Abstract
Background
Deep brain stimulation (DBS) is a well-established and highly effective treatment for patients with medically uncontrolled Parkinson’s disease (PD). This study presents the outcome of patients with PD after subthalamic deep brain stimulation (STN DBS) using the microtargeting the platform (MTP) stereotactic system (the STarFixSystem, FHC Inc., Bowdoin, Me., USA) for accurate localization of the target and precise placement of DBs electrodes. Patients were evaluated preoperatively and the follow up period was 1 year utilizing the Unified Parkinson’s Disease Rating Scale (UPDRS II and III) in on and off medication-stimulation conditions. It included 18 STN DBS procedures in 10 patients over a 2-year period. The technical features and the practical application of the STarFix system and the clinical outcome are reported. Also lead location analysis is done by doing postoperative CT to evaluate the clinical accuracy of the stereotactic system.
Results
The mean age of PD patients was 67.7 years. Six patients were males (60%) and 4 patients were females (40%). The mean postoperative improvement in ADL was 83.47 ± 2.39 over Dopa therapy alone. The mean postoperative improvement in UPDRs motor score was 78.96 ± 7.74 over Dopa therapy alone. The STarFix system showed high accuracy with target error 1.89 mm (SD 0.8) without accounting for brain shift.
Conclusion
Deep brain stimulation (DBS) targeting the subthalamic nucleus (STN) offers fundamental benefits for patients with advanced Parkinson’s disease (PD). The usage of the STarFix system for implanting DBS electrodes in the STN provides an accurate, safe, and effective alternative to traditional stereotactic techniques. This approach simplifies the surgical procedure, boosts patient comfort, and minimizes the duration of the operation.
Clinical trial registration ClinicalTrials.gov identifier: NCT03562403. Registered 19 June 2018, https://classic.clinicaltrials.gov/ct2/show/NCT03562403.
Similar content being viewed by others
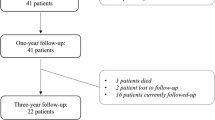
Background
Parkinson's disease (PD) is a common neurodegenerative condition affecting approximately 1% of individuals aged over 65 [1]. It is characterized by the loss of dopaminergic cells in the ventrolateral area of the substantia nigra and the presence of Lewy bodies, primarily in the brainstem [2]. Motor dysfunction, particularly bradykinesia, is the primary clinical hallmark for diagnosing PD, in addition to cardinal symptoms like resting tremors, rigidity, or postural instability. As the disease advances, managing symptoms becomes increasingly challenging due to motor fluctuations, medication-resistant symptoms, and medication-related side effects [1, 3].
Deep brain stimulation (DBS) has been proved as an effective surgical treatment for movement disorders like PD, with applications expanding to other conditions such as depression, obsessive–compulsive disorders, pain, and epilepsy. The success of DBS relies on the precise implantation of electrodes into small intracranial targets. Traditionally, DBS surgery has used rigid stereotactic systems mounted to the patient's skull, but recent advancements have introduced alternative methods to improve patient comfort, shorten surgical time, and enable precise bilateral electrode implantation [4].
One such alternative method involves the STarFix system, a customized surgical targeting fixture developed by FHC Inc (Bowdoin.Me.,USA). This system involves organizing software, bone fiducial markers, and the production of a customized mini stereotactic platform (MTP) that attaches to the bone fiducials.
Materials and methods
This study presents a prospective analysis of the practical application and clinical outcomes of STN DBS using the STarFix system. The study involved ten patients who underwent surgery at the Department of Neurosurgery, Lake Cumberland Regional Hospital, University of Louisville, Kentucky, USA, between July 2018 and July 2021. A multidisciplinary team, including movement disorder neurologists, functional neurosurgeons, neuropsychologists and a patient coordinator, systematically assessed each patient to ensure that the surgical approach would yield successful results.
Patient selection
Patient selection for DBS is a critical aspect of the procedure. Inclusion criteria comprised a diagnosis of idiopathic PD, responsiveness to dopaminergic medication, and a minimum disease duration of 4 years. Surgery was considered when motor symptoms were inadequately controlled with medications or when medication side effects became problematic. Responsiveness to dopaminergic medication was evaluated using the Unified Parkinson’s Disease Rating Scale (UPDRS), with a 30% reduction in UPDRS III considered an appropriate criterion for surgery [5]. Exclusion criteria included significant medical comorbidities, cognitive impairment, bleeding disorders, brain atrophy, or an inadequate response to dopamine, which would contraindicate DBS.
The study population included 10 PD patients, encompassing 18 STN DBS procedures, with a focus on bilateral implantation to address motor symptoms on both sides and optimize medication reduction.
Target selection
The subthalamic nucleus (STN) was selected as the primary target for DBS in PD. Bilateral STN DBS is typically performed to alleviate motor symptoms on both sides and facilitate medication reduction.
Study protocol
All patients underwent comprehensive clinical evaluations using the UPDRS for motor and activities of daily living (ADL) assessments before surgery and during follow-up at different intervals. Patients underwent detailed history-taking, neurological examinations, and brain imaging using CT and/or MRI to rule out any abnormalities that could affect target selection. Informed consent was obtained from each patient, and medications were withheld for 12 h before surgery.
Data collection
A data collection sheet was designed to gather patient demographics, medical history, preoperative neurological examinations, and details on CT and MR images, as well as intraoperative and early postoperative recovery information.
Methods
In this study, patients underwent STN DBS implantation using the STarFix system’s customized stereotactic frames (MTP). The STarFix system utilized bone fiducial markers attached to a personalized microtarget platform stereotactic system (MTP). These bone-anchored fiducial markers served as reference points for imaging and anchors for a custom-manufactured stereotactic frame (Fig. 1).
Surgical procedure
The STN DBS implantation procedure using the MTP consists of three stages:
Stage I Initial Outpatient Procedure: During this stage, bone fiducial markers are implanted, and imaging is conducted. CT and MRI scans are acquired while the patient is under general anesthesia to ensure motion-free images, enhancing trajectory planning accuracy. Bone anchor placement and image acquisition are combined in a single outpatient process.
The patient receives anesthetics while he is on the CT table, and the scalp is cleaned and scrubbed following proper sterile techniques. The skull is screwed for each bone anchor through a small stab in the scalp. Patients undergoing unilateral DBS implantation receive three bone anchors, while those undergoing bilateral implantation receive four. These bone anchors are completely hidden under the scalp without externalized hardware, then the patient goes home. Patients are advised to have the anchor sites clean with an antiseptic until the day of surgery. Non-contrast CT images are taken with a 1 mm slice thickness, and MRI with contrast is done using a 3-T magnet (typically with a voxel dimension of 1 × 1 × 1 mm) (Fig. 2).
Planning software compatible with the STarFix MTP system, the StimPilot™ (Medtronic Neurological Inc., Minneapolis, Minn., USA), the Voxim™, and the microTargeting™WayPoint™ planner (FHC Inc.) is used. CT and MRI files are imported into the software and co-registered, and targets are chosen on the MRI images through the use of the Schaltenbrand and Wahren Atlas coordinates for the STN [6, 7]. The STN is targeted at 11–12 mm lateral to the midcommissural point, 3 mm posterior, and 6 mm inferior. Once targets, trajectories, and bone fiducial markers are shown, a personalized MTP is made by the help of the software, and either a single or double-trajectory MTP frame can be asked for. Typically, the trajectory specific MTP arrives at the hospital within 3 days (Fig. 1).
Stage II Inpatient Procedure: This stage involves electrode implantation and macrostimulation, performed under local anesthesia approximately 1–2 weeks after Stage I. Patients remain awake during this stage. They are positioned supine on the operating table with their heads slightly elevated and not locked to the table. The area just above the scalp incision is shaved, sterilized, and covered, leaving the face uncovered. Burr holes are drilled 2 cm lateral to the midline and about 1 cm anterior to the coronal suture to avoid ventricular wall penetration.
The MTP is securely attached to the existing bone anchors using provided couplers (Fig. 3). The entry point is marked, and a burr hole is drilled accordingly. An electronic microTargeting™ drive is attached on the MTP to guide leads through the planned trajectories for macrostimulation (Fig. 4). Once the optimal tract and target are identified through macrostimulation, the the quadripolar DBS lead (model 3387 or 3389; Medtronic Neurological Inc.) takes the place of the test electrode.
Proper targeting is confirmed through macrostimulation, performed by the neurologist responsible for postoperative programming. The final DBS lead is secured to the skull, with the extracranial end buried under the scalp. The MTP and bone fiducial markers are taken out, and a postoperative CT is obtained within 24 h to detect any structural complications.
Stage III Subsequent Outpatient Procedure: This stage includes planning for a subclavicular pectoral pocket for the battery. The connection of the DBS electrode to an internal pulse generator is done as an outpatient process while the patient is under general anesthesia on a different day. The battery (Medtronic, Soletra Type) is positioned correctly, with its connection border facing upward and any excess wire positioned behind it.
Results
Our study involved 18 STN DBS implantation procedures in 10 PD patients, at the time of surgery the average age was 67.7 years (56–77 Years), 60% of the patients were males, and 40% were females. And the average duration of symptoms ranged from (6 to 20 years), with a mean of 11.4 years (Table 1). The most common comorbidity was hypertension, present in 7 patients, all 10 patients exhibited tremors and rigidity, while 3 had bradykinesia, and 5 had abnormal gait.
2 patients underwent unilateral STN DBS electrode implantation, while 8 patients underwent bilateral STN DBS implantation over a 2-year period. Postoperative complications included seizures in 2 patients, a small subdural hematoma in 1 patient, photophobia in 1 patient, diplopia in 1 patient, and difficulty projecting the voice in 1 patient. All complications were managed and resolved within 3 months of follow-up.
In terms of clinical outcomes, there was a significant improvement in ADL (83.47 ± 2.39) with STN DBS compared to medication alone. The improvement in UPDRS motor score with STN DBS (78.96 ± 7.74) over medication alone was also highly significant. These improvements were consistent across all follow-up time points compared to preoperative conditions. Tremor, rigidity, and bradykinesia all showed substantial improvement with STN DBS (Tables 2, 3). The PD patients progress after 3, 6 months and 1 year as shown in Figs. 5, 6, 7, 8.
On 1-year follow-up, patients experienced excellent control of tremors in 80% of cases and complete resolution of bradykinesia in 100% of cases. Abnormal gait also showed excellent control in 80% of patients. Any residual symptoms were managed through reprogramming. Postoperative medications were reduced in eight patients and completely stopped in two patients.
Discussion
Advancements in our understanding of the pathophysiology of movement disorders and the anatomy and physiology of the basal ganglia have played a crucial role in identifying surgical targets for movement disorder interventions. Moreover, improvements in neuroradiology and neurophysiological mapping techniques have made target localization easier, leading to enhanced surgical outcomes and increased safety in surgical interventions for movement disorders [8].
Over the last few decades, Deep Brain Stimulation (DBS) has become a widely adopted treatment for various conditions. It all began in 1989 when Benabid and his colleagues first used DBS to treat tremors. The implantation of DBS systems can be carried out using various methods, stereotactic systems, image-guided targeting, and intraoperative microelectrode recordings (MERs) and testing. There are now multiple hardware and software options available for DBS, including different types of leads and implanted generators [9]. Deciding who the optimal candidate for surgery is and determining the suitable treatment strategy for each patient requires a multidisciplinary team.
The effectiveness of DBS depends on the experience of the neurosurgical team and the characteristics of the patient. Accurate patient selection depends on their symptoms, age, and cognitive status is essential because the proper selection of patients can significantly enhance the efficacy of DBS [10]. Patients with nonmotor symptoms who do not respond to medication are unlikely to benefit from DBS. A multidisciplinary team approach is critical for successful DBS, including thorough preoperative evaluation and ongoing postoperative care after the placement of DBS hardware. Ethical considerations in medical practice are an integral part of this multidisciplinary management [11].
In this study we report on the outcomes and experiences of managing advanced Parkinson's disease (PD) patients through STN DBS implantation using the STarFix system. The study covers the period from 2018 to 2020, involving 10 patients and 18 STN DBS implantation procedures. The patients were followed up for 1 year. The results align with findings from other studies in many aspects. The study utilized CT and MRI-guided target localization and employed various methods to enhance image quality and spatial resolution, including CT and MRI fusion [12].
The mean age of PD patients in this study was 67.7 years, ranging from 56 to 77 years. Mathkour et al. 2017 reported on 37 patients and the mean age was 72.45 years [13]. Also, Sharma et al. 2019 reported on 30 patients with a mean age of 77.5 years (range 75.0–84.5 years) [14]. Other studies have reported varying mean ages, highlighting the age diversity among PD patients. Male patients were predominant in this series (77%), consistent with the male predilection observed in other studies [15, 16].
The average duration of symptoms in this study was 11.4 years, while other studies have reported longer durations. It was 13 (5–25) years in a study by Thomsen et al., 2020 [17]. This indicates a trend among neurologists and neurosurgeons to delay surgical intervention in PD patients to maximize the potential benefits of medical treatment.
In the study by Rabie et al. (2016), they reported a significant improvement of 73.8% in Activities of Daily Living (ADL) scores when patients were in the medication off state [18]. Pusswald et al. (2019) observed a range of improvement in ADL scores between 60.0 and 90.0% in the medication off state [19]. In comparison, our study demonstrated a 72.74 ± 3.44% improvement in ADL scores in the medication off state, with values ranging from 65.38 to 76.67%.
Regarding the Unified Parkinson’s Disease Rating Scale (UPDRS) motor score, Rabie et al. (2016) reported a postoperative improvement of 49.3% [18]. Varma et al. (2003) reported a slightly higher improvement of 61% [20]. In our study, we observed a 67.4 ± 10.96% improvement in the UPDRS motor score in the medication off state. Shin et al. (2020) also found significant improvements in both UPDRS motor and UPDRS ADL scores in the medication off state, with a p-value of less than 0.0001 [21].
These findings collectively suggest that Deep Brain Stimulation (DBS) has a positive effect on both Activities of Daily Living and motor function, particularly when patients are in the medication off state. The exact degree of improvement may vary between different studies, but the trend consistently indicates substantial enhancements in these aspects of Parkinson's disease following DBS treatment.
The study results demonstrate substantial improvements in various motor aspects of Parkinson's disease (PD) after unilateral and bilateral Subthalamic Nucleus Deep Brain Stimulation (STN DBS). Tremors showed a remarkable improvement of 80.9% with a statistically significant result (P < 0.001). This indicates a significant reduction in tremor severity in patients who underwent STN DBS. Rigidity improved by 51.0% with a statistically significant result (P < 0.001). This suggests a significant alleviation of muscle rigidity, which is a common symptom in PD. Bradykinesia, characterized by slowness of movement, improved by 40.2% with a statistically significant result (P < 0.001). This indicates that STN DBS led to a substantial increase in movement speed and agility in patients.
Overall, there was a significant improvement in all motor aspects evaluated by the Unified Parkinson's Disease Rating Scale Part III (UPDRS III), which includes assessments of tremor, rigidity, and bradykinesia. This improvement was also reflected in the UPDRS II (Activities of Daily Living—ADL) scores, suggesting that patients experienced enhanced daily functioning and quality of life as a result of STN DBS. Additionally, the study noted a significant reduction in medication doses and a decrease in medication-related side effects, underscoring the potential of STN DBS to not only improve motor symptoms but also diminish the reliance on medication.
Furthermore, when patients were assessed in the “on medication-on stimulation” state, there was still a significant improvement in UPDRS III scores and all motor subscores in comparison to the preoperative state. This suggests that STN DBS continued to provide benefits even when patients were on their regular medication regimen, emphasizing the enduring positive impact of this treatment approach.
The postoperative results in our study and those of Rabie et al. (2016), Varma et al. (2003), Shin et al. (2020), and Pusswald et al. (2019) consistently indicate significant improvements in the condition of patients with Parkinson's disease (PD) after Subthalamic Nucleus Deep Brain Stimulation (STN DBS) [18,19,20,21]. This implies that the quality of life for these patients improved compared to their baseline condition, particularly in the medication-off state.
Some studies have reported significant improvements in the Unified Parkinson's Disease Rating Scale Part III (UPDRS III) score 1 year after STN DBS [22]. However, there are variations in the long-term outcomes. Some studies have shown a deterioration in UPDRS III scores over longer follow-up periods [23,24,25]. These findings suggest that while STN DBS can lead to significant short-term improvements, the long-term impact on motor symptoms may vary, and some patients may experience a decline in motor function over time. On the other hand, UPDRS II scores, which assess activities of daily living (ADL), proved little improvement in comparison to the preoperative "on" state in some studies, and this lack of improvement was statistically non-significant at longer follow-up periods [22, 26, 27]. This suggests that STN DBS may have a more limited impact on ADL in the "on" state, and the benefits in this aspect of PD management may be less pronounced or enduring.
Overall, the effectiveness and long-term outcomes of STN DBS can vary among individuals, and the treatment's impact on motor symptoms and daily functioning may evolve over time. Long-term follow-up and continued assessment are crucial for understanding the full spectrum of effects that STN DBS has on PD patients' quality of life and motor function.
Complications of STN stimulation in this study fell within an acceptable range, with all reported complications being reversible and improving within 3 months. These complications can be classified as procedure-related, hardware-related, or stimulation-related [28]. The overall incidence of complications was 11%, but it varies among studies [29]. Death is an exceedingly rare occurrence after STN DBS surgery.
The incidence of device-related complications in Deep Brain Stimulation (DBS) procedures, as reported by Hamani et al. (2005), is approximately 9% [30]. These complications typically involve issues related to infections, problems with the leads, and pulse generator-related problems. Additionally, they reported a 2.8% incidence of intracerebral hemorrhage (ICH), which is a relatively rare but serious complication.
In our study, it is reassuring to note that there were no reported cases of postoperative intracerebral hemorrhage (ICH). ICH is a critical concern in neurosurgical procedures, and its absence in our study suggests that the surgical team maintained a high level of precision and care during the procedure.
However, our study did report one patient (5.5%) who developed a small subdural hematoma. It's important to note that subdural hematomas are a potential complication of neurosurgical procedures, and their spontaneous resolution is a positive outcome. The relatively low incidence in our study, along with the favorable resolution, suggests that the surgical team was attentive to postoperative monitoring and management.
Procedure-related complications in Deep Brain Stimulation (DBS) procedures can encompass various issues [28, 29, 31]. Intracranial Hemorrhage reported incidence rates typically range from 0.0 to 3.9%. Intracranial hemorrhage is a critical complication. The incidence may vary depending on various factors, including patient characteristics and surgical techniques. Suboptimal Electrode placement reported incidence rates range from 0 to 2.5%. Suboptimal electrode placement refers to the improper positioning of the DBS electrode within the target area of the brain. Precise electrode placement is crucial for the success of DBS therapy. Infection reported incidence in DBS procedures ranges from 3 to 10%. Infection is a potential complication associated with the device. Maintaining aseptic (sterile) operative techniques, administering intraoperative antibiotics, and irrigating the wound with antibiotic solutions are essential measures to prevent infection. Short surgical times can also help minimize the risk of infection. Hardware-Related Complications account for 5% to 18.5% of the total complications. These complications may involve issues with the DBS leads, pulse generator, or other hardware components. Proper selection of hardware and meticulous surgical technique can help reduce the risk of hardware-related problems.
Overall, while DBS is an effective treatment for various neurological conditions, it is not without risks. The incidence of complications can vary depending on several factors, including the surgical approach, patient characteristics, and the experience of the surgical team. To mitigate these risks, maintaining strict adherence to sterile techniques, antibiotic prophylaxis, and careful surgical planning and execution are essential elements of successful DBS procedures.
Stimulation-related complications are commonly observed after Deep Brain Stimulation (DBS) surgery, but they are often reversible or manageable through adjustments to the stimulation parameters. Many stimulation-related complications, such as mood changes and confusion, can be reversed or mitigated by fine-tuning the DBS stimulation parameters. This flexibility allows clinicians to optimize the therapy for each patient's specific needs. Several studies have indicated that DBS in the Subthalamic Nucleus (STN) does not have a significant negative impact on overall cognitive functions. Moreover, any cognitive changes that do occur are often reversible. This suggests that DBS can be a relatively safe treatment option in terms of cognitive functioning [32,33,34]. Perioperative confusion, which can occur during the DBS surgery or in the immediate postoperative period, is typically transient. Multiple factors may contribute to its development, including the penetration of frontal lobes during surgery, the prolonged duration of the procedure, and the withdrawal of dopaminergic medications.
Other reversible stimulation-related complications involve eye deviation, facial pulling, contralateral numbness, speech disturbances, and dysphonia. Stimulation sometimes cause psychiatric adverse effects including acute depression. These complications are reversible when the stimulation parameters are changed [35].
While stimulation-related complications can occur, they are generally manageable, and the benefits of DBS therapy often outweigh the potential side effects. Close monitoring and communication between the patient and the medical team are essential for optimizing the stimulation parameters and addressing any adverse effects that may arise during the course of DBS treatment.
In the context of Deep Brain Stimulation (DBS) procedures, the incidence of intracerebral hemorrhage (ICH) is generally lower when compared to radiofrequency (RF) lesioning procedures. Reported rates of ICH in DBS surgery can vary but typically range from 0.3% to 3.6% per electrode track. This suggests that while ICH is a known potential complication of DBS, it is relatively rare compared to other neurosurgical interventions [36]. Some factors may increase the risk of ICH during DBS surgery. Gorgulho et al. (2005) reported that the presence of microelectrode recording, and hypertension can elevate the risk of ICH [37]. Therefore, it's crucial to maintain good control of perioperative blood pressure to minimize this risk. Screening patients for coagulopathy (a disorder of blood clotting) or recent use of antiplatelet agents is important. Patients with these risk factors may be at a higher risk for bleeding complications, including ICH. Identifying and addressing these factors preoperatively can help reduce the risk. The choice of the entry point for electrode insertion is a critical consideration. Care must be taken to avoid injury to superficial cortical veins and sulci under the entry point, as damage to these structures can increase the risk of bleeding and ICH.
Overall, while ICH is a known potential complication of DBS surgery, it occurs relatively infrequently. The careful management of patient risk factors, perioperative blood pressure control, and precise surgical technique are essential measures to minimize the risk of ICH in DBS procedures.
It's important to note that individual responses to DBS can vary, and not all patients will experience these complications. The ability to adjust the stimulation parameters is a valuable aspect of DBS therapy, as it allows for the optimization of treatment while minimizing adverse effects. Close collaboration between patients and healthcare providers is crucial for managing and mitigating these reversible complications effectively.
In our research, we shared our practical experience utilizing the STarFix MicroTargeting Platform (MTP) system for performing Subthalamic Nucleus Deep Brain Stimulation (STN DBS) in patients diagnosed with Parkinson's disease (PD). Notably, we did not encounter any technical complications while employing this system.
It's worth mentioning that the STarFix MTP system has demonstrated a commendably low incidence of technical issues, as reported by Konrad et al. in 2011, with a rate of 0.1% or less. This suggests that the system is highly reliable and user-friendly for neurosurgical procedures like STN DBS.
Furthermore, when comparing the risk of bone marker dislodgement with patients where the traditional stereotactic frame becomes disconnected from the skull, our findings indicate that the STarFix MTP system offers a level of stability and precision that is on par with other established systems, as supported by studies by Fitzpatrick et al. in 2005 and Ball et al. in 2020 [38, 39].
In summary, our study and the referenced research by Konrad et al. (2011), as well as the work by Fitzpatrick et al. (2005) and Ball et al. (2020), underscore the effectiveness and precision of the STarFix MTP system when it comes to guiding and facilitating STN DBS procedures [4, 38, 39]. This technology is evidently a valuable asset for neurosurgeons seeking to conduct accurate and successful DBS surgeries.
We observed several benefits associated with using the STarFix MicroTargeting Platform (MTP) system compared to traditional stereotactic systems. One significant advantage was that the patient's head did not need fixation to the operating table. This contributed to reducing patient anxiety and discomfort during the procedure. Additionally, the dual-mount MTP allowed for the simultaneous implantation of electrodes on both sides, enabling bilateral procedures and test stimulation in a single session.
Another noteworthy advantage was the flexibility to perform the CT and MRI scans on a separate day during stage I, after placing the fiducial anchors in the skull under general anesthesia. This approach yielded motion-free images before the surgery. In contrast, traditional frame-based cases required obtaining preoperative CT and MRI scans on the same day as lead implantation, often under local anesthesia, which could cause discomfort and anxiety for patients.
With the STarFix MTP system, the fiducial bone anchors could remain securely implanted for an extended period of 2–3 weeks without an increased risk of infection, as supported by studies by Konrad et al. (2011) and Vinas et al. (1997) [4, 40]. Moreover, the MTP and bone anchors could be reused for multiple procedures without the need to reapply new bone markers. This feature offered the option to stage bilateral DBS implantations without the necessity of repeating the planning process.
However, it's worth noting that a disadvantage of the STarFix MTP system is the time required for MTP frame manufacturing and shipping to the center, which typically takes three days. Nevertheless, this did not pose a problem in practice, as patients were willing to accept the staging of the procedure. The observations and conclusions regarding the advantages and disadvantages of the STarFix system in our report align with those reported in previous studies, as referenced in the work by Vinas et al. (1997), Konrad et al. (2011), and Ball et al. (2020) [4, 38, 40].
Limitations
Our study has certain limitations that should be acknowledged. Firstly, the sample size of patients included in our study was relatively small. To draw more comprehensive and robust conclusions, larger-scale studies with a more extensive patient cohort would be beneficial. Secondly, the postoperative follow-up period in our study was limited to 1 year. Given the chronic nature of Parkinson's disease and the potential for long-term effects and complications related to Deep Brain Stimulation (DBS), it would be advantageous to conduct studies with longer follow-up periods. This extended observation would provide valuable insights into how DBS-related complications may impact the quality of life and overall outcomes in patients with Parkinson's disease over an extended duration.
In summary, while our study has provided reliable alternative for the use of DBS in Parkinson's disease patients using the STarFix MTP system, it is critical to recognize these limitations and consider them in the context of future research and clinical practice. Conducting larger-scale, long-term studies will contribute to a more comprehensive understanding of the benefits and potential challenges associated with this therapeutic approach.
Conclusions
In conclusion, our findings demonstrate that Subthalamic Nucleus Deep Brain Stimulation (STN DBS) is an effective intervention for improving motor symptoms, enhancing activities of daily living, and alleviating drug complications in patients with advanced Parkinson's disease (PD).
Furthermore, the utilization of the STarFix system for STN DBS implantation offers a reliable, safe, well-tolerated, and efficient alternative to traditional stereotactic systems for the placement of DBS electrodes. This system is characterized by its simplicity of use, which not only enhances accuracy but also contributes to improved patient comfort and reduced surgical operating time.
Taken together, our study underscores the efficacy and advantages of STN DBS as a treatment modality for advanced PD, while also highlighting the merits of the STarFix system as a valuable tool for facilitating this therapeutic approach.
Availability of data and materials
All data generated or analyzed during this study are available from the corresponding author upon request.
Abbreviations
- PD:
-
Parkinson’s disease
- DBS:
-
Deep brain stimulation
- STN:
-
Subthalamic nucleolus
- ICH:
-
Intracerebral hemorrhage
- ADl:
-
Activity of daily living
- UPDRS:
-
Unified Parkinson’s Disease Rating Score
- CT:
-
Computerized tomography
- MRI:
-
Magnetic resonance imaging
- RF:
-
Radiofrequency
References
Hartmann CJ, Fliegen S, Groiss SJ, Wojtecki L, Schnitzler A. An update on best practice of deep brain stimulation in Parkinson’s disease. Ther Adv Neurol Disord. 2019;12:1756286419838096. https://doi.org/10.1177/1756286419838096.
Dickson DW, Fujishiro H, Orr C, DelleDonne A, Josephs KA, Frigerio R, et al. Neuropathology of non-motor features of Parkinson disease. Parkinsonism Relat Disord. 2009;15(Suppl 3):S1-5. https://doi.org/10.1016/s1353-8020(09)70769-2.
Mehanna R, Fernandez HH, Shukla AW, Bajwa JA. Deep brain stimulation in Parkinson’s disease. Parkinson’s Disease. 2018;2018:9625291. https://doi.org/10.1155/2018/9625291.
Konrad PE, Neimat JS, Yu H, Kao CC, Remple MS, D’Haese P-F, et al. Customized, miniature rapid-prototype stereotactic frames for use in deep brain stimulator surgery: initial clinical methodology and experience from 263 patients from 2002 to 2008. Stereotact Funct Neurosurg. 2011;89(1):34–41. https://doi.org/10.1159/000322276.
Groiss SJ, Wojtecki L, Südmeyer M, Schnitzler A. Deep brain stimulation in Parkinson’s disease. Ther Adv Neurol Disord. 2009;2(6):20–8. https://doi.org/10.1177/1756285609339382.
Niemann K, Naujokat C, Pohl G, Wollner C, Keyserlingk DV. Verification of the Schaltenbrand and Wahren stereotactic atlas. Acta Neurochir. 1994;129(1):72–81. https://doi.org/10.1007/BF01400876.
Zonenshayn M, Mogilner A, Rezai AR. Neurostimulation and functional brain imaging. Neurol Res. 2000;22(3):318–25.
Smith Y, Wichmann T, Factor SA, DeLong MR. Parkinson’s disease therapeutics: new developments and challenges since the introduction of levodopa. Neuropsychopharmacology. 2012;37(1):213–46. https://doi.org/10.1038/npp.2011.212.
Paff M, Loh A, Sarica C, Lozano AM, Fasano A. Update on current technologies for deep brain stimulation in Parkinson’s disease. J Mov Disord. 2020;13(3):185–98. https://doi.org/10.14802/jmd.20052.
Dallapiazza RF, De Vloo P, Fomenko A, Lee DJ, Hamani C, Munhoz RP et al. Considerations for patient and target selection in deep brain stimulation surgery for Parkinson’s disease. In: Stoker TB, Greenland JC (eds). Parkinson’s disease: pathogenesis and clinical aspects. Brisbane (AU): Codon Publications. Copyright: The Authors. 2018.
Pollak P. Deep brain stimulation for Parkinson’s disease—patient selection. In: Brain stimulation. Elsevier; 2013. p. 97–105. https://doi.org/10.1016/B978-0-444-53497-2.00009-7.
Honey CR, Nugent RA. A prospective randomized comparison of CT and MRI pre-operative localization for pallidotomy. Can J Neurol Sci. 2000;27:236–40.
Mathkour M, Garces J, Scullen T, Hanna J, Valle-Giler E, Kahn L, et al. Short- and long-term outcomes of deep brain stimulation in patients 70 years and older with Parkinson disease. World Neurosurg. 2017;97:247–52. https://doi.org/10.1016/j.wneu.2016.06.001.
Sharma VD, Lyons KE, Nazzaro JM, Pahwa R. Deep brain stimulation of the subthalamic nucleus in Parkinson’s disease patients over 75 years of age. J Neurol Sci. 2019;399:57–60. https://doi.org/10.1016/j.jns.2019.02.019.
Shpiner DS, Di Luca DG, Cajigas I, Diaz JS, Margolesky J, Moore H, et al. Gender disparities in deep brain stimulation for Parkinson’s disease. Neuromodul Technol Neural Interface. 2019;22(4):484–8. https://doi.org/10.1111/ner.12973.
Hitti FL, Ramayya AG, McShane BJ, Yang AI, Vaughan KA, Baltuch GH. Long-term outcomes following deep brain stimulation for Parkinson’s disease. J Neurosurg. 2019;132:1–6.
Thomsen BLC, Jensen SR, Clausen A, Karlsborg M, Jespersen B, Løkkegaard A. Deep brain stimulation in Parkinson’s disease: still effective after more than 8 years. Mov Disord Clin Pract. 2020;7(7):788–96. https://doi.org/10.1002/mdc3.13040.
Rabie A, Verhagen Metman L, Fakhry M, Eassa AYE, Fouad W, Shakal A, et al. Improvement of advanced Parkinson’s disease manifestations with deep brain stimulation of the subthalamic nucleus: a single institution experience. Brain Sci. 2016;6(4):58.
Pusswald G, Wiesbauer P, Pirker W, Novak K, Foki T, Lehrner J. Depression, quality of life, activities of daily living, and subjective memory after deep brain stimulation in Parkinson disease—a reliable change index analysis. Int J Geriatr Psychiatry. 2019;34(11):1698–705. https://doi.org/10.1002/gps.5184.
Varma TRK, Fox SH, Eldridge PR, Littlechild P, Byrne P, Forster A, et al. Deep brain stimulation of the subthalamic nucleus: effectiveness in advanced Parkinson’s disease patients previously reliant on apomorphine. J Neurol Neurosurg Psychiatry. 2003;74(2):170–4. https://doi.org/10.1136/jnnp.74.2.170.
Shin HW, Kim MS, Kim SR, Jeon SR, Chung SJ. Long-term effects of bilateral subthalamic deep brain stimulation on postural instability and gait difficulty in patients with Parkinson’s disease. J Mov Disord. 2020;13(2):127–32. https://doi.org/10.14802/jmd.19081.
Herzog J, Volkmann J, Krack P, Kopper F, Pötter M, Lorenz D, et al. Two-year follow-up of subthalamic deep brain stimulation in Parkinson’s disease. Mov Disord. 2003;18(11):1332–7. https://doi.org/10.1002/mds.10518.
Wider C, Pollo C, Bloch J, Burkhard PR, Vingerhoets FJG. Long-term outcome of 50 consecutive Parkinson’s disease patients treated with subthalamic deep brain stimulation. Parkinsonism Relat Disord. 2008;14(2):114–9. https://doi.org/10.1016/j.parkreldis.2007.06.012.
Weaver FM, Follett KA, Stern M, Luo P, Harris CL, Hur K, et al. Randomized trial of deep brain stimulation for Parkinson disease: thirty-six-month outcomes. Neurology. 2012;79(1):55–65. https://doi.org/10.1212/WNL.0b013e31825dcdc1.
Castrioto A, Lozano AM, Poon Y-Y, Lang AE, Fallis M, Moro E. Ten-year outcome of subthalamic stimulation in Parkinson disease: a blinded evaluation. Arch Neurol. 2011;68(12):1550–6. https://doi.org/10.1001/archneurol.2011.182.
Krause M, Fogel W, Mayer P, Kloss M, Tronnier V. Chronic inhibition of the subthalamic nucleus in Parkinson’s disease. J Neurol Sci. 2004;219(1–2):119–24. https://doi.org/10.1016/j.jns.2004.01.004.
Limousin P, Foltynie T. Long-term outcomes of deep brain stimulation in Parkinson disease. Nat Rev Neurol. 2019;15(4):234–42. https://doi.org/10.1038/s41582-019-0145-9.
Chan DT, Zhu XL, Yeung JH, Mok VC, Wong E, Lau C, et al. Complications of deep brain stimulation: a collective review. Asian J Surg. 2009;32(4):258–63. https://doi.org/10.1016/s1015-9584(09)60404-8.
Kleiner-Fisman G, Herzog J, Fisman DN, Tamma F, Lyons KE, Pahwa R, et al. Subthalamic nucleus deep brain stimulation: summary and meta-analysis of outcomes. Mov Disord. 2006;21(Suppl 14):S290-304. https://doi.org/10.1002/mds.20962.
Hamani C, Richter E, Schwalb JM, Lozano AM. Bilateral subthalamic nucleus stimulation for Parkinson’s disease: a systematic review of the clinical literature. Neurosurgery. 2005;56(6):1313–21. https://doi.org/10.1227/01.neu.0000159714.28232.c4.
Fenoy AJ, Simpson RK Jr. Risks of common complications in deep brain stimulation surgery: management and avoidance. J Neurosurg. 2014;120(1):132–9. https://doi.org/10.3171/2013.10.jns131225.
Witt K, Daniels C, Reiff J, Krack P, Volkmann J, Pinsker MO, et al. Neuropsychological and psychiatric changes after deep brain stimulation for Parkinson’s disease: a randomised, multicentre study. The Lancet Neurol. 2008;7:605–14.
Shakal A, Ramadan E, Fawaz W. Effect of deep brain stimulation on motor and mental status of Parkinson’s disease patients: A preliminary Egyptian study. Age (years). 2011;14(48.1):12.5.
Accolla EA, Pollo C. Mood effects after deep brain stimulation for Parkinson’s disease: an update. Front Neurol. 2019;10:617.
Slavin K, Thulborn K, Wess C, Nersesyan H. Direct visualization of the human subthalamic nucleus with 3T MR imaging. Am J Neuroradiol. 2006;27(1):80–4.
Rosenow JM, Rezai AR. Surgical technique and complication avoidance. In: Surgical management of movement disorders. CRC Press; 2005. p. 67–84.
Gorgulho A, De Salles AA, Frighetto L, Behnke E. Incidence of hemorrhage associated with electrophysiological studies performed using macroelectrodes and microelectrodes in functional neurosurgery. J Neurosurgery. 2005;102(5):888–96. https://doi.org/10.3171/jns.2005.102.5.0888.
Ball TJ, John KD, Donovan AM, Neimat JS. Deep brain stimulation lead implantation using a customized rapidly manufactured stereotactic fixture with submillimetric euclidean accuracy. Stereotact Funct Neurosurg. 2020;98(4):248–55.
Fitzpatrick JM, Konrad PE, Nickele C, Cetinkaya E, Kao C. Accuracy of customized miniature stereotactic platforms. Stereotact Funct Neurosurg. 2005;83(1):25–31.
Vinas FC, Zamorano L, Buciuc R, Li QH, Shamsa F, Jiang Z, et al. Application accuracy study of a semipermanent fiducial system for frameless stereotaxis. Comput Aided Surg. 1997;2(5):257–63.
Acknowledgements
We are thankful to both Professor Joseph Neimat for his great help and advice in teaching DBS surgery and Professor Magdy Elkeleeny for his valuable help and comments on the earlier version of the manuscript.
Funding
This research received no specific grant from any funding agency in the public, commercial or not-for-profit sectors.
Author information
Authors and Affiliations
Contributions
AE and AN shared in all phases of the research and made the review of literature and wrote the manuscript. AE, MR and AE shared in analyzing the data, paper writing and revision. All authors read and approved the final manuscript.
Corresponding author
Ethics declarations
Ethics approval and consent to participate
Institutional Review Board (IRB) number 17200211 was granted by the Faculty of Medicine's ethics committee at Assiut University. This study was registered at ClinicalTrials.gov with the ClinicalTrials.gov identifier (NCT number): NCT03562403. The study adhered to the World Medical Association's Declaration of Helsinki. The patients provided their written informed consent for DBS surgery. All patients signed forms consenting the clinic to store and analyze the data.
Consent for publication
Written consent for publication was obtained for all patients with approval for publication of figures and data.
Competing interests
The authors declared no potential conflicts of interest concerning this research, authorship, and/or publication of this article.
Additional information
Publisher's Note
Springer Nature remains neutral with regard to jurisdictional claims in published maps and institutional affiliations.
Rights and permissions
Open Access This article is licensed under a Creative Commons Attribution 4.0 International License, which permits use, sharing, adaptation, distribution and reproduction in any medium or format, as long as you give appropriate credit to the original author(s) and the source, provide a link to the Creative Commons licence, and indicate if changes were made. The images or other third party material in this article are included in the article's Creative Commons licence, unless indicated otherwise in a credit line to the material. If material is not included in the article's Creative Commons licence and your intended use is not permitted by statutory regulation or exceeds the permitted use, you will need to obtain permission directly from the copyright holder. To view a copy of this licence, visit http://creativecommons.org/licenses/by/4.0/.
About this article
Cite this article
Elnaggar, A., Elshanawany, A., Elgheriany, A.E. et al. Subthalamic deep brain stimulation in advanced Parkinson’s disease using the STarFix system. Egypt J Neurosurg 39, 44 (2024). https://doi.org/10.1186/s41984-024-00281-w
Received:
Accepted:
Published:
DOI: https://doi.org/10.1186/s41984-024-00281-w