Abstract
The effects of the following drugs: nimodipine (1 mg/kg b. w., i. p.), 2-amino-5-phosphonovaleric acid (4mg/kg b.w., i.p.) and propentofylline (25mg/kg b.w., i.p.), administered (alone or in combination) at the end of 15 min bilateral ischemia in gerbils were evaluated on mitochondrial superoxide dismutase (SOD), glutathione reductase (GR), glucose-6 phosphate dehydrogenase (G6PD), monoamine oxidase (MAO) activities, and thiobarbituric acid reactive material (TBARM), and brain water content at 1 hour of reperfusion. The combined treatment virtually abolished early postischemic brain edema (4.1% v.s. 0.6%) and efficiently counteracted ischemia-induced changes [decreased SOD (79% v.s. 98%), GR (52% v.s. 105%) and MAO (25% v.s. 79%), and increased TBARM (198% v.s. 108%)]. The same combination of drugs administered 15 min before ischemia had a similar effect (e.g., reduced brain swelling and lipid peroxidation) as when given at the end of ischemia, whereas a limited or absent impact was seen when the drugs were given 15 min or 1 hour after ischemia, respectively. The data suggest that (post)ischemic brain swelling and mitochondrial dysfunction can be reduced by drugs which synchronously prevent processes induced in the early stages of reperfusion.
Similar content being viewed by others
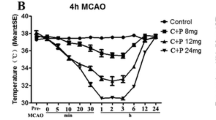
References
Djuricic, B. M., Pashen, W., Bosma, H. J., and Hossman, K. A. 1983. Biochemical changes during graded brain ischemia in gerbils. Part I. Global biochemical alterations. J. Neurol. Sci. 58: 25–36.
Siesjo, B. K. 1981. Cell damage in the brain: a speculative synthesis. J. Cerebral Blood Flow Metabol. 1: 155–185.
Meyer, F. B., Anderson, R. E., Yaksh, T. L., and Sundt, T. M. 1986. Effect of nimodipine on intracellular brain pH, cortical blood flow, and EEG in experimental focal cerebral ischemia. J. Neurosurg. 64: 617–626.
Goto, Y., Okamoto, S., Yonekawa, Y., Taki, W., Kikuchi, H., Handa, H., and Kito, M. 1988. Degradation of phospholipid molecular species during experimental cerebral ischemia in rats. Stroke 19: 728–735.
Globus, M. Y. T., Busto, R., Dietrich, W. D., Martinez, E., Valdes, J., and Ginsberg, M. D. 1988. Effect of ischemia on the in vivo release of striatal dopamine, glutamate and gamma-aminobutyric acid studied by intracerebral microdialysis. J. Neurochem. 51: 1455–1464.
Agardh, C.-D., Zhang, H., Smith, M. L., and Siesjo, B. K. 1991. Free radical production of ischemic brain damage: influence of postischemic oxygen tension. Int. J. Devel. Neurosci. 9: 127–138.
Kogure, K., Arai, H., Abe, K., and Nakano, M. 1985. Free radical damage of the brain following ischemia. Pages 237–259, in Kogure, K., Hossman, K. A., Siesjo, B. K. and Welsh, A. F. (eds.), Progress of Brain Research, Elsevier Science Publishers B. V., Amsterdam, New York, Oxford.
Dumuis, A., Sebben, M., Haynes, L., Pin, J. P., and Bockaert, J. 1988. NMDA receptors activate the arachidonic acid cascade system in striatal neurons. Nature 336: 68–70.
Cvejic, V., Micic, D. V., and Mrsulja, B. B. 1981. Catecholamine turnover in cerebral cortex and caudate during long-term reflow following transient ischemia in gerbils. Pages 261–265, in Mayer, J. S., Lechner, H., Reivich, M., Ott, E. O., and Aranibar, A. (eds.), Cerebral Vascular Disease, Excerpta Medica, Amsterdam Oxford. Princeton.
Kinuta, Y., Kimura, M., Itokawa, Y., Ishikawa, M., and Kikuchi, H. 1989. Changes in xanthine oxidase in ischemic rat brain. J. Neurosurg. 71: 417–420.
Chan, P. K., Schmidley, W. J., Fishman, A. R., and Longar, M. S. 1984. Brain injury, edema, and vascular permeability changes induced by oxygen-derived free radicals. Neurology 34: 315–320.
Hall, E. D., and Braughler, M. 1989. Central nervous system trauma and stroke. II Physiological and pharmacological evidence for involvement of oxygen radicals and lipid peroxidation. Free Radical Biology & Medicine 6: 303–313.
Mrsulja, B. B., Micic, D. V., and Stefanovich, V., 1985. Propentofylline and postischemic brain edema: relation to Na-K-ATPase activity. Drug Develop. Res. 6: 339–344.
Stanimirovic, D., Djuricic, B. M., and Mrsulja, B. B. 1988. Glutathione reductase during and after brain ischemia in gerbils. Metabolic Brain Disease 3: 293–296.
Elliot, K. A. C., and Jasper, H. 1949. Measurement of experimentally induced brain swelling and shrinkage. Am. J. Physiol. 157: 122–129.
Gurd, W. J., Jones, R. L., Mahler, R. H., and Moore, J. 1974. Isolation and partial characterization of rat brain synaptic membrane. J. Neurochem. 22: 281–290.
Lowry, O. H., Rosebrough, N. J., Farr, A. L., Randall, R. J. 1951. Protein measurement with the Folin phenol reagent. J. Biol. Chem. 193: 952–958.
Sun, M., and Zigman, S. 1978. An improved spectrophotometric assay for superoxide dismutase based on epinephrine autoxidation. Anal. Biochem. 90: 81–89.
Lowry, O. H., and Passonneau, J. V. 1974. A Flexible System of Enzymatic Analysis. Academic Press, New York.
Krajl, M. 1963. A rapid microfluorometric determination of monoamine oxidase. Biochem. Pharmacol. 14: 1684–1685.
Rehncrona, S., Smith, D. S., Akesson, B., Westenberg, E., and Siesjo, B. K. 1980. Peroxidative changes in brain cortical fatty acids and phospholipids, as characterized during Fe++-and ascorbic acid-stimulated lipid peroxidation in vitro. J. Neurochem. 34: 1630–1638.
Pulsinelli, W. A. 1985. Selective neuronal vulnerability: morphological and molecular characteristics. Pages 29–37, in Kogure, K., Hossman, K.-A., Siesjo, B. K., and Welsh, F. A. (eds.), Progress in Brain Research, Vol 63, Elsevier Science Publishers B. V., Amsterdam, New York, Oxford.
Ishii, H., Stanimirovic, D. B., Chang, C. J., Mrsulja, B. B., and Spatz, M. 1993. Dopamine metabolism and free-radical related mitochondrial injury during transient brain ischemia in gerbils. Neurochem. Res. 18: 1193–1201.
Maker, S. H., Weiss, C., Slides, J. D., and Cohen, G. 1981. Coupling of dopamine oxidation (monoamine oxidase activity) to glutathione oxidation via generation of hydrogen peroxide in the rat brain homogenates. J. Neurochem. 36: 589–593.
Matsuyama, T., Michishita, H., Nakamura, H., Tsuchiyama, M., Shimitzu, S., Watanabe, K., and Sugita, M. 1993. Induction of copper-zinc superoxide dismutase in gerbil hippocampus after ischemia. J. Cerebral Blood Flow Metab. 13: 135–144.
Michowiz, S. D., Melamed, E., Pikarsky, E., and Rappaport, Z. H. 1990. Effect of ischemia induced by middle cerebral artery occlusion on superoxide dismutase activity in rat brain. Stroke 21: 105–110.
Wagner, K. R., Kleinholz, M., and Myers, R. E. 1990. Delayed onset of neurologic deterioration following anoxia/ischemia coincides with appearance of impaired brain mitochondrial respiration and decreased cytochrome oxidase activity. J. Cerebral Blood Flow Metab. 10: 417–423.
Nakahara, I., Kikuchi, H., Taki, W., Nishi, S., Kito, M., Yonekawa, Y., Goto, Y., and Ogata, N. 1992. Changes in major phospholipids of mitochondria during postischemic reperfusion in rat brain. J. Neurosurg. 76: 244–250.
Heffez, D. S., Nowak, T. S., Passonneau, J. V. 1985. Nimodipine levels in gerbil brain following parenteral drug administration. J. Neurosurg. 63: 589–592.
DeLeo, J., Toth, L., Schubert, P., Rudolphi, P., and Kreutzberg, W. G. 1987. Ischemia-induced neuronal cell death, calcium accumulation, and glial response in the hippocampus of the Mongolian gerbil and protection by propentophylline (HWA-285). J. Cerebral Blood Flow Metab. 7: 745–751.
Meldrum, B. 1985. Possible therapeutic applications of antagonists of excitatory amino acid neurotransmitters. Clin. Sci., 68: 113–122.
Ginsberg, M. D. 1993. Emerging strategies for the treatment of ischemic brain injury. Pages 207–237,in Waxman, S. G. (ed.), Molecular and Cellular Approaches to the Treatment of Neurological Diseases. Raven Press Ltd., New York.
Harris, R. J., Branston, N. M., Symon, L., Bayhan, M., and Watson, A. 1982. The effects of a calcium antagonist, nimodipine, upon physiological responses of the cerebral vasculature and its possible influence upon foral cerebral ischemia. Stroke, 13: 759–766.
Gelmers, J. H. 1987. Nimodipine in ischemic stroke. Clin. Neuropharmacol. 10: 412–422.
Porche, E., and Stefanovich, V. 1982. The effect of 1-(5′-oxohexyl)-3-methyl-7-propyl-xanthine on the respiratory activity of the rat brain mitochondria. Drug Res. 32: 1–5.
Fredholm, B. B., and Lindstrom, K. 1986. The xanthine derivative 1-(5′-oxohexyl)-3-methyl-7-propyl xanthine (HWA 285) enhances the action of adenosine. Acta Pharmacol. Toxicol. 58: 187–192.
Miller, L. P., and Hsu, C. 1992. Therapeutic potential for adenosine receptor activation in ischemic brain injury. J. Neurotrauma. 9: S563-S577.
Globus, M. Y. T., Dietrich, W. D., Busto, R., Valdes, J., and Ginsberg, M. D. 1989. The combined treatment with dopamine D1 antagonist (SCH-23390) and NMDA receptor blocker (MK-801) dramatically protect against ischemia-induced hippocampal damage. J. Cerebral Blood Flow Metab. 9: S 5.
Krieglstein, J., Sief El Nasr, M., Sauer, D., Mennel, D. H., and Rossberg, C. 1989. Inhibition of postichemic cell death by various drug combinations. J. Cerebral Blood Flow Metab. 9: S648.
Uematsu, D., Araki, N., Greenberg, J. H., Sladky, J., and Reivich, M. 1991. Combined therapy with MK-801 and nimodipine for protection of ischemic brain damage. Neurology 41: 88–94.
Author information
Authors and Affiliations
Rights and permissions
About this article
Cite this article
Stanimirovic, D.B., Micic, D.V., Markovic, M. et al. ‘Therapeutic window’ for multiple drug treatment of experimental cerebral ischemia in gerbils. Neurochem Res 19, 189–194 (1994). https://doi.org/10.1007/BF00966815
Accepted:
Issue Date:
DOI: https://doi.org/10.1007/BF00966815