. Thermoeconomic analysis of a multigeneration system using waste heat from a triple power cycle. Appl Therm Eng. 2021;190:116790. https://doi.org/10.1016/j.applthermaleng.2021.116790.
Lin H, Liu J, Ifseisi AA, Taghavi M. A novel bio-waste-driven multigeneration cycle integrated with a solar thermal field and atmospheric water harvesting cycle: an effort to mitigate the environmental impacts of the wastewater treatment plants. Process Saf Environ Prot. 2023;180:386–403. https://doi.org/10.1016/j.psep.2023.09.066.
Habib MA, Zubair SM. Second-law-based thermodynamic analysis of regenerative-reheat Rankine-cycle power plants. Energy. 1992;17(3):295–301. https://doi.org/10.1016/0360-5442(92)90057-7.
Bamisile O, Huang Q, Dagbasi M, Abid M, Okafor EC, Ratlamwala TAH. Concentrated solar powered novel multi-generation system: a energy, exergy, and environmental analysis. J Sol Energy Eng. 2020;142(5):051005–17. https://doi.org/10.1115/1.4046392.
Bamisile OO, Dagbasi M, Abbasoglu S. Energy and exergy analyses of a novel solar PTC assisted multi-generation system. Int J Exergy. 2019;28(2):158–82. https://doi.org/10.1504/IJEX.2019.097978.
Bamisile O, Huang Q, Hu W, Dagbasi M, Kemena AD. Performance analysis of a novel solar PTC integrated system for multi-generation with hydrogen production. Int J Hydrog Energy. 2020;45(1):190–206. https://doi.org/10.1016/j.ijhydene.2019.10.234.
Bamisile O, et al. Modelling and performance analysis of an innovative CPVT, wind and biogas integrated comprehensive energy system: An energy and exergy approach. Energy Convers Manag. 2020;209:112611. https://doi.org/10.1016/j.enconman.2020.112611.
Cengel Y, Boles M. Thermodynamics: an engineering approach. 8th ed. New York: McGraw-Hill Education; 2014.
Bamisile O, et al. An innovative approach for geothermal-wind hybrid comprehensive energy system and hydrogen production modeling/process analysis. Int J Hydrog Energy. 2022;47(27):13261–88. https://doi.org/10.1016/j.ijhydene.2022.02.084.
Bamisile O, et al. Environmental impact of hydrogen production from Southwest China’s hydro power water abandonment control. Int J Hydrog Energy. 2020;45(46):25587–98. https://doi.org/10.1016/j.ijhydene.2020.06.289.
Acknowledgements
This study was supported by National Natural Science Foundation of China (Grant No. 52007025), Sichuan Provincial Key Lab for Power System-Wide Area Measurement (Grant No. 2021KP012), Science and Technology Innovation Talent Program of Sichuan Provincial (Grant No. 22CJDRC0025), and Science and Technology Innovation Talent Program of Sichuan Provincial (Grant No. 22CXRC0010).
Author information
Authors and Affiliations
Corresponding author
Ethics declarations
Conflict of interest
The authors declare that they have no known competing financial interests or personal relationships that could have appeared to influence the work reported in this paper. The authors declare the following financial interests/personal relationships which may be considered as potential competing interests.
Additional information
Publisher's Note
Springer Nature remains neutral with regard to jurisdictional claims in published maps and institutional affiliations.
Rights and permissions
Springer Nature or its licensor (e.g. a society or other partner) holds exclusive rights to this article under a publishing agreement with the author(s) or other rightsholder(s); author self-archiving of the accepted manuscript version of this article is solely governed by the terms of such publishing agreement and applicable law.
About this article
Cite this article
Acen, C., Bamisile, O., Adedeji, M. et al. Energy, exergy, and exergoeconomic cost optimization of wind-biomass multi-energy systems integrated for hydrogen production. J Therm Anal Calorim (2024). https://doi.org/10.1007/s10973-024-13135-2
Received:
Accepted:
Published:
DOI: https://doi.org/10.1007/s10973-024-13135-2