Abstract
Context
Using transition state theory, acetone sensing by pristine and rhodium-doped tin dioxide is discussed. The Evans–Polanyi principle is modified from its original formulation commensurate with the Arrhenius equation to be more suitable for transition state theory. The new formalism for the activation energy replaces enthalpy with Gibbs free energy in the original Evans–Polanyi principle. The new formalism considers reaction entropy, which is not considered previously in Evans–Polanyi principle. Response and response time of interaction of acetone with both pristine and Rh-doped SnO2 clusters is calculated. Variations of response in terms of acetone concentration and temperature are calculated and compared to the experiment. Acceptable agreement between theory and experiment that calls for more comparisons to demonstrate the modified approach.
Methods
The pristine and Rh-doped clusters and their interaction with acetone are simulated using density functional theory at the B3LYP level. 6-311G** and SDD (for heavy atoms) basis sets are used to optimize the structures examined in the present work. Gaussian 09 program and accompanying software performed the current tasks.









Similar content being viewed by others
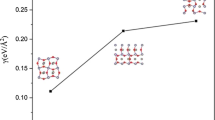
Data Availability
Data are available at request.
References
Liew FE, Nogle R, Abdalla T, Rasor BJ, Canter C, Jensen RO, Wang L, Strutz J, Chirania P, De Tissera S, Jewett MC, Köpke M (2022) Carbon-negative production of acetone and isopropanol by gas fermentation at industrial pilot scale. Nat Biotechnol 40:335–344. https://doi.org/10.1038/s41587-021-01195-w
Ujor V, Bharathidasan AK, Cornish K, Ezeji TC (2014) Evaluation of industrial dairy waste (milk dust powder) for acetone-butanol-ethanol production by solventogenic Clostridium species. Springerplus 3:1–10. https://doi.org/10.1186/2193-1801-3-387
Li G, Cheng Z, Xiang Q, Yan L, Wang X, Xu J (2019) Bimetal PdAu decorated SnO2 nanosheets based gas sensor with temperature-dependent dual selectivity for detecting formaldehyde and acetone. Sensors Actuators B: Chem 283:590–601. https://doi.org/10.1016/j.snb.2018.09.117
Li C, Choi PG, Masuda Y (2023) Large-lateral-area SnO2 nanosheets with a loose structure for high-performance acetone sensor at the ppt level. J Hazard Mater 455:131592. https://doi.org/10.1016/j.jhazmat.2023.131592
Abdulsattar MA, Mahmood TH (2023) Enhancement of SnO2 sensitivity to acetone by Au loading: An application of Evans-Polanyi principle in gas sensing. Optik 275:170604. https://doi.org/10.1016/j.ijleo.2023.170604
Lokhande SD, Awale MB, Umadevi G, Mote VD (2023) Effect of Ni doping on structural, optical and gas sensing properties of ZnO films for the development of acetone sensor devices. Mater Chem Phys 301:127667. https://doi.org/10.1016/j.matchemphys.2023.127667
Dang TK, Cuong ND, Van Minh Hai H, Phuong TQ, Son LL, Thi Thanh Nhan D, Van Tan V, Hien MD, Jeon KJ, Hung NQ, Tuyen LA, Van Hieu N (2023) Exploring novel p-n core/shell structure in single α-Fe2O3 nanorods of hierarchical hollow microspheres for ultrasensitive acetone gas sensor. Sensors Actuators B: Chem 383. https://doi.org/10.1016/j.snb.2023.133573
Li C, Kim K, Fuchigami T, Asaka T, ichi Kakimoto K, Masuda Y (2023) Acetone gas sensor based on Nb2O5 @SnO2 hybrid structure with high selectivity and ppt-level sensitivity. Sensors Actuators B: Chem 393. https://doi.org/10.1016/j.snb.2023.134144
Han B, Xia F, wen Zhang Z, Wang M, nan Zhang Y (2023) Acetone gas sensor based on PVA self-assembled WGM microbottle resonator coated with PDMS. Opt Fiber Technol 80. https://doi.org/10.1016/j.yofte.2023.103383
Roduner E (2014) Understanding catalysis. Chem Soc Rev 43:8226–8239. https://doi.org/10.1039/c4cs00210e
Fajín JLC, Viñes F, Cordeiro MNDS, Illas F, Gomes JRB (2016) Effect of the exchange-correlation potential on the transferability of Brønsted-Evans-Polanyi relationships in heterogeneous catalysis. J Chem Theory Comput 12:2121–2126. https://doi.org/10.1021/acs.jctc.6b00168
Abdulsattar MA, Abduljalil HM, Abed HH (2023) Reaction mechanisms of pristine and Cu-doped ZnO clusters with ethanol using Evans-Polanyi principle. Emerging Mater Res 12:60–67. https://doi.org/10.1680/jemmr.22.00149
Kou X, Meng F, Chen K, Wang T, Sun P, Liu F, Yan X, Sun Y, Liu F, Shimanoe K, Lu G (2020) High-performance acetone gas sensor based on Ru-doped SnO2 nanofibers. Sensors Actuators B: Chem 320. https://doi.org/10.1016/j.snb.2020.128292
Li J, Xian J, Wang W, Cheng K, Zeng M, Zhang A, Wu S, Gao X, Lu X, Liu JM (2022) Ultrafast response and high-sensitivity acetone gas sensor based on porous hollow Ru-doped SnO2 nanotubes. Sensors Actuators B: Chem 352. https://doi.org/10.1016/j.snb.2021.131061
Cui S, Qin J, Liu W (2023) Ultrafine Pt-doped SnO2 mesopore nanofibers-based gas sensor for enhanced acetone sensing. Chin J Anal Chem 51. https://doi.org/10.1016/j.cjac.2022.100188
Kou X, Xie N, Chen F, Wang T, Guo L, Wang C, Wang Q, Ma J, Sun Y, Zhang H, Lu G (2018) Superior acetone gas sensor based on electrospun SnO2 nanofibers by Rh doping. Sensors Actuators B: Chem 256:861–869. https://doi.org/10.1016/j.snb.2017.10.011
Arshadi S, Abdolahzadeh F, Vessally E (2023) Butadiyne-linked porphyrin nanoring as a highly selective O2 gas sensor: A fast response hybrid sensor. J Mol Graph Model 119:108371. https://doi.org/10.1016/j.jmgm.2022.108371
Frisch MJ, Trucks GW, Schlegel HB, Scuseria GE, Robb MA, Cheeseman JR, Scalmani G, Barone V, Mennucci B, Petersson GA, Nakatsuji H, Caricato M, Li X, Hratchian HP, Izmaylov AF, Bloino J, Zheng G, Sonnenberg JL, Hada M, Ehara M, Toyota K, Fukuda R, Hasegawa J, Ishida M, Nakajima T, Honda Y, Kitao O, Nakai H, Vreven T, Montgomery JAJ, Peralta JE, Ogliaro F, Bearpark M, Heyd JJ, Brothers E, Kudin KN, Staroverov VN, Kobayashi R, Normand J, Raghavachari K, Rendell A, Burant JC, Iyengar SS, Tomasi J, Cossi M, Rega N, Millam JM, Klene M, Knox JE, Cross JB, Bakken V, Adamo C, Jaramillo J, Gomperts R, Stratmann RE, Yazyev O, Austin AJ, Cammi R, Pomelli C, Ochterski JW, Martin RL, Morokuma K, Zakrzewski VG, Voth GA, Salvador P, Dannenberg JJ, Dapprich S, Daniels AD, Farkas Ö, Foresman JB, Ortiz JV, Cioslowski J, Fox DJ (2013) Gaussian 09, Revision D.01
Zhao Z, Li Z (2020) First-principle calculations on the structures and electronic properties of the CO-adsorbed (SnO2)2 clusters. Struct Chem 31:1861–1867. https://doi.org/10.1007/s11224-020-01554-4
Chen W, Zhou Q, Wan F, Gao T (2012) Gas sensing properties and mechanism of Nano-SnO2-based sensor for hydrogen and carbon monoxide. J Nanomater 2012. https://doi.org/10.1155/2012/612420
Abdulsattar MA, Nassrullah DA, Abdulhamied ZT (2019) Light alkanes physisorption and chemisorption on SnO2 pyramid clusters surface as a function of temperature: A DFT study. J Adv Pharm Educ Res 9:118–124
Shi XH, Xu KJ (2017) Properties of fluorine-doped tin oxide films prepared by an improved sol-gel process. Mater Sci Semicond Process 58:1–7. https://doi.org/10.1016/j.mssp.2016.09.038
Abdulsattar MA (2020) Transition state theory application to H2 gas sensitivity of pristine and Pd doped SnO2 clusters. Karbala Int J Mod Sci 6:13. https://doi.org/10.33640/2405-609X.1615
Song BY, Li C, Lv MS, Zhang XF, Deng ZP, Xu YM, Huo LH, Gao S (2023) Biotemplate-inherited porous SnO2 monotubes with oxygen vacancies for ultra-high response and fast detection of nitric oxide at low temperature. Sensors Actuators B: Chem 387:133811. https://doi.org/10.1016/j.snb.2023.133811
Zhang Y, Yang Z, Zhao L, Xing Y, Xin C, Wei Z, Fei T, Liu S, Zhang T (2023) The synergy of Pd nanoparticles and oxygen vacancy to modulate SnO2 modified reduced graphene oxide hybrids for room-temperature ppb-level NO2 detection. Appl Surf Sci 624:157146. https://doi.org/10.1016/j.apsusc.2023.157146
Abdulsattar MA, Mahmood TH, Abed HH, Abduljalil HM (2023) Adsorption and desorption of acetone by TiO2 clusters: Transition state theory and sensing analysis. ChemPhysMater. https://doi.org/10.1016/j.chphma.2023.05.001
Rufino M, Guedes S (2022) Arrhenius activation energy and transitivity in fission-track annealing equations. Chem Geol 595. https://doi.org/10.1016/j.chemgeo.2022.120779
Chen Y, Chang K-H, Meng F-Y, Tseng S-M, Chou P-T (2021) Broadening the horizon of the Bell–Evans–Polanyi principle towards optically triggered structure planarization. Angew Chem Int Ed 60:7205–7212. https://doi.org/10.1002/anie.202015274
Chang T-Y, Wang Z, Xu D (2023) Formation energetics/dynamics of icosahedral clusters in supercooled metallic liquids in the dynamic equilibrium regime: Gibbs free energy, entropy, enthalpy, and connection to coordination shells. J Mater Res 38:179–186. https://doi.org/10.1557/s43578-022-00664-5
Filonenko OV, Grebenyuk AG, Lobanov VV (2021) Quantum chemical modeling of the structure and properties of SnO2 nanoclusters. Him Fiz Tehnol Poverhni 12:283–290. https://doi.org/10.15407/HFTP12.04.283
Kohout J (2021) Modified Arrhenius equation in materials science, chemistry and biology. Molecules 26:7162. https://doi.org/10.3390/molecules26237162
Du R-L, Wu K, Xu D-A, Chao C-Y, Zhang L, Du X-D (2016) A modified Arrhenius equation to predict the reaction rate constant of Anyuan pulverized-coal pyrolysis at different heating rates. Fuel Process Technol 148:295–301. https://doi.org/10.1016/j.fuproc.2016.03.011
Yin XT, Tao L (2017) Fabrication and gas sensing properties of Au-loaded SnO2 composite nanoparticles for low concentration hydrogen. J Alloy Compd 727:254–259. https://doi.org/10.1016/j.jallcom.2017.08.122
Lide DR (ed) (2008) CRC handbook of chemistry and physics. CRC Press/Taylor and Francis, Boca Raton
Guo L, Shen Z, Ma C, Ma C, Wang J, Yuan T (2022) Gas sensor based on MOFs-derived Au-loaded SnO2 nanosheets for enhanced acetone detection. J Alloys Compd 906. https://doi.org/10.1016/j.jallcom.2022.164375
Koffyberg FP (1992) Optical bandgaps and electron affinities of semiconducting Rh2O3(I) and Rh2O3(III). J Phys Chem Solids 53:1285–1288. https://doi.org/10.1016/0022-3697(92)90247-B
Author information
Authors and Affiliations
Contributions
All research activities are performed by Mudar Ahmed Abdulsattar.
Corresponding author
Ethics declarations
Competing interests
The authors declare no competing interests.
Additional information
Publisher's Note
Springer Nature remains neutral with regard to jurisdictional claims in published maps and institutional affiliations.
Rights and permissions
Springer Nature or its licensor (e.g. a society or other partner) holds exclusive rights to this article under a publishing agreement with the author(s) or other rightsholder(s); author self-archiving of the accepted manuscript version of this article is solely governed by the terms of such publishing agreement and applicable law.
About this article
Cite this article
Abdulsattar, M.A. The reaction of pristine and Rh-doped SnO2 clusters with acetone: Application of Evans–Polanyi principle to transition state theory. J Mol Model 29, 304 (2023). https://doi.org/10.1007/s00894-023-05710-5
Received:
Accepted:
Published:
DOI: https://doi.org/10.1007/s00894-023-05710-5