Abstract
Using non-invasive techniques (CO2 gas exchange, light scattering, light absorption, chlorophyll fluorescence, chlorophyll luminescence), we have analysed the response of respiration and photosynthesis to dehydration and rehydration of leaves of the resurrection plants Craterostigma plantagineum Hochst., Ramonda mykoni Reichb. and Ceterach officinarum Lam. et DC. and of the drought-sensitive mesophyte spinach (Spinacia oleracea L.). The following observations were made: (i) The rate of water loss during wilting of detached leaves of drought-tolerant resurrection plants was similar to that for leaves of the sensitive mesophyte, spinach. Leaves of Mediterranean xerophytes lost water much more slowly. (ii) Below a residual water content of about 20%, leaves of spinach did not recover turgor on rewatering, whereas leaves of the resurrection plants did. (iii) Respiration was less sensitive to the loss of water during wilting in the resurrection plants than in spinach. (iv) The sensitivity of photosynthesis to dehydration was similar in spinach and the resurrection plants. Up to a water loss of 50% from the leaves, photosynthesis was limited by stomatal closure, not by inhibition of reactions of the photosynthetic apparatus. Photosynthesis was inhibited and stomates reopened when loss of water became excessive. (v) After the leaves had lost 80% of their water or more, the light-dependent reactions of photosynthetic membranes were further inhibited by rewatering in spinach; they recovered in the resurrection plants. (vi) In desiccated leaves of the resurrection plants, slow rehydration reactivated mitochondrial gas exchange faster than photosynthetic membrane reactions. Photosynthetic carbon assimilation recovered only slowly.
Similar content being viewed by others
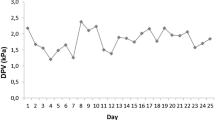
References
Bewley, J.D. (1979) Physiological aspects of desiccation tolerance. Annu. Rev. Plant Physiol. 30, 195–238
Björkmann, O., Powles, S.B. (1984) Inhibition of photosynthetic reactions under water stress: Interactions with light level. Planta 161, 490–504
Briantais, J.-M., Vernotte, C., Krause, G.H., Weis, E. (1986) Chlorophyll a fluorescence of higher plants: Chloroplasts and leaves. In: Light emission by plants and bacteria, pp. 539–577, Govindjee, Amesz, J., Fork, D.C., eds. Academic Press, New York
Crowe, J.H., Cooper, A.F., Jr. (1971) Cryptobiosis. Sci. Am. 225, 30–36
Dietz, K.-J., Heber, U. (1983) CO2-gas exchange and the energy status of leaves of Primula palinuri. Planta 158, 349–356
Gaff, D.F., Hallam, N.D. (1974) Resurrecting desiccated plants. R. Soc. N. Z. Bull. 12, 389–393
Gaff, D.F., Loveys, B.R. (1984) Abscisic acid content and its effects during dehydration of detached leaves of desiccation tolerant plants. J. Exp. Bot. 35, 1350–1358
Heber, U. (1969) Conformational changes of chloroplasts induced by illumination of leaves in vivo. Biochim. Biophys. Acta 180, 302–319
Heber, U., Santarius, K.A. (1964) Loss of adenosine thriphosphate synthesis caused by freezing and its relationship to frost hardiness problems. Plant Physiol. 39, 712–719
Hetherington, S.E., Smillie, R.M. (1982) Humidity sensitive degreening and regreening of leaves of Borya nitida Labill. as followed by changes in chlorophyll fluorescence. Austr. J. Plant Physiol. 9, 587–599
Hincha, D.K., Höfner, R., Schwab, K.B., Heber, U., Schmitt, J.M. (1987) Membrane rupture is the common cause of damage to chloroplast membranes in leaves injured by freezing or excessive wilting. Plant Physiol. 83, 251–253
Hincha, D.K., Müller, M., Hillmann, T., Schmitt, J.M. (1989) Osmotic stresses cause mechanical freeze-thaw injury to thylakoids in vitro and in vivo. In: Biochemical and physiological mechanisms associated with environmental stress tolerance in plants. Proc. of a NATO-workshop, Norwich, U.K., Cherry, J., Davies, D.D., eds. in press
Junge, W. (1977) Membrane potentials in photosynthesis. Annu. Rev. Plant Physiol. 28, 503–536
Kaiser, K., Gaff, D.F., Outlaw, W.H., Jr. (1986) Sugar content of leaves of desiccation sensitive and desiccation tolerant plants. Naturwissenschaften 85, 608–609
Kaiser, W.M., Kaiser, G., Prachuab, P.K., Wildman, S.G., Heber, U. (1981a) Photosynthesis under osmotic stress. Inhibition of photosynthesis of intact chloroplasts, protoplasts and leaf slices at high osmotic potentials. Planta 153, 416–422
Kaiser, W.M., Kaiser, G., Schöner, S., Neimanis, S. (1981b) Photosynthesis under osmotic stress. Differential recovery of photosynthetic activities of stroma enzymes, intact chloroplasts and leaf slices after exposure to high solute concentrations. Planta 153, 430–435
Kaiser, W.M., Schwitulla, M., Wirth, E. (1983) Reactions in chloroplasts, cytoplasm and mitochondria of leaf slices under osmotic stress. Planta 158, 302–308
Köster, S., Heber, U. (1982) Light scattering and quenching of 9-aminocridine fluorescence as indicators of the phosphorylation state of the adenylate system in intact spinach chloroplasts. Biochim. Biophys. Acta 680, 88–94
Krause, G.H. (1973) The high energy-state of the thylakoid system as indicated by chlorophyll fluorescence and chloroplast shrinkage. Biochim. Biophys. Acta 292, 715–728
Leopold, A.C., Musgrave, M.E., Williams, K.M. (1981) Solute lenkage resulting from leaf desiccation. Plant Physiol. 68, 1222–1225
Malkin, S. (1977) Delayed luminescence. In: Primary processes of photosynthesis, pp. 351–427, Barber, J., ed. Elsevier, Amsterdam
Mollenhauer, A., Schmitt, J.M., Caughlan, S.J., Heber, U. (1983) Loss of peripheral membrane proteins from thylakoids during freezing. Biochim. Biophys. Acta 728, 331–339
Olabiyi, O., Kaiser, W.M., Heber, U. (1983) Respiratory activities of plant mitochondria under osmotic stress. Z. Pflanzenphysiol. 111, 155–167
Powles, S.B. (1984) Photoinhibition of photosynthesis induced by visible light. Annu. Rev. Plant Physiol. 35, 15–44
Renger, G., Schreiber, U. (1986) Practical applications of fluorimetric methods to algae and higher plant research. In: Light emission by plants and bacteria, pp. 587–619, Govindjee, Amesz, J., Fork, D.C., eds. Academic Press, New York
Santarius, K.A. (1973) The protective effect of surgars on chloroplast membranes during temperature and water stress and its relationship to frost, desiccation and heat resistance. Planta 113, 23–46
Schreiber, U., Bilger, W. (1987) Rapid assessment of stress effects on plant leaves by chlorophyll fluorescence measurements. In: Plant response to stress, pp. 27–53, Tenhunen, J., Catarino, F., Lange, O.L., Oechel, W., eds. Springer, Berlin
Schreiber, U., Schliwa, U., Bilger, W. (1986) Continuous recording of photochemical and non-photochemical chlorophyll fluorescence quenching with a new type of modulation fluorometer. Photosynth. Res. 10, 51–62
Schwab, K.B. (1986) Morphologische, physiologische und biochemische Anpassungsstrategien austrocknungstoleranter höherer Pflanzen. Doctoral thesis, Universität Würzburg
Schwab, K.B., Gaff, D.F. (1986) Sugar and ion contents in leaf tissues of several drought tolerant plants under water stress. J. Plant Physiol. 125, 257–265
Schwab, K.B., Heber, U. (1984) Protection of thylakoid membranes from resurrection plants against dehydration. In: Advances in photosynthesis research, vol. 3, pp. 403–406, Sybesma, C., ed. Martinus Nijhoff, Dr. W. Junk Publishers, The Hague
Steponkus, P.L. (1984) Role of the plasma membrane in freezing injury and cold acclimitation. Annu. Rev. Plant Physiol. 35, 543–584
Steponkus, P.L., Dowgert, M.F., Gordon-Kamm, W.J. (1983) Destabilization of the plasma membrane if isolated plant protoplasts during a freeze-thaw cycle: The influence of cold acclimation. Cryobiology 20, 448–465
Volger, H.U., Heber, U., Berzborn, R.J. (1978) Loss of function of biomembranes and solubilization of membrane proteins during freezing. Biochim. Biophys. Acta 511, 455–469
Walter, H. (1955) The water economy and the hydrature in plants. Annu. Rev. Plant Physiol. 6, 239–252
Wiltens, J., Schreiber, U., Vidaver, W. (1978) Chlorophyll fluorescence induction: an indicator of photosynthetic activity in marine algae undergoing desiccation. Can. J. Bot. 50, 2787–2794
Wolter, F.P., Schmitt, J.M., Bohnert, H.J., Tsugita, A. (1984) Simultaneous isolation of three peripheral proteins — a 32 kDa protein, ferredoxin-NADP+-reductase and coupling factor — from spinach thylakoids and partial characterization of the 32 kDa protein. Plant. Sci. Lett. 34, 323–334
Author information
Authors and Affiliations
Rights and permissions
About this article
Cite this article
Schwab, K.B., Schreiber, U. & Heber, U. Response of photosynthesis and respiration of resurrection plants to desiccation and rehydration. Planta 177, 217–227 (1989). https://doi.org/10.1007/BF00392810
Received:
Accepted:
Issue Date:
DOI: https://doi.org/10.1007/BF00392810