Abstract
This work reports the results of an extended kinetic study involving both experimental measurements and modelling elaborations. It is specifically dedicated to investigate the thermal behaviour of biomasses undergoing to torrefaction treatment. Three biomasses, representative of the hardwood family, have been considered: ash-wood, beech-wood and hornbeam. As main purpose, this work evaluates the impact of the TG measurements on the Activation Energy (E a) results achieved by implementing the so-called isoconversional model-free methods on both their differential and integral version. Considering the heterogeneous nature of the biomasses and the thermo-chemical factors conditioning the involved solid-state reactions, several replicates of the TG data sets have been carried out and their impact on the E a reliability has been evaluated. An extended sensitivity analysis of the adopted models allows to identify a Confidential Boundary Range of the TG measurements and, in correspondence, an Activation Energy Boundary Range for the E a results. Considering the “model-free” nature of these methods, a preliminary selection of a kinetic scheme is not required, making particularly attractive this approach to match the purpose of this research. This study provides even a comparison among the models performances and a review of their application limits. Applied to heterogeneous materials, the proposed approach could be considered a general methodology to test the impact of the TG measurements on the Activation Energy results reliability.







Similar content being viewed by others
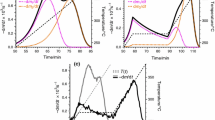
References
Prins MJ, Ptasinski KJ, Janssen FJJG. More efficient biomass gasification via torrefaction. Energy. 2006;31(15):3458–70.
Pimchuai A, Dutta A, Basu P. Torrefaction of agriculture residue to enhance combustible properties. Energy Fuels. 2010;24(9):4638–45.
Couhert C, Salvador S, Commandre JM. Impact of torrefaction on syngas production from wood. Fuel. 2009;88(11):2286–90.
Deng J, Wang G, Kuang J, Zhang Y, Luo Y. Pretreatment of agricultural residues for co-gasification via torrefaction. J Anal Appl Pyrolysis. 2009;86(2):331–7.
Miao Z, Shastri Y, Grift TE, Hansen AC, Ting KC. Lignocellulosic biomassfeedstock transportation alternatives, logistics, equipment configurations, and modelling. Biofuels Bioproducts Biorefining-BIOFPR. 2012;6:351–62.
Rentizelas A, Tolis A, Tatsiopoulos IP. Logistics issues of biomass: the storage problem and the multi-biomass supply chain. Renew Sustain Energy Rev. 2009;13(4):887–94.
Nordin A. The dawn of torrefaction BE-sustainable—the magazine of bioenergy and the bioeconomy. 2012; p. 21–3.
Uslu A, Faaij APC. Bergman PCA. Pre-treatment technologies, and their effect on international bioenergy supply chain logistics. Techno-economic evaluation of torrefaction, fast pyrolysis and pelletisation. Energy. 2008;33(8):1206–23.
Brouwers JJM. Commercialisation Torr-coal torrefaction technology. Sittard: Torr-Coal Group; 2011.
Delivand MK, Barz M, Gheewala SH. Logistics cost analysis of rice straw for biomass power generation in Thailand. Energy. 2011;36(3):1435–41.
Grigiante M, Antolini D. Mass yield as guide parameter of the torrefaction process. An experimental study of the solid fuel properties referred to two types of biomasses. Fuel. 2015;153:499–509.
Almeida G, Brito JO, Perre P. Alterations in energy properties of eucalyptus wood and bark subjected to torrefaction: the potential of mass loss as a synthetic indicator. Bioresource Technol. 2010;101:9778–84.
Batidzirai B, Mignot APR, Schakel WB, Junginger HM, Faaij APC. Biomass torrefaction technology: techno-economic status and future prospects. Energy. 2013;62:196–214.
Song H, Andreas J, Minhou X. Kinetic study of Chinese biomass slow pyrolysis: comparison of different kinetic models. Fuel. 2007;86:2778–88.
Zhu F, Feng Q, Xu Y, Liu R, Li K. Kinetics of pyrolysis of ramie fabric wastes from thermogravimetric data. J Thermal Anal Calorim. 2015;119:651–7.
Vyazovkin S. Evaluation of the activation energy of thermally stimulated solid state reactions under an arbitrary variation of the temperature. J Comput Chem. 1997;18:393–402.
Baroni EG, Tannous K, Rueda-Ordonez YJ, Tinoco-Navarro LK. The applicability of isoconversional models in estimating the kinetic parameters of biomass pyrolysis. J Thermal Anal Calorim. 2016;123:909–17.
Flynn JH, Wall LA. A quick, direct method for the determination of activation energy from thermogravimetric data. J Polym Sci Part B Polym Lett. 1966;4:323–8.
Orfao JJM, Antunes FJA, Figueiredo JL. Pyrolysis kinetics of lignocellulosic materials-three independent reactions model. Fuel. 1999;78:349–58.
Vyazovkin S, Burnham AK, Criado JM, Pérez-Maqueda LA, Popescu C, Sbirrazzuoli N. ICTAC Kinetics Committee recommendations for performing kinetic computations on thermal analysis data. Thermochim Acta. 2011;520:1–19.
Flynn JH. A general differential technique for the determination of parameters for d(α)/dt = f(α)A exp (-E/RT)—energy of activation, preexponential factor and order of reaction (when applicable). J Thermal Anal. 1991;37:293–305.
Vyazovkin S, Wight CA. Isothermal and non-isothermal kinetics of thermally stimulated reactions of solids. Int Rev Phys Chem. 1998;17:407–33.
AOAC method 930.15, Loss on drying (moisture) for feeds (at 135_C for 2 h)/dry matter on oven drying for feed (at 135_C for 2 h) official methods. In: Helrich K, editor. Official methods of analysis. 17th ed. Gaithersburg, MD: AOAC International; 2000.
DD CEN/TS 14775. Solid biofuels Method for the determination of ash content. London: BSI Publisher; 2004. p. 12.
Prins MJ, Ptasinski KJ. Jassen FJJG. Torrefaction of wood. Part 1. Weight loss kinetics. J Anal Appl Pyrolysis. 2006;77:28–34.
Starink MJ. Activation energy determination for linear heating experiments: deviations due to neglecting the low temperature end of the temperature integral. J Mater Sci. 2007;42:483–9.
Zsako J. Kinetic analysis of thermogravimetric data. V J Thermal Anal Calorim. 1973;5:239–51.
Zsako J. Kinetic analysis of thermogravimetric data. J Thermal Anal Calorim. 1996;46:1845–64.
Friedman HL. New methods for evaluating kinetic parameters from thermal analysis data. J Polym Sci Part B Polym Lett. 1969;7:41–6.
Friedman HL. Thermal degradation of plastics. I. The kinetics of polymer chain degradation. J Polym Sci. 1960;45:119–25.
Flynn JH, Wall LA. General treatment of the thermogravimetry of polymers. J Res Natl Bur Stand Part A. 1966;70:487–523.
Biagini E, Barontini F, Tognotti L. Devolatilization of biomass fuels and biomass components studied by TG/FTIR technique. Ind Eng Chem Res. 2006;45:4486–93.
Lyon RE. An integral method of nonisothermal kinetic analysis. Thermochim Acta. 1997;297:117–24.
Wang J, Zhao H. Error evaluation on pyrolysis kinetics of sawdust using iso-conversional methods. J Thermal Anal Calorim. 2016;124:6.
Senum GI, Yang RT. Rational approximations of the integral of the Arrhenius function. J Thermal Anal Calorim. 1977;11:445–7.
Murray P, White J. Kinetics of the thermal dehydration of clays. Part IV. Interpretation of the differential thermal analysis of the clay minerals. Trans Br Ceram Soc. 1955;54:204–38.
Starink MJ. A new method for the derivation of activation energies from experiments performed at constant heating rate. Thermochim Acta. 1996;288:97–104.
Starink MJ. The determination of activation energy from linear heating rate experiments: a comparison of the accuracy of isoconversion methods. Thermochim Acta. 2003;404:163–76.
Graydon JW, Thorpe SJ, Kirk DW. Interpretation of activation energies calculated from non-isothermal transformations of amorphous metals. Acta Metall et Mater. 1994;42:3163–6.
Doyle CD. Kinetic analysis of thermogravimetric data. J Appl Polym Sci. 1961;5:285–92.
Doyle CD. Estimating isothermal life from thermogravimetric data. JAppl Polym Sci. 1962;6:639–42.
Doyle CD. Series approximations to the equation of thermogravimetric data. Nature. 1965;207:290–1.
Yang H, Yan R, Chen H, Lee DH, Zheng C. Characteristic of hemicellulose, cellulose and lignin pyrolysis. Fuel. 2007;86:1781–8.
Bo LH, Yu ZG, Xia JC. Analysis on TG-FTIR and kinetics of biomass pyrolysis. International conference on sustainable power generation and supply 2009 (pp. 1–5).
Tumuluru JS, Sokhansanj S, Hess JR, Wright C, Boardman R. A review on biomass torrefaction process and product properties for energy applications. Ind Biotechnol. 2011;7:384–401.
Braga RM, Melo DMA, Aquino FM. Characterization and comparative study of pyrolysis kinetics of the rice husk and the elephant grass. J Thermal Anal Calorim. 2014;115:1915–20.
Brachi P, Francesco M, Michele M, Ruoppolo G. Isoconversional kinetic analysis of olive pomace decomposition under torrefaction operating conditions. Fuel Process Technol. 2015;130:147–54.
Vyazovkin S, Dollimore D. Linear and nonlinear procedures in isoconversional computations of the activation energy of thermally induced reactions in solids. J Chem Inf Comput Sci. 1996;36:42–5.
Biagini E, Fantei A, Tognotti L. Effect of the heating rate on the devolatilization of biomass residues. Thermochim Acta. 2008;472:55–63.
Benavente V, Fullana A. Torrefaction of olive mill waste. Biomass Bioenergy. 2015;73:186–94.
Damartzis TH, Vamvuka D, Sfakiotakis S, Zabaniotou A. Thermal degradation studies and kinetic modeling of cardoon (Cynara cardunculus) pyrolysis using thermogravimetric analysis (TG). Bioresour Technol. 2011;102:6230–8.
Chen W, Kuo P. Isothermal torrefaction kinetics of hemicellulose, cellulose, lignin and xylan using thermogravimetric analysis. Energy. 2011;36:6451–60.
Lasode OA, Balogunb AO, McDonald AG. Torrefaction of some Nigerian lignocellulosic resources and decomposition kinetics. J Anal Appl Pyrolysis. 2014;109:47–55.
Lopez-Velazquez MA, Santesa LV, Balmased J, Torres-Garciac E. Pyrolysisof orange waste: a thermo-kinetic study. J Anal Appl Pyrolysis. 2013;99:170–7.
Wang G, Li W, Li B, Chen H. TG study on pyrolysis of biomass and its three components under syngas. Fuel. 2008;87:552–8.
Golikeri SV, Luss D. Analysis of activation energy of grouped parallel reactions. AIChE J. 1972;18(2):277–82.
Brown ME, Gallagher PK. The Handbook of Thermal Analysis & Calorimetry. Vol. 5. Recent Advances, Techniques and Applications; 2008.
Vyazovkin S, Sbirrazzuoli N. Isoconversional kinetic analysis of thermally stimulated processes in polymers. Macromol Rapid Commun. 2006;27(18):1515–32.
Vyazovkin S. Modification of the integral isoconversional method to account for variation in the activation energy. J Comput Chem. 2001;22(2):178–83.
Author information
Authors and Affiliations
Corresponding author
Rights and permissions
About this article
Cite this article
Grigiante, M., Brighenti, M. & Antolini, D. Analysis of the impact of TG data sets on activation energy (E a). J Therm Anal Calorim 129, 553–565 (2017). https://doi.org/10.1007/s10973-017-6122-x
Received:
Accepted:
Published:
Issue Date:
DOI: https://doi.org/10.1007/s10973-017-6122-x