Abstract
In this study the performance of an indirect evaporative cooling system (IECS) of cross-flow configuration is numerically investigated. Considering the variation of water film temperature along the flowing path and the wettability of the wet channel, a two-dimensional theoretical model is developed to comprehensively describe the heat and mass transfer process involved in the system. After comparing the simulation results with available experimental data from literature, the deviation within ±5 % proves the accuracy and reliability of the proposed mathematical model. The simulation results of the plate type IECS indicate that the important parameters, such as dimension of plates, air properties, and surface wettability play a great effect on the cooling performance. The investigation of flow pattern shows that cross-flow configuration of primary air with counter-flow of secondary air and water film has a better cooling performance than that of the parallel-flow pattern. Furthermore, the performance of a novel flat tube working as the separating medium is numerically investigated. Simulation results for this novel geometry indicate that the tube number, tube long axis and short axis length as well as tube length remarkably affect its cooling performance.











Similar content being viewed by others
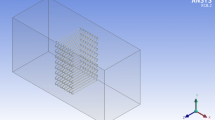
Abbreviations
- a :
-
Tube long axis length (m)
- b :
-
Tube short axis length (m)
- c p :
-
Specific heat capacity at constant pressure (J/kg K)
- dx :
-
Dimension of control element along x-axis (m)
- dy :
-
Dimension of control element along y-axis (m)
- D dh :
-
Degree of humidity (g/kg)
- D eq :
-
Equivalent diameter (m)
- f :
-
Friction factor
- h :
-
Convective heat transfer coefficient (W/m2 K)
- h d :
-
Convective mass transfer coefficient (kg/m2 s)
- g :
-
Acceleration due to gravity (m/s2)
- i :
-
Specific enthalpy (J/kg)
- i o :
-
Specific enthalpy of vapor at 0 °C (J/kg)
- k:
-
Thermal conductivity (W/m2 K)
- L x :
-
System length (m)
- L y :
-
System height (m)
- Le:
-
Lewis factor
- m :
-
Mass flow rate (kg/s)
- N :
-
Number of plates or tubes
- N t :
-
Number of tubes in one column
- N l :
-
Number of tubes in one row
- Nu :
-
Nusselt number
- Pr :
-
Prandtl number
- ΔP :
-
Pressure drop (Pa)
- Q :
-
Heat transfer rate (W)
- Re :
-
Reynolds number
- r :
-
Relative pitch
- S :
-
Tube spacing (m)
- T :
-
Temperature (°C)
- U :
-
Overall heat transfer coefficient (W/m2 K)
- ν :
-
Velocity (m/s)
- z :
-
Channel width (m)
- ρ :
-
Density (kg/m3)
- δ :
-
Thickness (m)
- σ :
-
Surface wetting factor
- μ :
-
Dynamic viscosity [kg/(m s)]
- ε :
-
Efficiency
- Γ:
-
Water linear flow rate [kg/(m s)]
- ω :
-
Humidity ratio (g/kg dry air)
- ϕ :
-
Relative humidity
- a :
-
Dry air
- c :
-
Calculated results
- e :
-
Experimental data
- i :
-
Inlet
- int :
-
Water and secondary air interface
- l :
-
Longitudinal
- lam :
-
Laminar flow
- o :
-
Outlet
- p :
-
Primary air
- ps :
-
Primary air to secondary air
- pw :
-
Primary air to water
- s :
-
Secondary air
- se :
-
Sensible
- la :
-
Latent heat
- m :
-
Moist air
- sat :
-
Saturated/saturation
- t :
-
Transversal
- tur :
-
Turbulent flow
- ν :
-
Vapor
- w :
-
Water film
- wb :
-
Wet bulb
- x :
-
x axis direction
- y :
-
y axis direction
References
Duan Z, Zhan C, Zhang X, Mustafa M, Zhao X, Alimohammadisagvand B et al (2012) Indirect evaporative cooling: past, present and future potentials. Renew Sustain Energy Rev 16:6823–6850
Chua KJ, Chou SK, Yang WM, Yan J (2013) Achieving better energy-efficient air conditioning—a review of technologies and strategies. Appl Energy 104:87–104
Maclaine-Cross IL, Banks PJ (1981) A general theory of wet surface heat exchangers and its application to regenerative evaporative cooling. J Heat Transf 103:579–585
Kettleborough CF, Hsieh CS (1983) The thermal performance of the wet surface plastic plate heat exchanger used as an indirect evaporative cooler. J Heat Transf 105:366–373
Erens P, Dreyer A (1993) Modelling of indirect evaporative air coolers. Int J Heat Mass Transf 36:17–26
Halasz B (1998) A general mathematical model of evaporative cooling devices. Revue Générale de Thermique 37:245–255
Tsay Y (1994) Analysis of heat and mass transfer in a countercurrent-flow wet surface heat exchanger. Int J Heat Fluid Flow 15:149–159
Hasan A (2012) Going below the wet-bulb temperature by indirect evaporative cooling: analysis using a modified ε-NTU method. Appl Energy 89:237–245
Ren C, Yang H (2006) An analytical model for the heat and mass transfer processes in indirect evaporative cooling with parallel/counter flow configurations. Int J Heat Mass Transf 49:617–627
Hsu S, Lavan Z, Worek W (1989) Optimization of wet-surface heat exchangers. Energy 14:757–770
Zhao X, Li JM, Riffat SB (2008) Numerical study of a novel counter-flow heat and mass exchanger for dew point evaporative cooling. Appl Therm Eng 28:1942–1951
Riangvilaikul B, Kumar S (2010) Numerical study of a novel dew point evaporative cooling system. Energy Build 42:2241–2250
Zhao X, Liu S, Riffat SB (2008) Comparative study of heat and mass exchanging materials for indirect evaporative cooling systems. Build Environ 43:1902–1911
Stoitchkov NJ, Dimitrov GI (1998) Effectiveness of crossflow plate heat exchanger for indirect evaporative cooling. Int J Refrig 21:463–471
Qureshi BA, Zubair SM (2007) Prediction of evaporation losses in evaporative fluid coolers. Appl Therm Eng 27:520–527
Kim NH, Lee EJ, Byun HW (2013) Condensation heat transfer and pressure drop in flattened smooth tubes having different aspect ratios. Exp Therm Fluid Sci 46:245–253
Kim N-H, Lee E-J, Byun H-W (2013) Evaporation heat transfer and pressure drop of R-410A in flattened smooth tubes having different aspect ratios. Int J Refrig 36:363–374
Tovaras NV, Bykov AV, Gogolin VA (1984) Heat exchange at film water flow under operating conditions of evaporative condenser. Holod Teh 1:25–29
Cengel Y, Boles M, Kanoğlu M (2011) Thermodynamics: an engineering approach. McGraw-Hill, New York
Kim T (2013) Effect of longitudinal pitch on convective heat transfer in crossflow over in-line tube banks. Ann Nucl Energy 57:209–215
Gaddis ES, Gnielinski V (1985) Pressure drop in cross flow across tube bundles. Int Chem Eng (United States) 25:1–15
Gómez EV, González AT, Martínez FR (2012) Experimental characterisation of an indirect evaporative cooling prototype in two operating modes. Appl Energy 97:340–346
Tejero-González A, Andrés-Chicote M, Velasco-Gómez E, Rey-Martínez FJ (2013) Influence of constructive parameters on the performance of two indirect evaporative cooler prototypes. Appl Therm Eng 51:1017–1025
Barzegar M, Layeghi M, Ebrahimi G, Hamzeh Y, Khorasani M (2012) Experimental evaluation of the performances of cellulosic pads made out of Kraft and NSSC corrugated papers as evaporative media. Energy Convers Manag 54:24–29
Dai YJ, Sumathy K (2002) Theoretical study on a cross-flow direct evaporative cooler using honeycomb paper as packing material. Appl Therm Eng 22:1417–1430
Acknowledgement
The authors gratefully acknowledge the kind support of National Research Foundation CRP Programme NRF2011NRF_CRP003_003 funding for this research.
Author information
Authors and Affiliations
Corresponding author
Rights and permissions
About this article
Cite this article
Chua, K.J., Xu, J., Cui, X. et al. Numerical heat and mass transfer analysis of a cross-flow indirect evaporative cooler with plates and flat tubes. Heat Mass Transfer 52, 1765–1777 (2016). https://doi.org/10.1007/s00231-015-1696-y
Received:
Accepted:
Published:
Issue Date:
DOI: https://doi.org/10.1007/s00231-015-1696-y