Abstract
Aerogel is a kind of nanoscopic materials aggregated from thermal insulating material with its thermal properties substantially enhanced. Considering the high price, a small amount of aerogel (0 –8 mass%) has been added into geopolymer pastes to produce aerogel-enhanced porous geopolymers (APG) with thermal conductivity < 0.055 W·(m·K)−1. The effects of aerogel on viscosity, geopolymerization, stability, bulk density, mechanical and thermal properties of geopolymers have been studied. Results show that the addition of aerogel increases the viscosity of APG pastes and aerogel could stably exist in APG without reacting with alkaline activator within. Due to its frangibility, overdosed aerogel (> 8 mass%) would result in a decline of compressive (σ) and flexural strength (δ) of APG to lower than 0.3 MPa and 0.2 MPa, respectively. However, a desired content of aerogel (about 6 mass%) could reduce the thermal conductivity (λ) of APG to 0.05 W· (m · K)−1 and keep the mechanical properties satisfying the standard of JC/T 2200-2013 (σ > 0.3 MPa and δ > 0.2 MPa for 180 kg · m−3) in the same time. The heat transfer simulation indicates that aerogel could enhance the thermal resistance of APG in a temperature difference of 25 °C.











Similar content being viewed by others
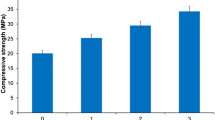
References
Mckinsey Company. Pathways to a low carbon economy. Version 2 of the global GHG Abatement Cost Curve. Mckinsey & Company. 2009.
Al-Homoud MS. Performance characteristics and practical applications of common building thermal insulation materials. Build Environ. 2005;40(3):353–66. https://doi.org/10.1016/j.buildenv.2004.05.013.
Papadopoulos AM. State of the art in thermal insulation materials and aims for future developments. Energ. Build. 2005;37(1):77–86. https://doi.org/10.1016/j.enbuild.2004.05.006.
Jelle BP. Traditional, state-of-the-art and future thermal building insulation materials and solutions - Properties, requirements and possibilities. Energ. Build. 2011;43(10):2549–63. https://doi.org/10.1016/j.enbuild.2011.05.015.
Jelle BP, Gustavsen A, Baetens R. The path to the high performance thermal building insulation materials and solutions of tomorrow. J. Build. Phys. 2010;34(2):99–123. https://doi.org/10.1177/1744259110372782.
Jelle BP, Gustavsen A, Grynning S., et al. Nanotechnology and possibilities for the thermal building insulation materials of tomorrow. Proceedings of the Renewable Energy Research Conference –Renewable Energy Beyond 2020. 2010. Trondheim, Norway.
Cuce E, Cuce PM, Wood CJ, et al. Toward aerogel based thermal superinsulation in buildings: a comprehensive review. Renew. Sust. Energ. Rev. 2014;34:273–99. https://doi.org/10.1016/j.rser.2014.03.017.
Baetens R, Jelle BP, Gustavsen A. Aerogel insulation for building applications: a state-of-the-art review. Energ. Build. 2011;43(4):761–9. https://doi.org/10.1016/j.enbuild.2010.12.012.
Lakatos A. Investigation of the thermal insulation performance of fibrous aerogel samples under various hygrothermal environment: laboratory tests completed with calculations and theory. Energy Build. 2020;214:1–9. https://doi.org/10.1016/j.enbuild.2020.109902.
Barbero S, Dutto M, Ferrua C, et al. Analysis on existent thermal insulating plasters towards innovative applications: evaluation methodology for a real cost-performance comparison. Energ. Build. 2014;77:40–7. https://doi.org/10.1016/j.enbuild.2014.03.037.
Gao T, Jelle BP, Gustavsen A, et al. Aerogel-incorporated concrete: an experimental study. Constr Build Mater. 2014;52:130–6. https://doi.org/10.1016/j.conbuildmat.2013.10.100.
Kim S, Seo J, Cha J, et al. Chemical retreating for gel-typed aerogel and insulation performance of cement containing aerogel. Constr Build Mater. 2013;40:501–5. https://doi.org/10.1016/j.conbuildmat.2012.11.046.
de Julio MF, Soares A, Ilharco LM, et al. Aerogel-based renders with lightweight aggregates: Correlation between molecular/pore structure and performance. Constr Build Mater. 2016;124:485–95. https://doi.org/10.1016/j.conbuildmat.2016.07.103.
Fickler S, Milow B, Ratke L, et al. Development of high performance aerogel concrete. Energ Procedia. 2015;78:406–11.
Ng S, Jelle BP, Zhen Y, et al. Effect of storage and curing conditions at elevated temperatures on aerogel-incorporated mortar samples based on UHPC recipe. Constr Build Mater. 2016;106:640–9. https://doi.org/10.1016/j.conbuildmat.2015.12.162.
Ng S, Jelle BP, Sandberg LIC, et al. Experimental investigations of aerogel-incorporated ultra-high performance concrete. Constr Build Mater. 2015;77:307–16. https://doi.org/10.1016/j.conbuildmat.2014.12.064.
de Julio MF, Soares A, Ilharco LM, et al. Silica-based aerogels as aggregates for cement-based thermal renders. Cem. Con. Comp. 2016;72:309–18. https://doi.org/10.1016/j.cemconcomp.2016.06.013.
Westgate P, Paine K, Ball RJ. Physical and mechanical properties of plasters incorporating aerogel granules and polypropylene monofilament fibres. Constr Build Mater. 2018;158:472–80. https://doi.org/10.1016/j.conbuildmat.2017.09.177.
Nosrati RH, Berardi U. Hygrothermal characteristics of aerogel-enhanced insulating materials under different humidity and temperature conditions. Energ. Build. 2018;158:698–711. https://doi.org/10.1016/j.enbuild.2017.09.079.
Cui Y, Wang D, Zhao J, et al. Thermal and Mechanical Properties of SiO2 Aerogel-Incorporated Geopolymer Insulation Materials. J Mater Civil Eng. 2019;31(7):1–10. https://doi.org/10.1061/(ASCE)MT.1943-5533.0002730.
Garcia-Lodeiro I, Fernandez-Jimenez A, Palomo A. Hydration kinetics in hybrid binders: early reaction stages. Cem Con Comp. 2013;39:82–92. https://doi.org/10.1016/j.cemconcomp.2013.03.025.
Fernandez-Jimenez A, Palomo A, Criado M. Microstructure development of alkali-activated fly ash cement: a descriptive model. Cem Con Res. 2005;35(6):1204–9. https://doi.org/10.1016/j.cemconres.2004.08.021.
Criado M, Fernandez-Jimenez A, Palomo A. Alkali activation of fly ash. Part III. Effect of curing conditions on reaction and its graphical description. Fuel. 2010;89(11):3185–92. https://doi.org/10.1016/j.fuel.2010.03.051.
Yao X, Zhang Z, Zhu H, et al. Geopolymerization process of alkali-metakaolinite characterized by isothermal calorimetry. Thermochim Acta. 2009;493(1–2):49–54. https://doi.org/10.1016/j.tca.2009.04.002.
Zhang Z, Provis JL, Wang H, et al. Quantitative kinetic and structural analysis of geopolymers. Part 2. Thermodynamics of sodium silicate activation of metakaolin. Thermochim. Acta. 2013;565:163–71. https://doi.org/10.1016/j.tca.2013.01.040.
Dimas D, Giannopoulou I, Panias D. Polymerization in sodium silicate solutions: a fundamental process in geopolymerization technology. J Mater Sci. 2009;44(14):3719–30. https://doi.org/10.1007/s10853-009-3497-5.
Esmaily H, Nuranian H. Non-autoclaved high strength cellular concrete from alkali activated slag. Constr Build Mater. 2012;26(1):200–6. https://doi.org/10.1016/j.conbuildmat.2011.06.010.
Kamseu E, Ngouloure ZNM, Ali BN, et al. Cumulative pore volume, pore size distribution and phases percolation in porous inorganic polymer composites: relation microstructure and effective thermal conductivity. Energ. Build. 2015;88:45–56. https://doi.org/10.1016/j.enbuild.2014.11.066.
Funding
This research was funded by the scientific and technological projects of Shanxi Province (MC2014-04) and the open fund of Key Laboratory of Thermal Science and Power Engineering of Ministry of Education.
Author information
Authors and Affiliations
Contributions
Conceptualization, DW.; methodology, DW. and DL.; writing—original draft preparation, DL. and YC.; writing—review and editing, DL. and DW.; supervision, DW.. All authors have read and agreed to the published version of the manuscript.
Corresponding author
Ethics declarations
Conflict of interest
The authors declare no conflict of interest.
Additional information
Publisher's Note
Springer Nature remains neutral with regard to jurisdictional claims in published maps and institutional affiliations.
Rights and permissions
About this article
Cite this article
Li, D., Wang, D. & Cui, Y. Study on pore structure and thermal conductivity of aerogel enhanced porous geopolymers. J Therm Anal Calorim 147, 1061–1070 (2022). https://doi.org/10.1007/s10973-020-10389-4
Received:
Accepted:
Published:
Issue Date:
DOI: https://doi.org/10.1007/s10973-020-10389-4