Abstract
Background
We used the Caribbean spiny lobster Panulirus argus, one of the largest brooding invertebrates in the Western Atlantic, to test for the presence/absence of active parental care and to explore reproductive performance in large brooding marine organisms. Given [i] the compact and large embryo masses produced by P. argus, [ii] the expected disproportional increase in brooding costs with increasing embryo mass size, and [iii] the theoretical allometry of egg production with increasing body size, we predicted that parental females in this large species will engage in active brood care. We also predicted that larger broods from larger lobsters should suffer higher mortality and brood loss than smaller broods from smaller lobsters if parental care was minimal or absent. Lastly, we expected smaller females to allocate disproportionably more resources to egg production than larger females in the case of minimal parental care.
Results
Females brooding early and late embryos were collected from different reefs in the Florida Keys Reef tract, transported to the laboratory, and maintained in separate aquaria to describe and quantify active parental care during day and night. A second set of females was retrieved from the field and their carapace length, fecundity, egg size, reproductive output and presence/absence of brood-dwelling pathogens was recorded. Laboratory experiments demonstrated that brooding females of P. argus engaged in active brood care. Females likely use some of the observed behaviors (e.g., abdominal flapping, pleopod beating) to provide oxygen to their brood mass. In Panulirus argus, females did not suffer brood loss during embryo development. Also, reproductive output increased more than proportionally with a unit increase in lobster body weight.
Conclusions
Our results agree with the view that large brooding marine invertebrates can produce large embryo masses if they engage in active parental care and that the latter behavior greatly diminishes reproductive performance costs associated with producing large embryo masses. Lastly, we report on a nemertean worm that, we show, negatively impacts female reproductive performance.
Similar content being viewed by others
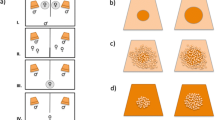
Background
The degree of parental care varies broadly among marine invertebrates, even within monophyletic clades [1, 2]. At one extreme, some groups do not provide any form of parental care, spawning small unfertilized or fertilized eggs into the pelagic environment in which development of embryos and/or larvae takes place (various bivalves and sea urchins [3, 4]). At another extreme, some species with abbreviated or direct development produce large yolky eggs that are brooded and hatch as advanced larval stages or juveniles. These early ontogenetic stages might remain in the parental brood chamber and/or dwelling for long periods of time and can be fed, defended, and groomed by females (various seastars, isopods, and amphipods, among others [5–7]). In between extremes, many species exhibit indirect development, and parental care is restricted to the protection of embryos incubated in bodily chambers of varying complexity (e.g., many crustaceans, including spiny lobsters [8]). Explaining the evolution and adaptive value of parental care is one of the most relevant yet still not completely understood problems in evolutionary biology [1, 2].
In marine brooding invertebrates, benefits to brooded offspring include, but are not limited to, protection against predators [5, 9, 10], protection from adverse abiotic conditions [5, 9, 10], and/or physiological provisioning [11]. Still, brooding embryos in bodily chambers is not exempt of costs. For instance, reproductive performance (i.e., fecundity, reproductive output) of parental females might decrease due to brood loss, in turn, driven by increases in embryo volume during development, embryo crowding and loss from the abdominal chamber [12]. Embryo masses accumulate sediment, detritus, bacteria, algae, fungi, and many other epizootic organisms (e.g., ciliates) that might further impact parental reproductive performance [13–16]. Egg predators are known to destroy embryo masses when experiencing population outbreaks, impacting not only female fecundity but also the host population health [17–19]. Perhaps more importantly, large densely packed embryo masses can be considered living tissue but without a circulatory system [20]. Most embryo masses are larger than the theoretical 1 mm limiting thickness that allows sufficient oxygen supply by diffusion [21] and oxygen limitation does occur at their centers [20, 22, 23]. Oxygen depletion at the interior of embryo masses has been shown to be severe, even early during embryo development, when respiration rates of early embryos are much lower than those of late embryos [20]. Hypoxic conditions do impact embryo development, often driving asynchronous development within embryo masses (e.g., periphery versus center) [9, 24, 25] and even embryo mortality [26].
Likely, parental behaviors exclusively directed toward embryo masses, as reported for various invertebrates, have evolved as a mechanism to retard or prevent fouling, repel egg-predators, and improve oxygen availability to developing embryos [14, 20, 27]. Ultimately, active parental care is expected to, and has been shown to, improve reproductive performance. For instance, grooming of the brood mass by parental females increases embryo survival and hatching rates [14, 27]. Similarly, ventilation of brooded embryos by parental females (e.g., abdominal flapping or pleopod fanning [23, 25]) is known to increase oxygen levels at the center and periphery of embryo masses and appears to speed up embryo development [25]. Our understanding of what constitutes 'active parental care' has improved considerably during the last decade thanks to studies focused on a few marine invertebrates [25, 27–30]. Nonetheless, whether or not active parental care is the rule rather than the exception in brooding marine invertebrates still remains an open question.
In marine brooding invertebrates, brooding costs should be considerable in large females from large species, as they have the potential to produce massive embryo masses and also suffer the putatively heavy costs of brooding [31, 32]. Theoretical considerations suggest that the costs associated with brooding (i.e., oxygen provision, grooming) increase non-linearly with increases in brood mass, potentially resulting in the inability of large adults to successfully rear all brooded embryos [23–25]. Furthermore, with increases in body size, the capacity for egg production is also expected to scale at a pace greater than the space available for brooding, and thus, larger adults might be capable of producing more gametes than can be successfully brooded [33, 34] (Fig. 1a).
Relationship between body size and egg production constraining/favoring brooding and the model species. a Hypothetical allometric relationship between body size and egg production (continuous curve), and brooding space (dashed curve) in marine invertebrates (after [34]). In the figure, the capacity for egg production increases faster than the space available for brooding. The conditions above are expected to favor brooding in small but not large marine invertebrates and determine heavy costs, in terms of reproductive investment, for large brooding marine invertebrates. b The Caribbean spiny lobster Panulirus argus. Photo credit: J. Antonio Baeza
Overall, large species of brooding marine invertebrates should suffer exacerbated brooding costs, resulting in poor reproductive performance, unless these large parental individuals allocate considerable time and energy to attending their broods [23]. Indeed, brood mass size and parental behaviors are likely interlinked; large parental individuals with large broods either allocate a considerable amount of energy and time to brood their embryos, or suffer the costs in terms of reproductive performance, when producing large embryo masses. Various studies during the last decades have demonstrated that physiological costs (i.e., increased metabolic rate) are considerable in females engaging in active parental care [20, 23, 35]. Yet, whether brooding costs also result in diminished reproductive performance in large species of brooding marine invertebrates has been poorly explored. Studies on large species are particularly relevant as it will help to test whether or not excessive physiological costs associated to brooding large embryo masses limit the evolution of brooding in large marine invertebrates [34].
In this study, we are particularly interested in testing whether or not large marine invertebrates engage in active parental care, and whether brooding costs, measured in terms of reproductive performance, are suffered by these brooding species. For this purpose, we used the Caribbean spiny lobster Panulirus argus as a model organism, one of the largest crustaceans in the Atlantic, attaining up to 45 cm in total length [36, 37] (Fig. 1b). As reported for all members of the Pleocyemata, a species-rich clade of crustaceans to which spiny lobsters belong, P. argus produces a large number of embryos that are carried by females underneath the abdomen immediately after spawning and are maintained until they hatch as larvae [38, 39]. The problem of oxygen limitation seems particularly critical in this lobster, which produces compact, large, semi-spherical masses of embryos that frequently exceed 8–10 cm in maximum diameter (JAB, pers. obs). In agreement with the notion above, a recent study has demonstrated asynchronous development of the embryo mass of P. argus: embryos at the periphery develop more quickly (24–48 h ahead) than those at the center [40]. We predict that ovigerous females of P. argus should engage in active brood care given the large embryo masses produced by this species. If the above is correct, then costs in terms of reproductive performance should be low or absent in this large marine invertebrate. By contrast, if P. argus does not engage in active parental care, then we expect considerable costs in terms of reproductive performance, including severe brood loss, decreased reproductive output, as well as negative allometric scaling of brood loss and reproductive output with body size. While studying reproductive performance in P. argus, we noticed the presence of an egg mass-dwelling nemertean belonging to the genus Carcinonemertes. We provide information about the prevalence and impact of this worm on the brood masses of infected lobsters.
Results
Active parental care in Panulirus argus
Six behaviors were observed and recorded in ovigerous and non-ovigerous (control) females of P. argus. Four of these behaviors were classified as events (i.e. abdominal flapping, pleopod fanning, 4th and 5th pereopod probing), while the remaining two behaviors were considered as states (i.e. standing, abdomen extension) (Fig. 2).
Active parental care in the Caribbean spiny lobster Panulirus argus. Different behaviors performed exclusively or predominantly by ovigerous females under laboratory conditions. a–c Abdominal flapping and pleopod beating. Notice the movement of the embryos carried by the pleopods forward (b) and backward (in a and c) while the abdomen is partially extended. Also, watch video in Additional file 1. d 4th pereopod probing in female displaying a standing position. e 5th pereopod probing in female displaying a standing position. In (d) and (e), the arrows point to the 4th and 5th pereopod, respectively
Only one (i.e., 4th pereopod probing, Fig. 2) out of the six recorded behaviors were found to be exclusive to ovigerous females in P. argus. However, the remaining of the recorded behaviors were observed at extremely low frequencies in non-ovigerous females. For instance, abdominal flapping and pleopod fanning were observed for a very short time period only three times in all non-ovigerous females.
Ovigerous females were observed to either exclusively or predominantly perform certain behaviors in a specific sequence. Females first raised their body from the bottom of the aquarium (stand up) by extending the pereopods and, immediately or after a few seconds of raising their bodies, females expanded the abdomen (abdomen extension). Next, females flapped the abdomen (abdominal flapping), fanned the pleopods back and forth (pleopod fanning) or performed both behaviors at the same time repetitively from a few seconds to up to an hour (maximum time period for analysis of behavior) (Additional file 1: Video 1). While standing up and with the abdomen extended, females were also observed to probe their embryos either with the 4th or 5th (chelae bearing) pereopods. The latter two behaviors were performed while females were fanning their embryos or during short time periods in which females stopped flapping and/or fanning the abdomen and/or pleopods. The sequence of the behaviors above lasted from a few seconds to an hour (maximum time period used for measuring female behaviors). After performing the behavioral sequence above for a few seconds, minutes or hours, females either walked around or crouched again at the bottom of the aquarium (Fig. 2).
The frequency or duration of the behaviors above did change depending on embryo developmental stage and/or diel cycle. The frequency of grooming bouts by the 4th pereopods, pleopod fanning / abdominal flapping, and the total time spent in a standing position were greater in females carrying late than early stage embryos (mixed nested ANOVAs: P < 0.05 in all cases; Table 1 and Fig. 3). Also, grooming bouts by the 4th pereopod, and pleopod fanning / abdominal flapping bouts were more frequently performed during night than during day hours (mixed nested ANOVA: P < 0.05 in all cases; Table 1 and Fig. 3). The remainder of the studied behaviors did not vary statistically between females carrying early or late embryos during day or night.
Active parental care in the Caribbean spiny lobster Panulirus argus: behavior of ovigerous and non-ovigerous females under laboratory conditions. The frequency (n bouts per hour) and total percentage of time (% time in 1 h) that each event (discrete acts, < 1 min duration) and state (>1 min duration) was performed by ovigerous and non-ovigerous females carrying embryos at each developmental stage during day (D) and night (N) hours is shown
Reproductive performance in Panulirus argus
A total of 19, 18, 20, and 11 female lobsters carrying embryos in stages I, II, III, and IV, respectively were sampled. The mean (± standard deviation, SD) CL was 76.06 ± 8.95 mm and ranged from 51.3 to 100.4 mm. No significant differences in body size (CL, carapace length) were detected between females carrying embryos in different developmental stages (ANOVA: F = 2.41, df = 3,64, P = 0.0750). A total of five females were found to host nemertean parasites in their brood masses. These parasitized females were not considered for the initial analysis of reproductive investment (but, see below).
Average (± SD, range) fecundity varied between 299 328 (±162 537, 105 858–757 278) embryos lobster−1 and 347 001 (±131 652, 205 569–674 041) embryos lobster−1 in females carrying early and late stage embryos, respectively. An ANCOVA did not detect any effect of embryo stage (I versus IV) on fecundity (F = 0.8, d.f. = 1, 29, P = 0.3784). On the other hand, female body size (CL) did affect fecundity; large females carried more embryos than small females (F = 58.04, d.f. = 1, 29, P < 0.0001). The interaction term of the ANCOVA was not significant (F = 0.1972, d.f. = 1, 29, P = 0.6603) (Fig. 4a). Thus, females, either large or small, did not loose embryos significantly throughout embryo development.
Reproductive performance in the Caribbean spiny lobster Panulirus argus. a Fecundity, relationship between female body size (CL) and egg number in females carrying early and late embryos. b Embryo size, relationship between female body size (CL) and egg volume in females carrying early and late embryos. Bars represent standard deviations. c Reproductive output, relationship between female body weight (dry) and egg mass weight (dry) after log-log transformation of the variables in females carrying early embryos. White, light gray, dark gray, and black dots represent females carrying embryos in stages I, II, III, and IV, respectively. Arrows and circle in (a) and arrows in (c) indicate ovigerous females infected with the egg predator Carcinonemertes sp
Embryo volume varied between 0.0834 (±0.0204, 0.122 - 0.054) mm3 and 0.1008 (±0.0142, 0.1200 - 0.083) mm3 in females carrying early and late stage embryos, respectively. An ANCOVA demonstrated an effect of embryo stage on egg volume (F = 6.55, d.f. = 1, 29, P = 0.0160). In turn, CL did not affect embryo volume (F = 0.20, d.f. = 1, 29, P = 0.6570). The interaction term of the ANCOVA was not significant (F = 0.349, d.f. = 1, 29, P = 0.5589; Fig. 4b). In general, embryo volume in P. argus increased by 17.2 % from early to late stage and females from all body sizes carried similarly sized embryos.
Reproductive output varied between 31.30 and 61.46 % and represented a mean ± SD of 49.21 % (±8.17) of lobster body dry weight. Reproductive output increased with lobster body weight, i.e., RO exhibited positive allometry, as the slope (b = 1.46, SE b = 0.14) of the line describing the relationship between these two variables (after log-log transformation) was significantly greater than unity (t-test: t = 3.29, df = 1, 14, P = 0.0026; Fig. 4c).
Carcinonemertes sp. in brood masses of Panulirus argus
We noticed the presence of parasitic nemertean worms from the genus Carcinonemertes in five (7.4 %) out of the 68 sampled ovigerous females and we estimated density of Carcinonemertes and embryo mortality in four of these infested females (one each carrying embryos in stages I, II, III, and IV, respectively). Density of Carcinonemertes varied between one worm per 1262 embryos (or 0.08 worms per 100 embryos) in the female carrying Stage I embryos (78.45 mm CL) and one worm per 193 embryos (or 0.51 worms per 100 embryos) in the female carrying Stage IV eggs (73.78 mm CL). The mean ± (SD) density of Carcinonemertes in these parasitized females was one worm per 667 ± (460) embryos or 0.24 ± (0.19) worms per 100 embryos (Fig. 5).
Infection by the egg predator Carcinonemertes sp. in embryo masses of the Caribbean spiny lobster Panulirus argus. a Density of Carcinonemertes sp. (number of worms per 100 embryos) in ovigerous females brooding eggs in different stages of development. b Effect of Carcinonemertes sp. in embryo masses of ovigerous females brooding eggs in different stages of development. The proportion of healthy embryos (white bars), dead embryos (gray), and empty capsules (black) in samples from each embryo mass is shown. The photographs show the nemertean egg predator (top right) and the effect of the nemertean on the egg masses of ovigerous lobsters (bottom right)
The mean proportion of empty capsules in the infected females was 12.7 ± (10.8) % and varied between 0.8 % in the female carrying Stage I embryos (78.45 mm CL) and 25 % in the female carrying Stage III embryos (76.1 mm CL). In turn, the number of dead embryos varied between 0 % in the female carrying Stage I embryos (78.45 mm CL) and 23 % in the female carrying Stage III embryos (76.1 mm CL). The mean number of dead embryos in these infected females was 7.2 ± 10.7 %. No empty capsules or dead embryos were noticed in non-infected females (Fig. 5).
We compared fecundity, egg size and reproductive output among infected and non-infected females. Visual examination of the data indicated that fecundity in one of the infected females carrying stage I embryos and the infected female carrying stage II embryos was not dissimilar from that found in non-infected females of similar body size (arrows in Fig. 4a). By contrast, fecundity in the second infected female carrying stage I embryos and in infected females carrying stage III, and IV embryos was much smaller than that expected for non-infected females of similar body size (see encircled data points in Fig. 4a). Indeed, these last three infected females represented data outliers whose fecundity was statistically lower than that expected for a non-infected female of equal body size and carrying embryos in the same stage (Fig. 4a). Carcinonemertes sp. did not affect embryo volume of infected females, regardless of embryo developmental stage. Lastly, reproductive output in one of the infected females carrying stage I embryos was much lower than that expected for non-infected females of the same body size (lower arrow in Fig. 4c).
Discussion
Active parental care in Panulirus argus
We expected the Caribbean spiny lobster Panulirus argus to engage in active brood care, considering the putatively high costs, in terms of reproductive performance, that large brooding marine invertebrates should face [20, 23–25]. Our results agree with the notion above; laboratory observations have clearly shown that ovigerous females do direct precise behaviors toward their embryo masses. These behaviors were performed either exclusively (i.e., 4th pereopod probing, standing) or predominantly by ovigerous females (i.e., abdominal flapping, pleopod fanning, 5th pereopod probing). Therefore, abdominal flapping, pereopod fanning, standing, and pereopod probing, among a few others, can be considered ‘active brood care’ behaviors. We believe that some of the observed behaviors are used by parental females to provide oxygen to their brood mass while others are related to the detection of oxygen conditions and/or foreign objects (e.g., detritus, egg predators) that have the potential to impact reproductive performance [41, 42].
Ovigerous females of P. argus ventilate their embryo masses (i.e., likely increasing oxygen ability at its center) using abdominal flapping and fanning of the pleopods. Abdominal flapping has been demonstrated to be the specific behavior used by ovigerous females to increase oxygen concentration at the center of the brood mass in brachyuran crabs [20, 23, 25]. Abdominal flapping and a second behavior, pleopod fanning, have also been observed in various other groups of crustaceans that engage in active brood care and their role in oxygen delivery to embryos was proposed earlier in brachyuran crabs [15, 16, 22, 43] and clawed lobsters [26], and most recently in caridean shrimps [44]. In turn, the standing position, another behavior frequently observed in ovigerous females of P. argus, probably improves the efficiency of oxygen delivery to embryos in a manner similar to that previously reported for crabs [20]. Experiments measuring particular behaviors concomitantly with changes in oxygen availability at the center of the embryo mass are required to demonstrate that abdominal flapping and pleopod fanning are indeed delivering oxygenated water to the center of the embryo mass in P. argus.
In contrast to abdominal flapping and pleopod fanning, 4th pereopod probing might be used in sensing the environment at the periphery or within the embryo mass of ovigerous lobsters. In decapod crustaceans, including lobsters, the dactyls of pereopods do bear setae that serve as mechanoreceptors or contact chemoreceptors [45–51]. Information gathering from the brood mass via pheromones has been previously reported for brooding female crabs and spiny lobsters hatching their embryos [41, 42, 52–56] and most recently in caridean shrimps [44]. Ovigerous females of P. argus might use their 4th pereopods to detect compounds produced by embryos and/or foreign objects (e.g., detritus, parasites) and respond accordingly, either by providing oxygen or removing particles, respectively. The 5th pereopods, each bearing a small but well developed claw [36], might play a function similar to that of the 4th pereopods, helping both with (chemical) information gathering and removal of exogenous particles that might potentially impact reproductive performance, as shown before in other crustaceans with minute but ornamented claws on their last pair of pereopods (in the anomuran crab Petrolisthes violaceus [27]). Although 4th and 5th pereopod probing was frequently performed by ovigerous females carrying both early and late stage embryos, its putative function might not be entirely efficient as suggested by the presence of predatory worms in the brood mass of a few ovigerous females (see below).
The Caribbean spiny lobster P. argus exhibits active parental care and the intensity with which ovigerous females performed different behaviors differed with embryo developmental stage and time of the day. In particular, abdominal flapping and pereopod fanning were more often performed by females carrying late compared to early embryos, in agreement with preliminary observations (in [41, 42]). Since the frequency of abdominal flapping increases throughout the brooding period, an increase in the amount of oxygen provided to the developing embryos is likely to occur [20]. Oxygen consumption in embryos has been shown to increase dramatically during development in every species of crustacean in which this physiological function has been studied [20, 22, 23, 25, 29, 57–59]. In general, the increased brood care by brooding females throughout embryo development probably serves to avoid problems related to oxygen limitation for the embryos (e.g. increased mortality, low development rate, small size at hatching [9, 24]). Studies on oxygen consumption of embryos through development in P. argus are needed to corroborate the assertion above.
Panulirus argus females are also 'full time mothers' as they engage in parental care both during day and night [60]. Nonetheless, abdominal flapping and pereopod fanning, two behaviors that likely deliver oxygen to the embryos, together with embryo grooming using the 4th pereopods, a third behavior likely involved in information gathering from the brood mass, were more often performed during the day than night. Previous studies examining daily locomotor activity rhythm in P. argus have shown that females are more active at dusk and night than during the day [61, 62]. The information above suggests that ovigerous females of P. argus allocate time and energy differentially during the circadian cycle to different functions likely to optimize resource acquisition and use.
Lastly, previous studies in brachyuran crabs have demonstrated that active parental care does result in metabolic costs to ovigerous females [20, 58]. Herein, we have focused on costs associated with reproductive performance (see below). Nonetheless, studying metabolic costs associated with active brood care in P. argus remains to be addressed. These brooding costs (and others, e.g., lost feeding opportunities) are expected to be considerable in a large brooding marine invertebrate like P. argus as well as in other spiny lobsters [20].
Reproductive performance in Panulirus argus
We expected P. argus to suffer high brooding costs (e.g., elevated brood loss during development), if ovigerous females did not exhibit parental care. By contrast, if parental females engaged in active parental care, then we expected ovigerous females to suffer low brooding costs. Our results agree with the rationale above; we have shown that parental care is considerable in this species and concomitantly, costs measured in terms of reproductive performance were low or absent.
In the Caribbean spiny lobster, fecundity increased with body size as reported for every species of spiny lobster in which this reproductive parameter has been studied [63–71]. Also, average fecundity reported herein for P. argus is within the range expected for a spiny lobster of its body size range. For instance, fecundity varied between 109,865 and 590,530 in P. marginatus from Hawaii, a species in which ovigerous females vary between 54.3 and 105.4 mm CL [72]. Perhaps more importantly, differences in fecundity between females carrying early and late embryos were negligible (i.e., non-statistically significant), and thus, brood loss (if any) did not increase disproportionately with increases in brood size. Our results agree with those reported for the Indo-Pacific ornate rock lobster P. ornatus and the Western Australian rock lobster P. cygnus, two species in which no significant brood loss was observed during incubation [73, 74]. In the few other species of spiny lobsters in which brood loss has been estimated, this has been rather low (7.9 % in P. gracilis [75], 14–17 % in P. gilchristi [76], 10–16 % in P. delagoae [77], 7.2 % in P. inflatus [78]). Palinurus elephas from the western Mediterranean can be considered an exception as brood loss reaches 26 % [70]. Brood loss appears to increase with incubation time in lobsters [79]. Interestingly, brood loss can be considerable in crustaceans that attain much smaller body sizes than spiny lobsters. For instance, in fiddler crabs from the genus Uca that grow no larger than 20 mm in carapace width, brood loss varies and can represent up to 43 % of initial fecundity [80]. In turn, in caridean shrimps, in which ovigerous females attain body sizes no larger than 25 cm total length, brood loss varies between 12 % and can reach up to 74 % (Table 6 in [81]). The information above, albeit limited, suggests an inverse relationship between brood loss and body size within one of many clades of brooding marine invertebrates. This relationship deserves further attention. As yet, that brood loss was negligible in P. argus does agree with the notion that large brooding species that allocate considerable attention to their brood masses experience reduced costs in terms of reproductive performance.
In P. argus, embryo volume did increase, but not considerably, during development. The difference between late and early embryos was, on average, only ~20 %, slightly larger than that reported for other spiny lobsters (egg size increase: 8.3 % in P. delagoae [77]). The modest increase in volume throughout development in embryos of P. argus might alleviate or ease, to some extent, the costs associated with brooding. Studies on oxygen consumption of eggs might help reveal whether or not small changes in embryo size throughout development do represent an adaptation to alleviate brooding costs in large marine invertebrates that produce large embryo masses such as P. argus.
Reproductive output (RO) in P. argus was high (49.21 % ± 8.17 of body dry weight) compared to that of other crustaceans. For instance, in most of brachyuran crabs, RO is limited to about 10 % (range: 3–22 %) of body weight ([82–84], but see [85] for exceptions). In caridean shrimp, with a body shape similar to that of lobsters, RO is slightly greater, varying between ~12 and 21 % of body weight [86, 87]. Unfortunately, to the best of our knowledge, no previous studies have estimated reproductive output in other species of spiny lobsters. Importantly, the scaling of reproductive output with female body weight in P. argus was positively allometric; egg mass did increase more than proportionally with a unit of increase in female body weight. By contrast, brood weight most often exhibits an isometric or nearly isometric constraint with increasing female body size in most brachyuran crabs [85]. A limitation on space available for yolk accumulation in the cephalothorax appears to be the main factor constraining RO and its scaling with body size in other brooding crustaceans [85]. Unlike crabs, but resembling caridean shrimps, the abdomen of lobsters is massive and their ovaries extend out of the cephalothorax into the first abdominal segments ([88], and JAB, pers. obs.). An elongated abdomen most likely allows distension of the organs (including gonads), and subsequent increases in reproductive output, likely explaining the positive allometric increases in RO with body size in P. argus. We argue in favor of additional studies examining constraints driven by body shape in determining RO in marine invertebrates. As yet, the positive allometric scaling of reproductive output with body size in P. argus supports the notion that RO and other reproductive parameters related to reproductive performance do not decrease in large brooding invertebrates that do actively provide intensive brood care.
Carcinonemertes sp. in brood masses of Panulirus argus
Our study reports for the first time an embryo predator from the brood mass of the Caribbean spiny lobster P. argus. Preliminary observations on preserved specimens have confirmed that the observed worms represent a previously undescribed species from the genus Carcinonemertes and its description is underway. The genus Carcinomertes can and does have a major impact on the reproductive performance of crustaceans, and has been implicated in the decline of king crab fisheries [18, 19, 89–91]. A few species of Carcinonemertes have been reported before in spiny lobsters from the Pacific (see Table 1 in [92]). However, the impact of this worm on the reproductive performance of spiny lobsters is unknown [19]. This study demonstrates for the first time the negative impact of Carcinonemertes on the reproductive performance of a spiny lobster. One infected lobster carrying early embryos (Stages I) experienced a significant reduction in fecundity and reproductive output. In turn, lobsters with late stage (III and IV) embryos suffered considerable reductions in fecundity and reproductive output. The above pattern suggests that the effect of the predator on the embryo mass progresses quickly during a single brooding event in P. argus.
Prevalence of Carcinonemertes. sp on P. argus was relatively low (7.4 %) when compared to reports in other infected populations (Callinectes arcuatus – 35 % [93], Libinia spinosa – 69.2 % [94], Randallia ornata – 70 % [95], Panulirus interruptus – 42 % [96]). Importantly, in some crustacean host species, Carcinonemertes might experience population outbreaks, destroying embryo masses of their crustacean hosts, and, sometimes, affecting host population health, and even resulting in decreased crab landings [18, 19, 91]. In the Caribbean, landings of P. argus have declined during the last decades, and various conditions are thought to play a role in such population declines, including hurricanes, overfishing, pollution, poor water quality, hypoxia, temperature extremes, viral disease, or a combination of the conditions above [19, 97]. We argue in favor of future studies focusing on the population biology of Carcinonemertes sp., given the potential of this embryo predator to cause extensive damage to natural populations of the commercially and ecologically important spiny lobster P. argus.
Conclusions
Parental care behaviors have rarely been reported for marine invertebrates (for exceptions see [6, 20, 43, 59], including crustaceans, a species-rich clade with great anatomical, physiological, reproductive, and ecological disparity. We have shown that the Caribbean spiny lobster Panulirus argus, a large marine invertebrate that broods bulky embryo masses, engages in active parental care. Costs, measured in terms of reproductive performance, were absent in this species, reinforcing the idea that brooding can be achieved in marine invertebrates with relatively large body sizes, if parental individuals play a major role in attending to brooded embryos. Active parental care should result in considerable metabolic costs to parental individuals [20]. Future studies in P. argus examining these putative metabolic costs are warranted as they will help to illustrate the relationship between mode of development (i.e., brooding versus broadcasting) and body size, recognized for marine invertebrates [9, 25, 34, 98].
Lastly, our study reports for the first time a nemertean egg predator belonging to the genus Carcinonemertes from the embryo mass of the Caribbean spiny lobster P. argus. We argue in favor of studies aimed at revealing the relative importance of this and other pathogens in explaining declines in the landing of P. argus throughout the wider Caribbean during recent decades.
Methods
Model organism: the Caribbean spiny lobster Panulirus argus
Panulirus argus supports a multimillion-dollar fishery in the Greater Caribbean and Gulf of Mexico, with landings from 1984 to 1998 surpassing 330,187 metric tons [99]. The above explains in great part the large body of literature on its anatomy, life history, ecology, behavior and physiology accumulated during the last decades [36]. Still, despite the commercial value and ecological importance of this large brooding invertebrate, no studies on active brood care have been conducted (for exceptions see [41, 42]) and limited information exists on individual-level reproductive performance [38, 100].
Mating in Panulirus argus may occur year round but peaks in the early spring and summer with an increasing photoperiod and warmer temperatures [101, 102]. Generally, males occupy and defend dens in shallow water and attract females who are ready to spawn [39, 103]. Males transfer a spermatophore to the ventral surface of the female [104]. Larger males have the potential to produce larger spermatophores, but males can scale their spermatophore to the size of the female [105, 106]. Female mating receptivity appears to decrease upon receipt of the spermatophore which is used to fertilize a single clutch of eggs [107]. Clutch size increases with female size [39] and the developing embryos are incubated for approximately 3–4 weeks [108], during which time females may migrate to deep reefs before the embryos hatch [109, 110]. Female can produce 2–4 clutches of eggs per year with larger, older females reproducing earlier and having more clutches per year [111]. Fishing pressure has a strong influence on reproduction, which slows growth [112] and decreases the size (but not the age) at first reproduction [111]. Protection from fishing not only increases the average size of females [113] but may also increase the proportion of reproductively mature females in each size class [114]. Previous studies of commercially exploited marine fishes have suggested that larger females may produce higher quality larvae [115] but in P. argus female size was found to be unrelated to egg size, egg C:N ratio, larvae size, or larvae mortality [106].
Sampling of Panulirus argus
Ovigerous females of P. argus were collected between June 22nd and July 25th, 2015 from various coral reefs (6–20 m depth) along the Florida Reef Tract, 2 to 8 km off Long Key (N 24° 49’26”, W 80°48’48”), Florida Keys, USA. At each locality, lobsters were gently captured by hand (with the aid of a tickle stick and hand net) while scuba diving and transported alive to the laboratory. Lobsters were euthanized in coolers full of ice and maintained therein until measurements on reproductive investment were taken.
In the laboratory, the carapace length (CL) of each lobster was measured using a dial caliper (precision = 0.1 mm). Next, the embryos carried by brooding females were gently removed with micro-forceps and classified according to four different categories. Stage I embryos displayed uniformly distributed yolk; stage II embryos showed cell differentiation and yolk clusters; stage III embryos had well-developed eyes and some visible chromatophores but no obvious development of abdomen and thoracic appendages; stage IV embryos possessed well-developed eyes and chromatophores, free abdomens, and thoracic appendages.
Lastly, ovigerous lobsters used to test whether or not P. argus engages in active parental care (see below) were collected during the same time period and from the same coral reefs above and maintained in 3.8 L transparent glass aquaria (51 × 51 × 30 cm3) filled with aerated sea water (25–31 °C and 35–38 ppt) in an outdoor wet lab with natural photoperiod (day : night = ~ 13: 11 h) before being used for experiments.
Active parental care in Panulirus argus
To test whether ovigerous females of P. argus engaged in active parental care (i.e. displaying behaviors likely resulting in the provision of oxygen to and maintenance of brooded embryos) female behavior was recorded under laboratory conditions. Females with embryos at different stages of development (I and IV, n = 3 per stage) were placed individually in a 3.8 L transparent glass aquarium (51 × 51 × 30 cm3) filled with aerated sea water (25–31 °C and 35–38 ppt) in an outdoor wet lab with natural photoperiod (day : night = ~ 13: 11 h). The behavior of each female was videotaped continuously over a 24-h period using a Brinno High Dynamic Range (HDR) Time Lapse Camera - TLC200 Pro, starting 1 h after the lobster was introduced to the tank. Infrared illuminators (Model IRLamp6, 40° beam angle, Wildlife Engineering) were used to continuously record female behavior throughout the night. Behavior of ovigerous females during the experimental period was classified and quantified as events or states, according to their relative duration [20]. Behaviors classified as states occurred over relatively long time periods (more than 1 min, e.g. standing) while discrete acts of relatively short duration (less than 1 min, e.g. pleopod beating) were classified as events most often occurring in bouts [116]. The frequency of the occurrence of each behavioral event (n° event bouts h−1) and the proportion of time that females spent in each behavioral state (% time h−1) were recorded during two haphazardly selected time blocks of 1 h each, for females carrying embryos at the two developmental stages. Time blocks were randomly selected when females were facing laterally, diagonally, or frontally to the camera, and their behavior could be recorded. Also, to identify brooding (i.e., active parental care) from non-brooding behaviors, non-ovigerous females (n = 3) were recorded and their behavior was measured as described above.
To test whether behaviors related to brood care varied during embryonic development, the frequency or percentage of time that each behavior was performed per unit of time (1 h) was compared between day and night (daytime effect) among brooding females carrying embryos at different developmental stages (embryo stage effect: early vs late or non-brooding vs early eggs vs late eggs depending upon the dependent variable, see Table 1) using independent mixed nested ANOVAs [117]. In the different ANOVAs, daytime and embryo stage were treated as fixed effects while female identity was treated as a random effect [117]. The behavior of non-brooding females was included in these ANOVAs only when these females performed the events or states analyzed more than once. Whenever, a specific event or state was observed only once or not observed in each one of the three non-brooding females, the behavior was not considered part of the behavioral repertoire of these females, and was not included in the mixed nested ANOVAs. Data were square root or log-transformed in order to meet the assumptions of the statistical model [117].
Reproductive performance in Panulirus argus
We estimated three different individual-level reproductive performance parameters in brooding females of P. argus: fecundity, embryo size, and reproductive output. For this purpose, we gently detached with forceps the totality of the embryos carried by females underneath the abdomen. Then, ten embryos from each mass were sub-sampled randomly, and the lengths along the short and long axis of each embryo were measured under the microscope (Leica S8AP0) to a precision of 0.001 mm. Embryo volume was estimated with the formula for the volume of an ellipsoid [118], EV = 1 / 6(L S2 π), where L = long axis and S = short axis. The effect of female body size (CL, covariate) and egg stage (Stage I vs IV, main factor) on egg volume was tested using an ANCOVA [117].
Next, four sub-samples of 100 embryos each were isolated from the brood mass of each female and dried with the respective remaining embryo mass and female body for at least 72 h at 70 °C and weighed to the nearest 0.01 mg with an analytical balance (Sartorius; ± 0.1 mg). Fecundity was calculated with the equation F = EmMass / (Ess1 + Ess2 + Ess3 + Ess4) * 400 + 400, where F = total number of embryos, and EmMass = dry weight of the remaining embryo mass after the four egg sub-samples (Ess1-4) have been taken. The effect of female body size (CL) and egg stage (Stage I vs IV) on fecundity was tested using an ANCOVA [117].
Lastly, reproductive output was estimated as the ratio between dry weight of embryos and dry weight of the females carrying early embryos (Stage I). This latter parameter represents the amount of resources (biomass) that females invest in reproduction [119]. We tested whether reproductive output increased linearly (isometrically) with female body size. The relationship between egg dry mass and female body dry mass was examined using the allometric model y = a*xb [120]. The slope b of the log-log least-squares linear regression represents the rate of power increase (b > 1) or decrease (b < 1) of the estimate of reproductive allocation with a unit of increase in lobster dry mass. To determine whether the relationship deviated from linearity, a t-test was used to test if the estimated slope b deviated from the expected slope of unity. Before conducting the test above, assumptions of normality and homogeneity of variances were checked and found to be satisfactory [121]. Previous studied in P. argus has calculated fecundity using the wet weight of the embryo and female mass [39]. No previous studies have estimated egg size and reproductive output in this species as well as fecundity using the dry weight of the embryo and females mass, known to provide more accurate estimates [39, 108].
Carcinonemertes sp. in brood masses of Panulirus argus
We noticed the presence of parasitic nemertean worms from the genus Carcinonemertes in five (7.4 %) out of the 68 studied ovigerous females (two lobsters carrying stage I embryos and one each carrying stages II, III, and IV embryos). We examined four out of these five infected lobsters to describe the density of Carcinonemertes as well as the impact that the parasite had on brood mortality and individual-level reproductive parameters (see above). First, we estimated embryo mortality by haphazardly collecting five sub-samples, each comprised 100 eggs from the embryo mass of each female and counting the number of live embryos, dead embryos, and empty capsules (i.e., embryos putatively consumed by the parasite [19]). These embryo samples were then returned to the egg-mass of each female to be included in future fecundity measurements. Embryo mortality was calculated as the average proportion (%) of empty capsules and dead eggs present in our samples. Next, the density of Carcinonemertes sp. was estimated by haphazardly removing five sub-samples of 500 to 900 embryos each from the embryo mass of each infected female and counting the total number of worms in all sub-samples. These embryos were also returned to the egg-mass of each infected female. Density of Carcinonemertes was calculated as the number of worms found per 100 embryos. All examinations and counts were done under a Leica S8AP0 Stereoscope and a Wild M5-97874 dissecting scope.
Abbreviations
ANCOVA, analysis of covariance; ANOVA, analysis of variance; CL, carapace length; RO, reproductive output; SD, standard deviation
References
Clutton-Brock TH. The evolution of parental care. Princeton: Princeton University Press; 1991.
Royle NJ, Smiseth PT, Kolliker M. The evolution of parental care. Cambridge: Oxford University Press; 2012.
Evans JP, Marshall DJ. Male-by-female interactions influence fertilization success and mediate the benefits of polyandry in the sea urchin Heliocidaris erythrogramma. Evolution. 2005;59:106–12.
Neo ML, Vicentuan K, Teo SLM, Erftemeijer PLA, Todd PA. Larval ecology of the fluted giant clam, Tridacna squamosa, and its potential effects on dispersal models. J Exp Mar Biol Ecol. 2015;469:76–82.
Thiel M. Extended parental care in marine amphipods II. Maternal protection of juveniles from predation. J Exp Mar Biol Ecol. 1999;234:235–53.
Thiel M. Extended parental care in crustaceans – an update. Rev Chil Hist Nat. 2003;76:205–18.
Byrne M, Hart MW, Cerra A, Cisternas P. Reproduction and larval morphology of broadcasting and viviparous species in the Cryptasterina species complex. Biol Bull. 2003;205:285–94.
Phillips B, Kittaka J. Spiny lobsters: fisheries and culture 2nd edn. Oxford: Blackwell Science; 2000.
Chaffee C, Strathmann RR. Constraints on egg masses. I. Retarded development within thick egg masses. J Exp Mar Biol Ecol. 1984;84:73–83.
Strathmann RR. Feeding and nonfeeding larval development and life-history evolution in marine invertebrates. Ann Rev Ecol Syst. 1985;16:339–61.
Morritt D, Spicer JI. The physiological ecology of talitrid amphipods: an update. Can J Zool. 1988;76:1965–82.
Ramirez-Llodra E. Fecundity and life-history strategies in marine invertebrates. Adv Mar Biol. 2002;43:87–170.
Fisher TR. Oxygen uptake of the solitary tunicate Styela plicata. Biol Bull. 1976;1976(151):297–305.
Bauer RT. Adaptive modification of appendages for grooming (cleaning; antifouling) and reproduction in the Crustacea. In: Thiel M, Watling L, editors. Functional Morphology of Crustacea, vol. 1. New York: Oxford University Press; 2013. p. 337–75.
Silva P, Luppi TA, Spivak ED. Limb autotomy, epibiosis on embryos, and brooding care in the crab Crytograpsus angulatus (Brachyura: Varunidae). J Mar Biol Ass UK. 2003;83:1015–22.
Silva P, Luppi TA, Spivak ED. Epibiosis on eggs and brooding care in the burrowing crab Chasmagnathus granulatus (Brachyura: Varunidae): comparison between mudflats and salt marshes. J Mar Biol Ass UK. 2007;87:893–901.
Aiken DE, Waddy SL, Uhazy LS. Aspects of the biology of Pseudocarcinonemrtes homari and its association with the American Lobster, Homarus americanus. Can J Fish Aquat Sci. 1985;42:351–6.
Kuris AM. Crustacean Egg Production. Boca Raton: CRC; 1990.
Shields JD. The impact of pathogens on exploited populations of decapod crustaceans. J Invertebr Pathol. 2012;110:211–24.
Baeza JA, Fernández M. Active brood care in Cancer setosus (Crustacea: Decapoda): the relationship between female behavior, embryo oxygen consumption and the cost of brooding. Funct Ecol. 2002;16:241–51.
Prosser CL. Animal models for biomedical-research. Invertebrates – Introduction. Fed Proc. 1973;32:2177–8.
Naylor JK, Taylor EW, Bennett DB. Oxygen uptake of developing eggs of Cancer pagurus (Crustacea: Decapoda: Cancridae) and consequent behavior of the ovigerous female. J Mar Biol Ass UK. 1999;79:305–15.
Fernández M, Bock C, Pörtner HO. The cost of being a caring mother: the ignored factor in the reproduction of marine invertebrates. Ecol Lett. 2000;3:487–94.
Strathmann RR, Strathmann MF. Oxygen supply and limits on aggregation of embryos. J Mar Biol Ass UK. 1995;75:413–28.
Fernández M, Pardo LM, Baeza JA. Patterns of oxygen supply in embryo masses of brachyuran crabs throughout development: the effect of oxygen availability and chemical cues in determining female brooding behavior. Mar Ecol Prog Ser. 2002;245:181–90.
Eriksson SP, Nabbing M, Sjöman E. Is brood care in Nephrops norvegicus during hypoxia adaptive or a waste of energy? Funct Ecol. 2006;20:1097–104.
Förster C, Baeza JA. Active brood care in the anomuran crab Petrolisthes violaceus (Decapoda: Anomura: Porcellanidae): Grooming of brooded embryos by the fifth pereipods. J Crustac Biol. 2001;21:606–15.
Sarvesan R. Some observations on parental care in Octopus dollfusi Robson (Cephalopoda: Octopodidae). J Mar Biol Ass India. 1969;11:203–5.
Dick JT, Faloon SE, Elwood RW. Active brood care in an amphipod: influences of embryonic development, temperature, and oxygen. Anim Behav. 1998;56:663–72.
Burris ZP. Costs of exclusive male parental care in the sea spider Achelia simplissima (Arthropoda: Pycnogonida). Mar Biol. 2011;158:381–90.
Gardner C. Effect of size on reproductive output of giant crabs Pseudocarcinus gigas (Lamarck): Oziidae. Mar Freshw Res. 1997;48:1323–650.
McClain CR, et al. Sizing ocean giants: patterns of intraspecific size variation in marine megafauna. PeerJ. 2015;3, e715.
Heath DJ. Simultaneous hermaphroditism: cost and benefit. J Theor Biol. 1977;64:363–73.
Strathmann RR, Strathmann MF. The relationship between adult size and brooding in marine invertebrates. Am Nat. 1982;119:91–101.
Arundell KL, Wedell N, Dunn AM. The impact of predation risk and of parasitic infection on parental care in brooding crustaceans. Anim Behav. 2014;96:97–105.
Holthuis LB. Marine lobsters of the world. Rome: FAO; 1991.
Carpenter KE, De Angelis N. The living marine resources of the eastern central Atlantic. Rome: FAO; 2014.
Fonseca-Larios ME, Briones-Fourzán P. Fecundity of the spiny lobster Panulirus argus (Latreille, 1804) in the Caribbean coast of Mexico. Bull Mar Sci. 1998;63:21–32.
Bertelsen RD, Matthews TR. Fecundity dynamics of female spiny lobster (Panulirus argus) in a south Florida fishery and Dry Tortugas National Park lobster sanctuary. Mar Freshw Res. 2001;52:1559–65.
Ziegler TA. Larval release rhythms and larval behavior of palinurid lobsters: a comparative study. (Doctoral dissertation). Nicholas School of the Environment and Earth Sciences Duke University. Durham, Duke University; 2007.
Ziegler TA, Forward RB. Control of larval release in the Caribbean spiny lobster, Panulirus argus: role of chemical cues. Mar Biol. 2007;152:589–97.
Ziegler TA, Forward RB. Larval release behaviors in the Caribbean spiny lobster, Panulirus argus: Role of peptide pheromones. J Chem Ecol. 2007;33:1795–805.
Wheatly MG. The provision of oxygen to developing eggs by female shore crabs (Carcinus maenas). J Mar Biol Ass UK. 1981;61:117–28.
Reinsel KA, et al. Egg mass ventilation by caridean shrimp: similarities to other decapods and insight into pheromone receptor location. J Mar Biol Ass UK. 2014;94:1009–17.
Ache BW, Derby CD. Functional organization of olfaction in crustaceans. Trends Neurosci. 1985;8:356–60.
Altner I, Hatt H, Altner H. Structural properties of bimodal chemosensitive and mechanosensitive setae on the pereiopod chelae of the crayfish, Austropotamobius torrentium. Cell Tissue Res. 1983;228:357–74.
Schmidt M, Gnatzy W. Are the funnel-canal organs the campaniform sensilla of the shore crab, Carcinus maenas (Decapoda, Crustacea) 2. Ultrastructure. Cell Tissue Res. 1984;237:81–93.
Ache BW, McClintock TS. The lobster olfactory receptor cell as a neurobiological model: the action of histamine. In: Wiese K, Krenz WD, Tautz J, Reichert H, Mulloney B, editors. Advances in Life Sciences: Frontiers in Crustacean Neurobiology. Basel: Verlag; 1990. p. 33–9.
Schmidt M, Ache BW. Processing of antennular input in the brain of the spiny lobster, Panulirus argus. Non-olfactory chemosensory and mechanosensory pathway of the lateral and median antennular neuropils. J Comp Physiol A. 1996;178:579–604.
Derby CD. Learning from spiny lobsters about chemosensenory coding of mixtures. Physiol Behav. 2000;69:203–9.
Rittschof D. Chemosensation in the daily life of crabs. Am Zool. 1992;32:363–9.
Rittschof D, Forward RB, Mott DD. Larval release in the crab Rhithropanopeus harrisii (Gould) – chemical cues from hatching eggs. Chem Senses. 1985;10:567–77.
Forward RB. Larval release rhythms of decapod crustaceans – an overview. Bull Mar Sci. 1987;41:165–76.
De Vries MC, Forward RB. Control of egg-hatching time in crabs from different tidal heights. J Crustac Biol. 1991;11:29–39.
Rittschof D. Body odors and neutral-basic peptide mimics – a review of responses by marine organisms. Am Zool. 1993;33:487–93.
Rittschof D, Cohen JH. Crustacean peptide and peptide-like pheromones and kairomones. Peptides. 2004;25:1503–16.
Tankersley RA, Bullock TM, Forward RB, Rittschof D. Larval release behaviors in the blue crab Callinectes sapidus: role of chemical cues. J Exp Mar Biol Ecol. 2002;273:1–14.
Fernández M, Brante A. Brood care in brachyuran crabs: the effect of oxygen provision on reproductive costs. Rev Chil Hist Nat. 2003;76:157–68.
Phillips G. Incubation of English prawn Palaemon serratus. J Mar Biol Ass UK. 1971;51:43–8.
Ruiz-Tagle N, Fernández M, Pörtner HO. Full time mothers: daily rhythms in brooding and nonbrooding behaviors of brachyuran crabs. J Exp Mar Biol Ecol. 2002;276:31–47.
Kanciruk P, Herrnkind WF. Preliminary investigation of the daily and seasonal locomotor activity rhythms of the spiny lobster, Panulirus argus. Mar Freshw Behav Phy. 1972;1:351–9.
Lipcius RN, Herrnkind WF. Molt cycle alterations in behavior, feeding, and diel rhythms of a decapod crustacean, the spiny lobster Panulirus argus. Mar Biol. 1982;68:241–52.
Arana E, Dupre M, Gaete M. Ciclo reproductivo, talla de primera madurez sexual y fecundidad de la langosta de Juan Fernandez (Jasus frontalis). Investig Mar. 2000;28:165–74.
Annala JH, Bycrofft BL. Fecundity of the New Zealand red rock lobster Jasus edwardsii. New Zeal J Mar Fresh Res. 1987;21:591–7.
Kagwade PV. Fecundity in the spiny lobster Panulirus polyphagus (Herbst). J Mar Biol Ass India. 1988;30:114–20.
MacDiarmid AB. Size at onset of maturity and size-dependent reproductive output of female and male spiny lobsters Jasus edwardsii (Hutton) (Decapoda, Palinuridae) in northern New Zealand. J Exp Mar Biol Ecol. 1989;127:229–43.
DeMartini EE, Ellis DM, Honda VA. Comparisons of spiny lobster Panulirus marginatus fecundity, egg size, and spawning frequency before and after exploitation. Fish Bull. 1992;91:1–7.
Melville-Smith R, Goosen PC, Stewart TJ. The spiny lobster Jasus lalandii (H. Milne Edwards, 1837) off the South African coast: inter-annual variations in male growth and female fecundity. Crustaceana. 1995;68:174–83.
Hogarth PJ, Barratt LA. Size distribution, maturity and fecundity of the spiny lobster Panulirus penicillatus (Oliver 1791) in the Red Sea. Trop Zool. 1996;9:399–408.
Goñi R, Quetglas A, Reñones O. Size at maturity, fecundity and reproductive potential of a protected population of the spiny lobster Palinurus elephas (Fabricius, 1787) from the western Mediterranean. Mar Biol. 2003;143:583–92.
Green BS, Gardner C, Kennedy RB. Generalized linear modeling of fecundity at length in southern rock lobsters, Jasus edwardsii. Mar Biol. 2009;156:1941–7.
DeMartini EE, DiNardo GT, Williams HA. Temporal changes in population density, fecundity, and egg size of the Hawaiian spiny lobster (Panulirus marginatus) at Necker Bank, Northwestern Hawaiian Islands. Fish Bull. 2003;101:22–31.
Morgan GR. Fecundity in western rock lobster Panulirus longipes-cygnus (George) (Crustacea, Decapoda, Palinuridae). Aust J Mar Fresh Res. 1972;23:133–41.
MacFarlane JW, Moore R. Reproduction of the ornate rock lobster Panulirus ornatus (Fabricius), in Papua New Guinea. Aust J Mar Freshw Res. 1986;37:55–65.
Pérez-González R, Puga-Lopez D, Castro-Longoria R. Ovarian development and size at sexual maturity of the Mexican spiny lobster Panulirus inflatus. New Zeal J Mar Freshw Res. 2009;43:163–72.
Groenveld JC. Fecundity of spiny lobster Palinurus gilchristi (Decapoda: Palinuridae) off South Africa. Af J Mar Sci. 2005;27:231–8.
Groeneveld JC, Greengrass CL, Branch GM, McCue SA. Fecundity of the deep-water spiny lobster Palinurus delagoae. W I O J Mar Sci. 2005;4:135–43.
Gracia GA. Seasonal variation of the fecundity of the lobster Panulirus inflatus (Bouvier, 1895) (Crustacea: Decapoda: Palinuridae). Cienc. 1985;11:7–27.
Pérez-González R, Valadez LM, Rodríguez-Domínguez G, Aragón-Noriega EA. Seasonal variation in brood size of the spiny lobster Panulirus gracilis (Decapoda: Palinuridae) in Mexican waters of the Gulf of California. J Shellfish Res. 2012;31:935–40.
Torres P, Penha-Lopez G, Narciso L, Macia A, Paula J. Fecundity and brood loss in four species of fiddler crabs, genus Uca (Brachyura: Ocypodidae), in the mangroves of Inhaca Island, Mozambique. J Mar Biol Assoc UK. 2009;89:371–8.
Oh CW, Hartnoll RG. Brood loss during incubation in Philocheras trispinosus (Decapoda) in Port Erin Bay, Isle of Man. J Crustac Biol. 1999;19:467–76.
Einum S, Hendry AP, Fleming IA. Egg-size evolution in aquatic environments: does oxygen availability constrain size? Proc Roy Soc B Biol Sci. 2002;269:2325–30.
Fernández M, Calderón R, Cifuentes M, Pappalardo P. Brooding behaviors and cost of brooding in small body size brachyuran crabs. Mar Ecol Prog Ser. 2006;309:213–20.
Hines AH. Allometric constraints and variables of reproductive effort in brachyuran crabs. Mar Biol. 1982;69:309–20.
Hines AH. Constraint on reproductive output in brachyuran crabs: Pinnotherids test the rule. Am Zool. 1992;32:503–11.
Clarke A. Temperature, latitude, and reproductive effort. Mar Ecol Prog Ser. 1987;38:89–99.
Anger K, Moreira GS. Morphometric and reproduction traits of tropical caridean shrimps. J Crustac Biol. 1998;18:823–38.
Anderson JR, Spadaro AJ, Baeza JA, Behringer DC. Ontogenetic shifts in resource allocation: colour change and allometric growth of defensive and reproductive structures in the Caribbean spiny lobster Panulirus argus. Biol J Linn Soc. 2013;108:87–98.
Wickham DE. Carcinomertes errans and the fouling and mortality of eggs of the Dungeness crab, Cancer magister. J Fish Res Board Can. 1979;36:1319–24.
Wickham DE. Epizootic infestation by nemertean brood parasites on commercially important crustaceans. Can J Fish Aquat Sci. 1986;43:2295–302.
Shields JD, Kuris AM. Temporal variation in abundance of the egg predator Carcinonemertes epialti (Nemertea) and its effect on egg mortality of its host, the shore crab, Hemigrapsus oregonensis. Hydrobiologia. 1988;156:31–8.
Shields JD. Diseases of spiny lobsters: a review. J Invertebr Pathol. 2011;106:79–91.
Okazaki RK, Wehrtmann IS. Preliminary survey of a nemertean crab egg predator, Carcinonemertes, on its host crab, Callinectes arcuatus (Decapoda, Portunidae) from Golfo de Nicoya, Pacific Costa Rica. ZooKeys. 2014;457:367–75.
Santos C, Bueno SLS, Norenburg JL. Infestation by Carcinonemertes divae (Nemertea: Carcinonemertidae) in Libinia spinosa (Decapoda: Pisidae) from São Sebastião Island, SP, Brazil. J Nat Hist. 2006;40:999–1005.
Sadeghian PS, Kuris AM. Distribution and abundance of a nemertean egg predator (Carcinonmertes sp.) on a leucosiid crab, Randallia ornate. Hydrobiologia. 2001;456:59–63.
Shields JD, Kuris AM. Carcinonemertes wickhami n. sp. (Nemertea), a symbiotic egg predator from the spiny lobster Panulirus interruptus in Southern California, with remarks on symbiont-host adaptations. Fish Bull. 1990;88:279–87.
Shields JD, Behringer DC. A new pathogenic virus in the Caribbean spiny lobster Panulirus argus from the Florida Keys. Dis Aquat Org. 2004;59:109–18.
Lee CE, Strathmann RR. Scaling of gelatinous clutches: Effects of siblings’ competition for oxygen on clutch size and parental investment per offspring. Am Nat. 1998;151:293–310.
Tavares M. Lobsters. In: Carpenter KE, De Angelis N, editors. The living marine resources of the eastern central Atlantic. Rome: FAO; 2014. p. 294–310.
Cruz R, Bertelsen RD. The spiny lobster (Panulirus argus) in the wider Caribbean: A review of life cycle dynamics and implications for responsible fisheries management. Proc 61st Gulf Caribbean Fish Inst. 2008;61:10–4.
Lipcius RN, Herrnkind WF. Photoperiodic regulation and daily timing of spiny lobster mating behavior. J Exp Mar Biol Ecol. 1987;89:191–204.
Butler MJ, et al. Patterns of spiny lobster (Panulirus argus) postlarval recruitment in the Caribbean: a CRTR Project. Proc 62nd Gulf Caribbean Fish Inst. 2010;62:361–9.
Butler MJ, Bertelsen R, MacDiarmid A. Mate choice in temperate and tropical spiny lobsters with contrasting reproductive systems. ICES J Mar Sci. 2015;72 Suppl 1:i101–14.
Lipcius RN, Edwards ML, Herrnkind WF, Waterman SA. In situ mating behaviour of the spiny lobster Panulirus argus. J Crustac Biol. 1983;3:217–22.
MacDiarmid AB, Butler MJ. Sperm economy and limitation in spiny lobsters. Behav Ecol Sociobiol. 1999;46:14–24.
Butler MJ, MacDiarmid A, Gnanalingam G. The effect of parental size on spermatophore production, egg quality, fertilization success, and larval characteristics in the Caribbean spiny lobster, Panulirus argus. ICES J Mar Sci. 2015;72 Suppl 1:i115–23.
Butler MJ, Heisig-Mitchell J, MacDiarmid AB, Swanson RJ. The effect of male size and spermatophore characteristics on reproduction in the Caribbean spiny lobster, Panulirus argus. New Front Crustac Biol. 2011;15:69–84.
Saul S. A review of the literature and life history study of the Caribbean spiny lobster, Panulirus argus. Caribbean Southeast Data Assessment Review Workshop Report, SEDAR-DW-05. Sustainable Fisheries Division Contribution No. SFD- 2004–048. North Charleston: SouthEast Data, Assessment, and Review; 2004.
Bertelsen RD, Hornbeck J. Using acoustic tagging to determine adult spiny lobster (Panulirus argus) movement patterns in the Western Sambo Ecological Reserve (Florida, United States). New Zeal J Mar Freshw Res. 2009;43:35–46.
Bertelsen RD. Characterizing daily movements, nomadic movements, and reproductive migrations of Panulirus argus around the Western Sambo Ecological Reserve (Florida, USA) using acoustic telemetry. Fish Res. 2013;144:91–102.
Maxwell KE, Matthews TR, Bertelsen RD, Derby CD. Using age to evaluate reproduction in Caribbean spiny lobster, Panulirus argus, in the Florida Keys and Dry Tortugas, United States. New Zeal J Mar Freshw Res. 2009;43:139–49.
Matthews TR, Maxwell KE, Bertelsen RD, Derby CD. Use of neurolipofuscin to determine age structure and growth rates of Caribbean spiny lobster Panulirus argus in Florida, United States. New Zeal J Mar Fresh. 2009;43:125–37.
Cox C, Hunt JH. Change in size and abundance of Caribbean spiny lobsters Panulirus argus in a marine reserve in the Florida Keys National Marine Sanctuary, USA. Mar Eco Prog Ser. 2005;294:227–39.
Maxwell KE, Matthews TR, Bertelsen RD, Derby CD. Age and size structure of Caribbean spiny lobster, Panulirus argus, in a no-take marine reserve in the Florida Keys, USA. Fish Res. 2013;144:84–90.
Birkeland C, Dayton PK. The importance in fishery management of leaving the big ones. Trends Ecol Evol. 2005;20:356–8.
Martin P, Bateson P. Measuring Behaviour: An Introductory Guide. Cambridge: Cambridge University Press; 1986.
JMP Pro, Version 10, SAS Institute Inc., Cary, N.C., 1989–2007
Turner RL, Lawrence JM. In: Stancyk SE, editor. Volume and composition of echinoderm eggs: implications for the use of egg size in life history models. Columbia: University of South Carolina Press; 1979. p. 25–40.
Baeza JA. Testing three models on the adaptive significance of protandric simultaneous hermaphroditism in marine shrimp. Evolution. 2006;60:1840–50.
Hartnoll RG. Growth. In: Abele LG, editor. The Biology of Crustacea 2. New York: Academic; 1982. p. 111–96.
Zar JH. Biostatistical analysis. Upper Saddle River: Prentice-Hall; 1996.
Acknowledgements
We sincerely thank Kylie Smith and Cindy Lewis for logistic and material assistance that helped make possible this study. We also appreciate the research opportunity provided by the Clemson University Creative Inquiry program to Mr. Dallas Owen who gained research experience at the Baeza-LAB during the summer, 2015 field season. This is Smithsonian Marine Station at Fort Pierce contribution number 1040.
Funding
Funding provided by Clemson University to JAB.
Availability of data and material
All datasets on which the conclusions of the manuscript rely are presented in the main paper.
Authors’ contribution
Conceived and designed the experiments: JAB LJA. Performed the experiments: JAB LJA LS NM RG. Analyzed the data: JAB LJA. Contributed reagents/materials/analysis tools: JAB. Wrote the paper: JAB LJA MC. All authors read and approved the final manuscript.
Authors’ information
JAB is an ecologist specialized in evolutionary, behavioral and molecular ecology. JAB’s research goals focus on testing sex allocation and mating systems theories using marine invertebrates (mostly crustaceans) as model systems. He is also interested in understanding the role of the environment in determining the evolution of social behaviors and other behavioral traits (i.e., alternative mating tactics, territoriality, and symbiosis). Currently, JAB is an assistant professor at the Department of Biological Sciences, Clemson University, USA, and an associate researcher at the Smithsonian Marine Station, Fort Pierce, Florida, USA. He has published more than 80 original papers on the behavioral ecology and life history of crustaceans.
Competing interests
The authors declare that they have no competing interests.
Consent for publication
Non applicable.
Ethics approval and consent to participate
No approval by an ethical committee was required to accomplish the goals of the present study because experimental work was conducted with an unregulated marine invertebrate. Florida Fish and Wildlife Conservation Commission gently granted permission for sampling lobster specimens from the wild.
Author information
Authors and Affiliations
Corresponding author
Additional file
Active parental care in the Caribbean spiny lobster Panulirus argus. (AVI 14 kb)
Rights and permissions
Open Access This article is distributed under the terms of the Creative Commons Attribution 4.0 International License (http://creativecommons.org/licenses/by/4.0/), which permits unrestricted use, distribution, and reproduction in any medium, provided you give appropriate credit to the original author(s) and the source, provide a link to the Creative Commons license, and indicate if changes were made. The Creative Commons Public Domain Dedication waiver (http://creativecommons.org/publicdomain/zero/1.0/) applies to the data made available in this article, unless otherwise stated.
About this article
Cite this article
Baeza, J.A., Simpson, L., Ambrosio, L.J. et al. Active parental care, reproductive performance, and a novel egg predator affecting reproductive investment in the Caribbean spiny lobster Panulirus argus . BMC Zool 1, 6 (2016). https://doi.org/10.1186/s40850-016-0006-6
Received:
Accepted:
Published:
DOI: https://doi.org/10.1186/s40850-016-0006-6