Abstract
A diamond mixed tunneling and avalanche transit-time diode is designed in this letter. Schottky contact is used in this kind of diode to reduce the contact resistance. Electrical characteristics of n-type diamond Schottky contact have been accurately investigated. Total output power of such transit-time diode is evaluated using an accurate large-signal model. The results indicate that the new type transit-time diode can operate with the frequency up to several terahertzes. The output power density is more than 1.185 MW/cm2 from 1.07 to 2.12THz. About 17% improvement in efficiency is found at 2.12THz.





Similar content being viewed by others
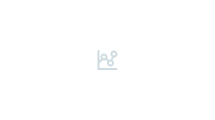
References
B. Ferguson, and X.-C. Zhang, Materials for terahertz science and technology. Nat. Materials 1, 26–33 (2002).
M. Tonouchi, Cutting-edge terahertz technology. Nat. Photonics 1, 99–105 (2007).
P.H. Siegel, Terahertz technology. IEEE Trans. MTT-50, 910–928 (2002).
H. Eisele, Active two-terminal devices as sources at THz frequencies: concepts, performance, and trends. Proc. SPIE 6257, 62570G (2006).
M.E. Elta, and G.I. Haddad, High-frequency limitations of IMPATT, MITATT, and TUNNETT mode devices. IEEE Trans. MTT-27, 442–449 (1979).
R.J. Trew, High-frequency solid-state electronic devices. IEEE Trans. ED-52, 638–649 (2005).
P.W. May, Diamond thin films: a 21st-century material. Phil. Trans. R. Soc. Lond. A 358, 473–495 (2000).
D.S. Hwang, T. Saito, and N. Fujimori, New etching process for device fabrication using diamond. Diamond and Related Materials 13, 2207–2210 (2004).
C.-C. Chen, R.K. Mains, G.I. Haddad, and H. Eisele, Structure and simulation of GaAs TUNNETT and MITATT devices for frequencies above 100 GHz. Conference on Advanced Concepts in High Speed Semiconductor Devices and Circuits, Cornell University, pp.194-202, (1993), August 2-4.
S.O. Scanlan, and T.J. Brazil, Large-signal computer simulation of IMPATT diodes. IEEE Trans. ED-28, 18–21 (1981).
P.M. Mock, Material and design parameter effects in IMPATT Diodes, Ph. D Thesis, North Carolina State University, pp.67-102, (1991)
R.J. Trew, J.B. Yan, and P.M. Mock, The potential of diamond and SiC electronic devices for microwave and millimeter-wave power applications. Proc. IEEE 79(5), 598–620 (1991).
K. Takahashi, A. Yoshikawa, and A. Sandhu, Wide bandgap semiconductors: fundamental properties and modern photonic and electronic devices. (Springer-Verlag, Berlin Heidelberg, 2007, )pp. 28–31.
M.W. Geis, N.N., Efremow, K.E. Krohn, and J.C. Twichell, Theory and experimental results of a new diamond surface-emission cathode. Linc. Lab. J. 10(1), 3–16 (1997).
H. Taniuchi, H. Umezawa, T. Arima, M. Tachiki, and H. Kawarada, High-frequency performance of diamond field-effect transistor. IEEE Electron Device Lett. 22, 390–392 (2001).
J. Isberg, J. Hammersberg, E. Johansson, and T. Wikström, High carrier mobility in single-crystal plasma-deposited diamond. Science 297, 1670–1672 (2002).
A. Vescan, I. Daumiller, P. Gluche, W. Ebert, and E. Kohn, Very high temperature operation of diamond schottky diode. IEEE Electron Device Lett. 18(11), 556–558 (1997).
M. Mukherjee, N. Mazumder, S.K. Roy, and K. Goswami, GaN IMPATT diode: a photo-sensitive high power terahertz source. Semicond. Sci. Technol. 22, 1258–1267 (2007).
Coherent Inc., http://www.coherent.com/
Microtech Instruments Inc., http://www.mtinstruments.com/
B.S. Williams, S. Kumar, Q. Hu, and J.L. Reno, Operation of terahertz quantum-cascade lasers at 164 K in pulsed mode and at 117 K in continuous-wave mode. Opt. Express 13, 3331–3339 (2005).
B.S. Williams, S. Kumar, Q. Hu, and J.L. Reno, High-power terahertz quantum cascade lasers. Electron. Lett. 42, 89–91 (2006).
G. Scalari, C. Walther, J. Faist, H. Beere, and D. Ritchie, Electrically switchable, two-color quantum cascade laser emitting at 1.39 and 2.3 THz. Appl. Phys. Lett. 88, 141102 (2006).
C. Worrall, J. Alton, M. Houghton, S. Barbieri et al., Continuous wave operation of a superlattice quantum cascade laser emitting at 2 THz. Opt. Express 14, 171–181 (2006).
C. Walther, M. Fischer, G. Scalari, R. Terazzi et al., Quantum cascade lasers operating from 1.2 to 1.6 THz. Appl. Phys. Lett. 91, 131122 (2007).
A. Maestrini, J. Bruston, D. Pukala, and S. Martin, Performance of a 1.2 THz frequency tripler using a GaAs frameless membrane monolithic circuit. IEEE MTT-S Int. Microwave Symp. Dig. 3, 1657–1660 (2001).
A. Maestrini, J. Ward, J. Gill, H. Javadi et al., A 1.7-1.9 THz local oscillator source. IEEE Microw. Wirel. Compon. Lett. 14, 253–255 (2004).
G. Chattopadhyay, E. Schlecht, J.S. Ward, J. Gill et al., An all-solid-state broad-band frequency multiplier chain at 1500 GHz. IEEE Trans. MTT-52, 1538–1546 (2004).
J. Ward, E. Schlecht, G. Chattopadhyay, A. Maestrini et al., Capability of THz sources based on Schottky diode frequency multiplier chains. IEEE MTT-S Int. Microw. Symp. Dig. 3, 1587–1590 (2004).
Acknowledgments
The author wish to acknowledge financial support from the National Natural Science Foundation of China ( 60471017 & 60621002)
Author information
Authors and Affiliations
Corresponding author
Rights and permissions
About this article
Cite this article
Wu, T. Diamond Schottky Contact Transit-time Diode for Terahertz Power Generation. Int J Infrared Milli Waves 29, 634–640 (2008). https://doi.org/10.1007/s10762-008-9363-8
Received:
Accepted:
Published:
Issue Date:
DOI: https://doi.org/10.1007/s10762-008-9363-8