Abstract
Through-mask electrochemical machining is a promising technique for producing micro-grooves in difficult-to-process materials. In this study, we proposed manipulating the current density distribution in the processing area by optimizing the size and arrangement of elliptical through-holes in the mask, to tune the cross-sectional geometry of micro-grooves in an approximately semicircular shape, and to achieve stable processing of a dense multi-micro-grooves. A model was developed to investigate the dynamic forming process of micro-grooves. The effects of minor- and major-axis dimensions of the elliptical through-holes and the scanning speed of the integrated tool on the micro-groove profile were investigated by simulations and experiments. The experimental consequences point out that with the increases of major-axis length, the size of the groove is increased and the quality of the edge contour of the micro-grooves is improved. Approximate semicircular grooves with a radius of 50 μm were fabricated by optimizing parameters (voltage 30 V, scanning speed 50 μm/s, pulse duty cycle 10%) and using a mask of elliptical holes (major-axis length 230 μm, minor-axis length 75μm). To further improve the processing efficiency, the scanning speed can be increased to 100 μm/s when the pulse duty cycle is 20%. Finally, by optimizing the arrangement of elliptical holes in the insulation mask, dense multi-micro-grooves with a spacing of 320 μm and 160 μm were successfully fabricated on Ti6Al4V.
























Similar content being viewed by others
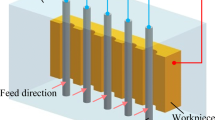
References
Wang GQ, Zhu D, Li HS (2018) Fabrication of semi-circular micro-groove on titanium alloy surface by through-mask electrochemical micromachining. J Mater Process Technol 258:22–28. https://doi.org/10.1016/j.jmatprotec.2018.03.015
Sasawat M, Necati CÖ, Muammer K (2010) Effect of manufacturing processes on formability and surface topography of proton exchange membrane fuel cell metallic bipolar plates. J Power Sources 195:5269–5277. https://doi.org/10.1016/j.jpowsour.2010.03.018
Sasawat M, Muammer K (2008) Fabrication of micro-channel arrays on thin metallic sheet using internal fluid pressure: investigations on size effects and development of design guidelines. J Power Sources 175:363–371. https://doi.org/10.1016/j.jpowsour.2007.09.036
Craig FR, Michael VJ (1996) Development of the micro-milling process for high aspect ratio microstructures. J. Microelectromech. Syst. 5:33–38
Li CZ, Xu ZT, Peng LF, Lai XM (2022) An electric-pulse-assisted stamping process towards springback suppression and precision fabrication of micro channels. Int J Mech Sci 218:107081. https://doi.org/10.1016/j.ijmecsci.2022.107081
Enrico S, Rachele B, Andrea G, Stefania B (2020) Mechanical and microstructural behaviour of AA7075 aluminium alloy for sub-zero temperature sheet stamping process. Int J Mech Sci 187:105919. https://doi.org/10.1016/j.ijmecsci.2020.105919
Priyabrata S, Karali P (2021) Cumulative reduction of friction and size effects in micro milling through proper selection of coating thickness of TiAlN coated tool: experimental and analytical assessments. J Manuf Process 67:635–654. https://doi.org/10.1016/j.jmapro.2021.05.037
Du YC, Liang ZQ, Ma Y, Su ZP, Chen R, Zhou TF, Wang XB (2022) Development of PCBN micro ball-end mill with multi-edge and spherical flank face. J Manuf Process 84:424–434. https://doi.org/10.1016/j.jmapro.2022.10.008
Han JJ, Ma R, Kong LL, Hao XQ, Chen N, Li L, He N (2022) Investigation on chip formation mechanism of high-aspect-ratio micro-milled structures. J Manuf Process 80:743–753. https://doi.org/10.1016/j.jmapro.2022.06.014
Sebastian G, Katja K, Benjamin K, Aurich JC (2021) Vibration-assisted micro milling of AISI 316L produced by laser-based powder bed fusion. J Manuf Process 71:298–305. https://doi.org/10.1016/j.jmapro.2021.09.020
Yan JW, Takuya K, Kazunori U, Nobuhito Y, Tsunemoto K (2010) Fabricating microgrooves with varied cross-sections by electrodischarge machining. Int J Adv Manuf Technol 50:991–1002. https://doi.org/10.1007/s00170-010-2563-0
Schreck S, Zum GK, H. (2005) Laser-assisted structuring of ceramic and steel surfaces for improving tribological properties. Appl Surf Sci 247:616–622. https://doi.org/10.1016/j.apsusc.2005.01.173
Wang CC, Chow HM, Yang LD, Lu CT (2009) Recast layer removal after electrical discharge machining via Taguchi analysis: a feasibility study. J Mater Process Technol 209:4134–4140. https://doi.org/10.1016/j.jmatprotec.2008.10.012
Murray JW, Clare AT (2012) Repair of EDM induced surface cracks by pulsed electron beam irradiation. J Mater Process Technol 212:2642–2651. https://doi.org/10.1016/j.jmatprotec.2012.07.018
Wu XY, Li SJ, Jia Z, Xin B, Yin XC (2019) Using WECM to remove the recast layer and reduce the surface roughness of WEDM surface. J Mater Process Technol 268:140–148. https://doi.org/10.1016/j.jmatprotec.2019.01.016
Murray J, Zdebski D, Clare AT (2012) Workpiece debris deposition on tool electrodes and secondary discharge phenomena in micro-EDM. J Mater Process Technol 212:1537–1547. https://doi.org/10.1016/j.jmatprotec.2012.02.019
Pauchard A., Meunier Michel, Lee K., Holmes Andrew S., Niino Hiroyuki, Vago N., Pavius M., Gu Bo, Obi S.2009., Advanced micromachining combining nanosecond lasers with water jet-guided laser technology, in: (Ed.)^(Eds.) Laser applications in microelectronic and optoelectronic manufacturing VII, , pp. 32-40.
Wang XS, Huang YK, Xu B, Xing YQ, Kang M (2019) Comparative assessment of picosecond laser induced plasma micromachining using still and flowing water. Opt Laser Technol 119:105623. https://doi.org/10.1016/j.optlastec.2019.105623
Wang XS, Huang YK, Wang XW, Xu B, Feng J, Shen B (2020) Experimental investigation and optimization of laser induced plasma micromachining using flowing water. Opt Laser Technol 126:106067. https://doi.org/10.1016/j.optlastec.2020.106067
Kumar SK, Qian J, Dominiek R (2018) A review on process capabilities of electrochemical micromachining and its hybrid variants. Int J Mach Tools Manuf 127:28–56. https://doi.org/10.1016/j.ijmachtools.2018.01.004
Bi XL, Zeng YB, Qu NS (2020) Wire electrochemical micromachining of high-quality pure-nickel microstructures focusing on different machining indicators. Precis Eng 61:14–22. https://doi.org/10.1016/j.precisioneng.2019.09.015
Liu Y, Qu NS (2020) Investigation on the performance of macro electrochemical machining of the end face of cylindrical parts. Int J Mech Sci 169:105333. https://doi.org/10.1016/j.ijmecsci.2019.105333
Wang YD, Xu ZY, Liu J, Zhang A, Xu ZL, Meng DM, Zhao JB (2021) Study on flow field of electrochemical machining for large size blade. Int J Mech Sci 190:106018. https://doi.org/10.1016/j.ijmecsci.2020.106018
Mitchell-Smith J, Speidel A, Clare AT (2018) Transitory electrochemical masking for precision jet processing techniques. J Manuf Process 31:273–285. https://doi.org/10.1016/j.jmapro.2017.11.028
Wang JK, Wataru N (2022) Mechanism and characteristics of electrochemical machining using electrolyte absorbed in solid porous ball. Precis Eng 77:307–319. https://doi.org/10.1016/j.precisioneng.2022.06.012
Hu XY, Zhu D, Li JB, Gu ZZ (2019) Flow field research on electrochemical machining with gas film insulation. J Mater Process Technol 267:247–256. https://doi.org/10.1016/j.jmatprotec.2018.12.019
Mitchell-Smith J, Clare AT (2016) Electrochemical jet machining of titanium: overcoming passivation layers with ultrasonic assistance. Procedia CIRP 42:379–383. https://doi.org/10.1016/j.procir.2016.02.215
Zhao YH, Masanori K (2019) Investigation on electrolyte jet machining of three-dimensional freeform surfaces. Precis Eng 60:42–53. https://doi.org/10.1016/j.precisioneng.2019.06.009
Matthias H-O, André M, Gunnar M, Mike Z, Andreas S (2013) Microstructuring of carbide metals applying jet electrochemical machining. Precis Eng 37:621–634. https://doi.org/10.1016/j.precisioneng.2013.01.007
Xue JB, Dong BY, Zhao YH (2022) Significance of waveform design to achieve bipolar electrochemical jet machining of passivating material via regulation of electrode reaction kinetics. Int J Mach Tools Manuf 177:103886. https://doi.org/10.1016/j.ijmachtools.2022.103886
Matthias H-O, Raphael P, André M, Gunnar M, Norbert L, Andreas S (2015) Study on the dynamic generation of the jet shape in jet electrochemical machining. J Mater Process Technol 223:240–251. https://doi.org/10.1016/j.jmatprotec.2015.03.049
Klocke F, Herrig T, Zeis M, Klink A (2018) Experimental investigations of cutting rates and surface integrity in wire electrochemical machining with rotating electrode. Procedia CIRP 68:725–730. https://doi.org/10.1016/j.procir.2017.12.145
Han W, Masanori K (2021) Research of micro EDM/ECM method in same electrolyte with running wire tool electrode. Precis Eng 70:1–14. https://doi.org/10.1016/j.precisioneng.2021.01.013
Han W, Masanori K (2018) Wire electrochemical grinding of tungsten micro-rod with electrostatic induction feeding method. Procedia CIRP 68:699–703. https://doi.org/10.1016/j.procir.2017.12.140
Vyom S, Singh PD, Jain VK, Ramkumar J (2020) Wire electrochemical micromachining: an overview. Int J Mach Tools Manuf 155:103579. https://doi.org/10.1016/j.ijmachtools.2020.103579
Wang GQ, Li HS, Qu NS, Zhu D (2016) Investigation of the hole-formation process during double-sided through-mask electrochemical machining. J Mater Process Technol 234:95–101. https://doi.org/10.1016/j.jmatprotec.2016.01.010
Wataru N, Tomone I, Masanori K (2007) Generating complicated surface with electrolyte jet machining. Precis Eng 31:33–39. https://doi.org/10.1016/j.precisioneng.2006.02.004
Matthias H-O, Gunnar M, Mike Z, André M, Andreas S (2012) Micro machining with continuous electrolytic free jet. Precis Eng 36:612–619. https://doi.org/10.1016/j.precisioneng.2012.05.003
Jonathon M-S, Alistair S, Jennifer G, Clare AT (2017) Energy distribution modulation by mechanical design for electrochemical jet processing techniques. Int J Mach Tools Manuf 122:32–46. https://doi.org/10.1016/j.ijmachtools.2017.05.005
Luo JX, Fang XL, Zhu D (2020) Jet electrochemical machining of multi-grooves by using tube electrodes in a row. J Mater Process Technol 283:116705. https://doi.org/10.1016/j.jmatprotec.2020.116705
Li TY, Yan X, Fang XL, Jin PH, Li JQ, Fazle RK, Nenad M (2021) In situ jet electrolyte micromachining and additive manufacturing. Appl Phys Lett 119:171602. https://doi.org/10.1063/5.0067988
Shik SH, Hyun KB, Nam CC (2008) Analysis of the side gap resulting from micro electrochemical machining with a tungsten wire and ultrashort voltage pulses. J Micromech Microeng 18:075009. https://doi.org/10.1088/0960-1317/18/7/075009
Wang XY, Fang XL, Zeng YB, Qu NS (2016) Fabrication of micro annular grooves on a cylindrical surface in aluminum alloys by wire electrochemical micromachining. Int J Electrochem Sci 11:7216–7229. https://doi.org/10.20964/2016.08.62
Zhou ZQ, Fang XL, Zeng YB, Zhu D (2020) Generating micro grooves with a semicircular cross-section using wire electrochemical micromachining. Int J Adv Manuf Technol 110:2929–2940. https://doi.org/10.1007/s00170-020-05934-2
Zhou X, Qu NS, Hou ZB, Zhao G (2018) Electrochemical micromachining of microgroove arrays on phosphor bronze surface for improving the tribological performance. Chin J Aeronaut 31:1609–1618. https://doi.org/10.1016/j.cja.2017.12.007
Chen XL, Dong BY, Zhang CY, Luo HP, Liu JW, Zhang YJ, Guo ZN (2019) Electrochemical direct-writing machining of micro-channel array. J Mater Process Technol 265:138–149. https://doi.org/10.1016/j.jmatprotec.2018.10.014
Chen XL, Dong BY, Fan GC, Zhang CY, Liu JW, Zhang YJ, Guo ZN (2019) Investigation on modified jet electrochemical machining of micro-channel. Int J Adv Manuf Technol 104:4433–4443. https://doi.org/10.1007/s00170-019-04312-x
Funding
This project was supported by the Natural Science Foundation of Jiangsu Province (Grant No. BK2022010), the Joint Funds of the National Natural Science Foundation of China and Guangdong Province (Grant No. U1601201), and the National Natural Science Foundation of China for Creative Research Groups (Grant No. 51921003).
Author information
Authors and Affiliations
Contributions
Guodong Miao: conceptualization, methodology, formal analysis, investigation, writing - review and editing. Shuai Ao: investigation, data curation, validation. Xiaolei Chen: methodology. Xiaolong Fang: funding acquisition, resources, review and editing. Di Zhu: resources, review and editing.
Corresponding author
Ethics declarations
Ethics approval
Not applicable
Consent to participate
Not applicable
Consent for publication
Not applicable
Competing interests
The authors declare no competing interests.
Additional information
Publisher’s note
Springer Nature remains neutral with regard to jurisdictional claims in published maps and institutional affiliations.
Rights and permissions
Springer Nature or its licensor (e.g. a society or other partner) holds exclusive rights to this article under a publishing agreement with the author(s) or other rightsholder(s); author self-archiving of the accepted manuscript version of this article is solely governed by the terms of such publishing agreement and applicable law.
About this article
Cite this article
Miao, G., Ao, S., Chen, X. et al. Fabrication of semicircular micro-grooves on Ti6Al4V by through-mask scanning electrochemical machining. Int J Adv Manuf Technol 126, 3175–3192 (2023). https://doi.org/10.1007/s00170-023-11325-0
Received:
Accepted:
Published:
Issue Date:
DOI: https://doi.org/10.1007/s00170-023-11325-0