Abstract
The objective of this study is to describe the oxygen profile obtained by electron paramagnetic resonance (EPR) oximetry of tissue after radiation, surgery, and hyperbaric oxygen therapy (HBOT) and its relationship to wound healing in a rodent model. The study design is rodent model for wound healing. A rodent model for wound healing was used for oxygen measurements before and after various treatments. EPR measurements and biopsies of normal vs irradiated and flap vs non-flap tissues were taken at 1–3-week intervals for 12 weeks. Wound healing was evaluated by gross photos, histology, and immunostaining. Student’s t test and a linear mixed model were used to compare oxygen levels and gross healing with radiation exposure. A Proportional Odds model was also used to calculate odds ratio toward better wound-healing rate with radiation exposure. In the rodent model, at 1–3 weeks after irradiation, the mean tissue oxygen measurement was significantly lower in irradiated versus non-irradiated leg tissue. There was a significant difference in oxygenation between flap and non-flap tissue in an irradiated bed at 1 and 3 weeks after surgery. On gross evaluation, wound healing from z-plasty flap was significantly worse in irradiated tissue compared to non-irradiated tissue. A rodent model for wound healing showed that radiation resulted in decreased tissue oxygenation at 1–3 weeks after irradiation. Wound healing was compromised in irradiated tissue at earlier time points when tissue oxygenation was lower. Oxygen profiling with EPR oximetry can be used to identify timing of oxygen interventions to improve wound healing. Level of evidence is NA, animal studies.









Similar content being viewed by others
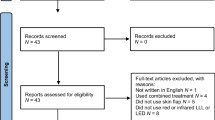
Availability of Data and Materials
The data supporting the results reported in the article are available upon request.
References
J.M. Arbeit, J.M. Brown, K.S.C. Chao et al., Hypoxia: importance in tumor biology, noninvasive measurement by imaging, and value of its measurement in the management of cancer therapy. Int. J. Radiat. Biol. 82, 699–757 (2006). https://doi.org/10.1080/09553000601002324
H.W. Hopf, M.D. Rollins, Wounds: an overview of the role of oxygen. Antioxid. Redox Signal. 9, 1183–1192 (2007)
C.K. Sen, Wound healing essentials: let there be oxygen. Wound Repair Regen. 17, 1–18 (2009). https://doi.org/10.1111/j.1524-475X.2008.00436.x
S. Barrientos, O. Stojadinovic, M.S. Golinko et al., Growth factors and cytokines in wound healing. Wound Repair Regen. 16, 585–601 (2008). https://doi.org/10.1111/j.1524-475X.2008.00410.x
G.M. Gordillo, S. Roy, S. Khanna et al., Topical oxygen therapy induces vascular endothelial growth factor expression and improves closure of clinically presented chronic wounds. Clin. Exp. Pharmacol. Physiol. 35, 957–964 (2008). https://doi.org/10.1111/j.1440-1681.2008.04934.x
R.B. Fries, W.A. Wallace, S. Roy et al., Dermal excisional wound healing in pigs following treatment with topically applied pure oxygen. Mutat. Res. Fundam. Mol. Mech. Mutagen. 579, 172–181 (2005). https://doi.org/10.1016/j.mrfmmm.2005.02.023
P. Kranke, M.H. Bennett, M. Martyn-St James et al., Hyperbaric Oxygen Therapy for Chronic Wounds (John Wiley and Sons Ltd., 2015)
R. Choudhury, Hypoxia and hyperbaric oxygen therapy: a review. Int. J. Gen. Med. 11, 431–442 (2018). https://doi.org/10.2147/IJGM.S172460
S.H. Ko, A.C. Nauta, S.D. Morrison et al., PHD-2 suppression in mesenchymal stromal cells enhances wound healing. Plast. Reconstr. Surg. 141, 55e–67e (2018). https://doi.org/10.1097/PRS.0000000000003959
M.K. Tibbs, Wound healing following radiation therapy: a review. Radiother. Oncol. 42, 99–106 (1997)
S. Delanian, J.L. Lefaix, Current management for late normal tissue injury: radiation-induced fibrosis and necrosis. Semin. Radiat. Oncol. 17, 99–107 (2007). https://doi.org/10.1016/j.semradonc.2006.11.006
M.S. Anscher, L. Chen, Z. Rabbani et al., Recent progress in defining mechanisms and potential targets for prevention of normal tissue injury after radiation therapy. Int. J. Radiat. Oncol. Biol. Phys. 62, 255–259 (2005). https://doi.org/10.1016/j.ijrobp.2005.01.040
H.B. Stone, C.N. Coleman, M.S. Anscher, W.H. McBride, Effects of radiation on normal tissue: consequences and mechanisms. Lancet Oncol. 4, 529–536 (2003). https://doi.org/10.1016/S1470-2045(03)01191-4
H.B. Stone, W.H. McBride, C.N. Coleman, Modifying normal tissue damage postirradiation. Report of a workshop sponsored by the Radiation Research Program, National Cancer Institute. Radiat. Res. 157, 204–223 (2002). https://doi.org/10.1667/0033-7587(2002)157[0204:MNTDP]2.0.CO;2
C.B. Westbury, A. Pearson, A. Nerurkar et al., Hypoxia can be detected in irradiated normal human tissue: a study using the hypoxic marker pimonidazole hydrochloride. Br. J. Radiol. 80, 934–938 (2007). https://doi.org/10.1259/bjr/25046649
C.E. Fife, D.R. Smart, P.J. Sheffield et al., Transcutaneous oximetry in clinical practice: consensus statements from an expert panel based on evidence. Undersea Hyperb. Med. 36, 43–53 (2009)
A.B. Flood, V.A. Wood, H.M. Swartz, Using India ink as a sensor for oximetry: evidence of its safety as a medical device. Adv. Exp. Med. Biol. 977, 297–312 (2017). https://doi.org/10.1007/978-3-319-55231-6_40
N. Khan, H. Hou, H.M. Swartz, P. Kuppusamy, Direct and Repeated Measurement of Heart and Brain Oxygenation Using in Vivo EPR Oximetry. In: Methods in Enzymology. Academic Press Inc. 564, 529–552 (2015)
H. Hou, R. Dong, J.P. Lariviere et al., Synergistic combination of hyperoxygenation and radiotherapy by repeated assessments of tumor pO2 with EPR oximetry. J. Radiat. Res. 52, 568–574 (2011)
M.A. Polacco, H. Hou, P. Kuppusamy, E.Y. Chen, Measuring flap oxygen using electron paramagnetic resonance oximetry. Laryngoscope 129, E415–E419 (2019). https://doi.org/10.1002/lary.28043
C.M. Desmet, A. Lafosse, S. Vériter et al., Application of electron paramagnetic resonance (EPR) oximetry to monitor oxygen in wounds in diabetic models. PLoS ONE 10, 144914 (2015). https://doi.org/10.1371/journal.pone.0144914
C.M. Desmet, G. Vandermeulen, C. Bouzin et al., EPR monitoring of wound oxygenation as a biomarker of response to gene therapy encoding hCAP-18/LL37 peptide. Magn. Reson. Med. 79, 3267–3273 (2018)
M.M. Kmiec, H. Hou, M. Lakshmi Kuppusamy et al., Transcutaneous oxygen measurement in humans using a paramagnetic skin adhesive film. Magn. Reson. Med. 81, 781–794 (2019). https://doi.org/10.1002/mrm.27445
B.F. Jordan, C. Baudelet, B. Gallez, Carbon-centered radicals as oxygen sensors for in vivo electron paramagnetic resonance: screening for an optimal probe among commercially available charcoals. Magn. Reson. Mater. Phys. Biol. Med. 7, 121–129 (1998)
O.Y. Grinberg, H. Hou, S.A. Grinberg et al., pO2 and regional blood flow in a rabbit model of limb ischemia. Physiol. Meas. 25, 659–670 (2004). https://doi.org/10.1088/0967-3334/25/3/006
G.A. Salam, J.P. Amin, T.J. Zuber, The basic Z-plasty. Am. Fam. Physician 67, 2329–2332 (2003)
M.S. Fazeli, M.G. Adel, A.H. Lebaschi, Comparison of outcomes in Z-plasty and delayed healing by secondary intention of the wound after excision of the sacral pilonidal sinus: results of a randomized, clinical trial. Dis. Colon Rectum 49, 1831–1836 (2006). https://doi.org/10.1007/s10350-006-0726-8
N. Khan, B.B. Williams, H. Hou et al., Repetitive tissue pO2 measurements by electron paramagnetic resonance oximetry: current status and future potential for experimental and clinical studies. Antioxid. Redox Signal. 9, 1169–1182 (2007). https://doi.org/10.1089/ars.2007.1635
N. Khan, S.P. Mupparaju, D. Mintzopoulos et al., Burn trauma in skeletal muscle results in oxidative stress as assessed by in vivo electron paramagnetic resonance. Mol. Med. Rep. 1, 813–819 (2008). https://doi.org/10.3892/mmr-00000033
J.F. Dunn, H.M. Swartz, In vivo electron paramagnetic resonance oximetry with particulate materials. Methods 30, 159–166 (2003). https://doi.org/10.1016/S1046-2023(03)00077-X
H.M. Swartz, H. Hou, N. Khan et al., Advances in probes and methods for clinical EPR oximetry. Adv. Exp. Med. Biol. 812, 73–79 (2014). https://doi.org/10.1007/978-1-4939-0620-8_10
E. Demidenko, Mixed Models: Theory and Applications with R, 2nd edn. (Wiley, Hoboken, 2013)
D.M. Ansell, K.A. Holden, M.J. Hardman, Animal models of wound repair: Are they cutting it? Exp. Dermatol. 21, 581–585 (2012). https://doi.org/10.1111/j.1600-0625.2012.01540.x
Z. Vujaskovic, M.S. Anscher, Q.F. Feng et al., Radiation-induced hypoxia may perpetuate late normal tissue injury. Int. J. Radiat. Oncol. Biol. Phys. 50, 851–855 (2001). https://doi.org/10.1016/S0360-3016(01)01593-0
S. Auerswald, S. Schreml, R. Meier et al., Wound monitoring of pH and oxygen in patients after radiation therapy. Radiat. Oncol. (2019). https://doi.org/10.1186/s13014-019-1413-y
M.S. Chin, B.B. Freniere, C.F. Bonney et al., Skin perfusion and oxygenation changes in radiation fibrosis. Plast. Reconstr. Surg. 131, 707–716 (2013). https://doi.org/10.1097/PRS.0b013e3182818b94
J. Wright, Hyperbaric oxygen therapy for wound healing (2001). In: http://www.worldwidewounds.com/2001/april/Wright/HyperbaricOxygen.html. Accessed 14 Feb 2021
S. Gehmert, S. Geis, P. Lamby et al., Evaluation of hyperbaric oxygen therapy for free flaps using planar optical oxygen sensors. Preliminary results. Clin. Hemorheol. Microcirc. 48, 75–79 (2011). https://doi.org/10.3233/CH-2011-1389
J. Tlapák, P. Chmátal, J. Pejchal et al., The effect of hyperbaric oxygen therapy on acute wound healing in rabbits: an experimental stude and histopathological analysis. Mil Med Sci Lett 90, 2 (2021). https://doi.org/10.31482/mmsl.2021.001
M.S. Anscher, B. Thrasher, Z. Rabbani et al., Antitransforming growth factor-β antibody 1D11 ameliorates normal tissue damage caused by high-dose radiation. Int. J. Radiat. Oncol. Biol. Phys. 65, 876–881 (2006). https://doi.org/10.1016/j.ijrobp.2006.02.051
M.S. Anscher, B. Thrasher, L. Zgonjanin et al., Small molecular inhibitor of transforming growth factor-β protects against development of radiation-induced lung injury. Int. J. Radiat. Oncol. Biol. Phys. 71, 829–837 (2008). https://doi.org/10.1016/j.ijrobp.2008.02.046
Y. Liu, K. Kudo, Y. Abe et al., Inhibition of transforming growth factor-beta, hypoxia-inducible factor-1alpha and vascular endothelial growth factor reduced late rectal injury induced by irradiation. J. Radiat. Res. 50, 233–239 (2009)
J.W. Lee, J.P. Tutela, R.A. Zoumalan et al., Inhibition of Smad3 expression in radiation-induced fibrosis using a novel method for topical transcutaneous gene therapy. Arch. Otolaryngol. Head Neck Surg. 136, 714–719 (2010). https://doi.org/10.1001/archoto.2010.107
C.A. Reddy, V. Somepalli, T. Golakoti et al., Mitochondrial-targeted curcuminoids: a strategy to enhance bioavailability and anticancer efficacy of curcumin. PLoS ONE 9, 1–11 (2014). https://doi.org/10.1371/journal.pone.0089351
N. Charlier, N. Beghein, B. Gallez, Development and evaluation of biocompatible inks for the local measurement of oxygen using in vivo EPR. NMR Biomed. 17, 303–310 (2004). https://doi.org/10.1002/nbm.902
G. Meenakshisundaram, E. Eteshola, R.P. Pandian et al., Fabrication and physical evaluation of a polymer-encapsulated paramagnetic probe for biomedical oximetry. Biomed. Microdevices 11, 773–782 (2009). https://doi.org/10.1007/s10544-009-9292-x
P.E. Schaner, J.R. Pettus, A.B. Flood et al., OxyChip implantation and subsequent electron paramagnetic resonance oximetry in human tumors is safe and feasible: first experience in 24 patients. Front. Oncol. (2020). https://doi.org/10.3389/fonc.2020.572060
Acknowledgements
We acknowledge George Zhongzi Li for his consultation and assistance with the biostatistical analyses. We also acknowledge Lesley Jarvis, Jack Hoopes, Andrew Giustini, and Rendy Strawbridge for their assistance with the rat model and radiation treatments. We thank Thomas Matthews, Kevin Rychert, Huagang Hou, Nadeem Khan, Oleg Grinberg, and Hal Swartz for their assistance with EPR measurements and analyses as well as Jay Buckey, Donna Alvarenga, Hermine Wallach, and Nancy Yazinski for their help with the hyperbaric oxygen treatments.
Funding
These studies were supported by The Triological Society Career Development Award and pilot award from the Norris Cotton Cancer Center’s American Cancer Society Institutional Research Grant (IRG-82-003-27).
Author information
Authors and Affiliations
Contributions
EYC and BBW contributed to the study conception and design. Material preparation and data collection were performed by EYC and SH. Analyses were performed by all authors. The first draft of the manuscript was written by EYC and all authors commented on previous versions of the manuscript. All authors read and approved the final manuscript.
Corresponding author
Ethics declarations
Conflict of interest
The authors do not have any conflicts of interest or competing interests to disclose.
Additional information
Publisher's Note
Springer Nature remains neutral with regard to jurisdictional claims in published maps and institutional affiliations.
Supplementary Information
Below is the link to the electronic supplementary material.
723_2021_1367_MOESM1_ESM.pdf
Supplementary Timeline 1. Schedule of char implantations, EPR measurements, treatments, and procedures in rat model for studies excluding (A) and including (B) hyperbaric oxygen therapy. Key: EPR, electron paramagnetic resonance oximetry; XRT, radiation therapy; HBOT, hyperbaric oxygen therapy; W, week (PDF 256 KB)
Rights and permissions
About this article
Cite this article
Chen, E.Y., Hodge, S. & Williams, B.B. Tissue Oxygen Profiling Using Electron Paramagnetic Resonance Oximetry to Improve Wound Healing After Radiation. Appl Magn Reson 52, 1489–1507 (2021). https://doi.org/10.1007/s00723-021-01367-6
Received:
Revised:
Accepted:
Published:
Issue Date:
DOI: https://doi.org/10.1007/s00723-021-01367-6