Abstract
Background
Even an ultraprotective ventilation strategy in severe acute respiratory distress syndrome (ARDS) patients treated with extracorporeal membrane oxygenation (ECMO) might induce ventilator-induced lung injury and apneic ventilation with the sole application of positive end-expiratory pressure may, therefore, be an alternative ventilation strategy. We, therefore, compared the effects of ultraprotective ventilation with apneic ventilation on oxygenation, oxygen delivery, respiratory system mechanics, hemodynamics, strain, air distribution and recruitment of the lung parenchyma in ARDS patients with ECMO.
Methods
In a prospective, monocentric physiological study, 24 patients with severe ARDS managed with ECMO were ventilated using ultraprotective ventilation (tidal volume 3 ml/kg of predicted body weight) with a fraction of inspired oxygen (FiO2) of 21%, 50% and 90%. Patients were then treated with apneic ventilation with analogous FiO2. The primary endpoint was the effect of the ventilation strategy on oxygenation and oxygen delivery. The secondary endpoints were mechanical power, stress, regional air distribution, lung recruitment and the resulting strain, evaluated by chest computed tomography, associated with the application of PEEP (apneic ventilation) and/or low VT (ultraprotective ventilation).
Results
Protective ventilation, compared to apneic ventilation, improved oxygenation (arterial partial pressure of oxygen, p < 0.001 with FiO2 of 50% and 90%) and reduced cardiac output. Both ventilation strategies preserved oxygen delivery independent of the FiO2. Protective ventilation increased driving pressure, stress, strain, mechanical power, as well as induced additional recruitment in the non-dependent lung compared to apneic ventilation.
Conclusions
In patients with severe ARDS managed with ECMO, ultraprotective ventilation compared to apneic ventilation improved oxygenation, but increased stress, strain, and mechanical power. Apneic ventilation might be considered as one of the options in the initial phase of ECMO treatment in severe ARDS patients to facilitate lung rest and prevent ventilator-induced lung injury.
Trial registration: German Clinical Trials Register (DRKS00013967). Registered 02/09/2018. https://www.drks.de/drks_web/navigate.do?navigationId=trial.HTML&TRIAL_ID=DRKS00013967.
Similar content being viewed by others
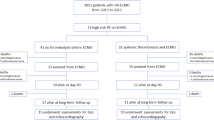
Background
Acute respiratory distress syndrome (ARDS) is a severe lung dysfunction due to inflammation, edema formation and pulmonary shunt resulting in hypoxia and often necessitating invasive mechanical ventilation (MV) [1]. In the most severe cases of ARDS with refractory hypoxemia, extracorporeal membrane oxygenation (ECMO) has been proposed as a viable therapy to maintain oxygenation and oxygen delivery (DO2) [2], possibly improving survival [3]. Although the use of ECMO in patients with ARDS is increasing [4], there is a paucity of physiological data regarding the optimal ventilator settings during ECMO treatment [5]. The Extracorporeal Life Support Organization (ELSO) recommends a ventilation strategy with low respiratory rates (RR), low inspiratory plateau pressure (Pplat) and moderate levels of positive end-expiratory pressure (PEEP) to limit ventilator-induced lung injury (VILI) [6]. Since the main principle of MV during ECMO is to protect the lung, gas exchange should primarily be managed with ECMO [6].
MV during ECMO, even with ultraprotective tidal volume (VT), may induce VILI due to the transmitted energy by the ventilator resulting in increased stress (transpulmonary pressure at end-inspiration) and strain (VT/end-expiratory lung volume) [7, 8] as well as high fractions of inspired oxygen (FiO2) [9] in the inflamed pulmonary parenchyma.
As the optimal ventilation strategy in ECMO patients is insufficiently defined [10], the primary endpoint of this physiological, short-term study was to investigate the effects of ultraprotective compared to apneic ventilation on oxygenation and DO2 in ARDS patients treated with ECMO in the initial phase of the management. We further hypothesized whether apneic ventilation with the sole application of a low FiO2 and reasonable PEEP reduces cyclic lung volume changes and may better protect the lung parenchyma from VILI. Therefore, the secondary endpoints were to evaluate mechanical power, stress, regional air distribution, recruitment and the resulting strain associated with the application of PEEP (apneic ventilation) and/or low VT (ultraprotective ventilation).
Methods
The study was approved by the local ethics committee (Medizinische Ethikkommission II, University Medical Centre Mannheim, Medical Faculty Mannheim of the University of Heidelberg, Mannheim, registration number 2016-601 N-MA) and registered at the German Clinical Trials Register (DRKS00013967). After obtaining written informed consent we collected prospective data from 24 patients with severe ARDS managed with ECMO admitted to the Department of Anesthesiology and Critical Care Medicine, University Medical Centre Mannheim, Medical Faculty Mannheim of the University of Heidelberg in Mannheim, Germany. Detailed inclusion and exclusion criteria, as well as criteria for discontinuation of the study are provided in Additional file 1.
ECMO therapy was initiated as indicated by the attending physician in accordance with the current ELSO guidelines [6]. SAPS II [11], SOFA [12] and APACHE II scores [13] were calculated for each patient at ICU admission. The RESP [14] and PRESERVE scores [15] were calculated immediately before ECMO cannulation. Vascular access for the extracorporeal circuit was established with a 29 French drainage cannula (HLS Cannula, Maquet, Rastatt, Germany) in the femoral vein and a 21 or 23 French return cannula (HLS Cannula, Maquet, Rastatt, Germany) in the internal jugular vein. The ECMO circuit was driven by a magnetically levitated rotor pump (Centrimag Circulatory Support System, Abbot GmbH, Wiesbaden, Germany) and completed with a gas exchange membrane (PLS System, Maquet, Rastatt, Germany). Patients were sedated (Richmond Agitation-Sedation Score of − 5) and paralyzed throughout each measurement [16]. Norepinephrine was used if mean arterial pressure (MAP) was below 65 mmHg despite sufficient intravascular volume. Dobutamine was used if cardiac index was below 2.0 l/min/m2 despite sufficient cardiac pre- and afterload. Extracorporeal blood flow was titrated to achieve an arterial partial pressure of oxygen (PaO2) of at least 60 mmHg with ventilation settings (Engström Carescape™, GE Healthcare, Munich, Germany) chosen by the attending physician. ECMO gas flow was titrated to achieve an arterial pH of 7.35 to 7.45. For the measurement of esophageal pressure, an esophageal balloon catheter (NutriVent™, Sidam Biomedical Solutions, Mirandola, Italy) was inserted, filled with 2.5 ml of air as indicated by the manufacturer and connected to the ventilator. Catheter position was confirmed in all patients as previously described [17]. Airway and esophageal pressures were recorded during end-expiratory and end-inspiratory hold (5 s without gas flow from the ventilator), respectively [18]. Cardiac output (CO) was measured using a thermodilution catheter (4F/5F Pulsiocath™, Pulsion Medical Systems, Feldkirchen, Germany) and a CO monitor (PiCCOplus™, Pulsion Medical Systems, Munich, Germany). For intermittent blood gas analyses for the measurement of pH, the PaO2 and carbon dioxide (PaCO2), the arterial oxygen saturation (SaO2) and hemoglobin (Hb) a blood gas analyzer (Radiometer ABL 800 FlexQ, Radiometer GmbH, Krefeld, Germany) was used.
Study protocol
After ensuring adequate analgosedation and neuromuscular blockade, dynamic recruitment was performed by slowly increasing the PEEP set by the attending physician from the individual baseline to 35 cmH2O over a time period of 5 min. The difference between end-inspiratory and end-expiratory airway pressure was kept at 15 cmH2O in a pressure-controlled ventilation mode. After 2 min of dynamic recruitment at a Pplat of 50 cm H2O, PEEP was empirically set to 30 cm H2O and a standardized ventilation setting (ultraprotective ventilation, RR of 12/min, VT of 3 ml/kg IBW, inspiration-to-expiration ratio of 1:1) established. PEEP was then decreased stepwise by 2 cm H2O and after a 10-min equilibration period we calculated the static elastance of the respiratory system (Estat,RS) as driving pressure (Pdriv)/VT. The decremental PEEP trial was stopped if Estat,RS did not progressively decrease because of the reduction of PEEP. For an unequivocal identification of the lowest achievable individual Estat,RS of the patient, a further reduction of PEEP had to result in a marked increase in Estat,RS. Subsequently, another recruitment maneuver was performed, and PEEP was set to the lowest Estat,RS [18]. Without further modifications to the established ventilation settings, the fraction of inspired oxygen was adjusted to 21%. After another 30-min equilibration period respiratory mechanic and hemodynamic parameters were assessed. Blood gas samples from an arterial catheter were analyzed. These measurements were then repeated with a FiO2 of 50% and 90% with a 30-min equilibration period between each measurement. Subsequently, the ventilation mode was changed from ultraprotective ventilation to apneic ventilation without any changes in PEEP and the measurements were repeated at a FiO2 of 21%, 50% and 90%, respectively. During each measurement respiratory mechanic and hemodynamic parameters were assessed. Blood gas samples from an arterial catheter were analyzed. Neither ECMO blood nor gas flow were changed during the study period. Further details of the ventilator management are provided in the Additional file 1. Ventilation mode was then again changed to ultraprotective ventilation with a RR of 12/min, a VT of 3 ml/kg IBW, an inspiration-to-expiration ratio of 1:1 and unchanged PEEP and the patients transferred to the CT scanner. Images of the whole lungs were acquired at end-inspiratory hold, at end-expiratory hold at PEEP as well as at a PEEP of 0 mmHg (ZEEP). We used a second-generation dual source CT scanner (Somatom Definition Flash) with 32 × 0.6 mm collimation, 89/76 reference mAs at 120 kV, a pitch of 0.8 and 0.5 s rotation time. CT scans were first segmented automatically with the Medical Imaging Interaction Toolkit (Version 2018.04.2, https://www.mitk.org/). The resulting segmentations were then used as a basis for manual segmentation to differentiate nonaerated lung and extrapulmonary soft tissue. Additional file 1: Fig. S1 shows the schematic flow chart of the study design.
Calculations
Gas exchange, respiratory system mechanics and hemodynamics were calculated after an equilibration period for each ventilation strategy and fraction of inspired oxygen (see Additional file 1). Lung parenchyma aeration was classified following the conventional thresholds [19] in the CT scans and segmented into a non-dependent and dependent compartment along a horizontal plane through the tracheal bifurcation using in-house software (details are provided in Additional file 1). Recruitment due to PEEP and VT as well as static and dynamic strain were calculated as described in Additional file 1.
Statistical analysis
The sample size calculation for testing the primary hypothesis (PaO2 would be decreased in apneic ventilation compared to ultraprotective ventilation with a FiO2 of 50%) was based on data obtained from 5 patients, not included in the study. Accordingly, we expected that a sample size of 24 would provide the appropriate power (1 – β = 0.9) to identify significant (α = 0.05) differences considering a partial η2 of 0.11 and an effect size of 0.35 analyzed with a repeated measurement ANOVA. Power analysis was performed with G*Power 3.1.9.7. For continuous variables, the normality of the data and the homogeneity of variances were tested by means of the Shapiro–Wilk test and Levene’s median test, respectively. As per the study protocol, longitudinal physiological and CT data were analyzed using repeated measures ANOVA followed by Holm–Sidak’s post-hoc test or the Friedman procedure as appropriate to control for variability between patients. Single timepoint data were analyzed using one-way ANOVA followed by Holm–Sidak’s post-hoc test or Mann–Whitney test, as appropriate. The results are expressed as mean ± standard deviation. The level of significance was set at p < 0.05. Statistical analysis was performed using SigmaPlot 12.5 (Systat Software GmbH, Erkrath, Germany).
Results
Patient characteristics
Twenty-four patients with severe ARDS treated with veno-venous ECMO completed the study and were included in the analysis. Table 1 shows the demographic and clinical characteristics of the patients. Nineteen patients had a primary pulmonary, while five patients had an extrapulmonary cause of ARDS. At study inclusion, patients were receiving MV for a mean duration of 5.7 ± 3.5 days. The mean duration of ECMO support in the study cohort was 13.5 ± 6.0 days with an ICU mortality of 42%.
Effect on oxygenation and oxygen delivery
SaO2 significantly increased from a FiO2 of 21% to 90% in ultraprotective ventilation and at every FiO2 step in apneic ventilation and was uniformly higher in ultraprotective ventilation than in apneic ventilation at each FiO2 (Fig. 1A). PaO2 increased at each FiO2 step in ultraprotective ventilation and from 21 to 90% and 50% to 90% in apneic ventilation. PaO2 differed significantly between ultraprotective and apneic ventilation only when applying a FiO2 of 50% and 90% (Fig. 1B). CO was lower in ultraprotective compared to apneic ventilation (Fig. 1C). DO2 was higher for apneic ventilation with a FiO2 of 90%, compared to the corresponding ultraprotective ventilation (Fig. 1D).
Oxygenation and oxygen delivery for ultraprotective and apneic ventilation and three different fractions of inspired oxygen. A Arterial oxygen saturation; SaO2, arterial oxygen saturation, FiO2, fraction of inspired oxygen. B Arterial partial pressure of oxygen. PaO2, arterial partial pressure of oxygen, FiO2, fraction of inspired oxygen. C Cardiac output, FiO2, fraction of inspired oxygen. D Oxygen delivery. FiO2, fraction of inspired oxygen, brackets denote statistically significant differences between ventilation strategies, p-values are shown above the brackets. a: p < 0.05 ultraprotective ventilation with a FiO2 of 21% vs. ultraprotective ventilation with a FiO2 of 50%; b: p < 0.05 ultraprotective ventilation with a FiO2 of 21% vs. ultraprotective ventilation with a FiO2 of 90%; c: p < 0.05 ultraprotective ventilation with a FiO2 of 50% vs. ultraprotective ventilation; with a FiO2 of 90%; d: p < 0.05 apneic ventilation with a FiO2 of 21% vs. apneic ventilation with a FiO2 of 50%; e: p < 0.05 apneic ventilation with a FiO2 of 21% vs. apneic ventilation with a FiO2 of 90%; f: p < 0.05 apneic ventilation with a FiO2 of 50% vs. apneic ventilation with a FiO2 of 90%
Effect on lung mechanics, hemodynamics and carbon dioxide elimination
Since lung mechanics, hemodynamics and carbon dioxide elimination did not differ between a FiO2 of 21%, 50% and 90%, we opted to present the pooled data during ultraprotective and apneic ventilation in Table 2.
Pplat, Pmean, Pdriv, stress and mechanical power were more reduced in apneic compared to ultraprotective ventilation with no changes in PEEP level. pH was lower during apneic ventilation compared to ultraprotective ventilation due to increased PaCO2.
Air distribution in lung parenchyma, percentage of lung recruitment and strain
Figure 2 shows the air distribution in the lung parenchyma based on chest CT-scan analysis. Aerated lung volume increased with PEEP and VT. In the non-dependent lung segments as well as in the whole lung, application of PEEP significantly reduced the non-aerated lung volume compared to ZEEP. No additional reduction of non-aerated lung volume was obtained by the application of VT. The application of PEEP significantly increased the amount of normally aerated lung parenchyma compared to ZEEP in all lung segments. VT further increased the normally aerated lung volume compared to PEEP (Fig. 2A). PEEP and VT recruited significantly more lung volume in the non-dependent compared to the dependent part of the lung (Fig. 2B). Static strain was significantly higher in the non-dependent than in the dependent lung segments (Fig. 2C).
Air distribution in lung parenchyma, percentage of lung recruitment and strain in the non-dependent, dependent, and whole lung. A Air distribution in lung parenchyma in in the non-dependent, dependent, and whole lung. Black, non-aerated lung volume (− 100 to + 100 hounsfield units); dark grey, poorly inflated lung volume (− 500 to − 100 hounsfield units); light grey, normally inflated lung volume (− 501 to − 900 hounsfield units); white, overinflated lung volume (< − 900 hounsfield units), ZEEP, air distribution at zero end-expiratory pressure; PEEP, air distribution at end-expiratory hold, INSP, air distribution at end-inspiratory hold. B Percentage of recruitment associated to the application of apneic and ultraprotective ventilation in the non-dependent, dependent and whole lung. PEEP, recruitment due to positive end-expiratory pressure from zero positive end-expiratory pressure (ZEEP); tidal volume; recruitment due to tidal volume from end-expiratory pressure. C Static and dynamic strain in the non-dependent, dependent and whole lung. Static, static strain due to the application of PEEP; dynamic, dynamic strain due to the application of tidal volume. Brackets denote statistically significant differences, p values are shown above the brackets. a: p < 0.05 PEEP vs. ZEEP. b p < 0.05 INSP vs. PEEP
Discussion
In patients with severe ARDS during ECMO treatment, we investigated the effects of VT, PEEP, and FiO2 on oxygenation as well as DO2 during an ultraprotective and an apneic ventilation strategy. As the transmitted energy by the ventilator to the lungs may be of critical importance to determine VILI, we also measured Pdriv, strain, stress and mechanical power to relate the physiological "cost" of the ventilation strategy to the potential benefit regarding oxygenation and DO2. We found that: (1) ultraprotective ventilation increased PaO2 and SaO2 but reduced CO compared to apneic ventilation; (2) both ventilation strategies preserved pH, PaCO2 and DO2, independently of the FiO2 without any changes in ECMO blood or gas flow; (3) VT of 3 ml/kg IBW increased Pdriv, stress, strain and mechanical power compared to apneic ventilation; (4) PEEP as well as VT recruited lung volume primarily in the non-dependent lung.
Oxygenation and oxygen delivery
The application of an ultraprotective VT resulted in higher SaO2 and PaO2 compared to the application of PEEP alone with an increase similar to that obtained with higher FiO2 in apneic ventilation (Fig. 1A, B). This may be the result of the higher airway pressures inducing parenchymal recruitment as the ultraprotective ventilation strategy increased the aerated lung volume in comparison to apneic ventilation (Fig. 2A). However, ultraprotective ventilation resulted in a significantly decreased CO, possibly due to the increased airway pressures compared to apneic ventilation (Fig. 1C). In severe ARDS, high VT and airway pressures may affect CO by decreasing systemic venous return and increasing right ventricular outflow impedance [20]. As CVP does not significantly increase, our data implies, that in patients with most severe ARDS even a VT of 3 ml/kg IBW and the resulting Pplat and Pdriv negatively affects right ventricular afterload and thus CO. Although the apneic ventilation strategy preserved CO and thus DO2 compared to ultraprotective ventilation, we observed a significant decrease in SaO2 (Fig. 1D) especially at FiO2 of 21%, presumably because of the lower Pmean resulting in less aerated lung parenchyma [21]. On the other hand, as long as hemoglobin content and CO remain adequate, SaO2 levels as low as 80% might be physiologically acceptable [22]. Of note, as the ratio between ECMO flow and CO decreased in apneic ventilation compared to ultraprotective ventilation, the improvement in arterial oxygenation may not only be the result of the pulmonary recruitment but rather a result of the altered hemodynamics [22]. A key component of ECMO treatment is to increase or preserve DO2 [6, 22]. The safe threshold of DO2 for the individual patient is unknown, but a range between 400 and 600 ml/min/m2 is probably reasonable [23, 24] in critical ill patients. Therefore, both ventilation strategies appear to be viable in patients treated with ECMO. Because a further increase of DO2 due to higher VT and concomitant tidal recruitment is not beneficial [24], using the ventilation strategy with the lowest energy transmission on lung parenchyma may be favorable to improve outcomes in these patients [7, 25].
Respiratory system mechanics
Limiting VT to approximately 6 ml/kg IBW and thus cyclic end-inspiratory hyperinflation of the inhomogeneous lung has been shown to reduce mortality and is recommended in all current guidelines for the management of ARDS [26, 27]. On the other hand, there is a subgroup of patients with most severe ARDS characterized by low end-expiratory lung volume and low recruitability of atelectatic lung parenchyma, that show tidal hyperinflation in spite of low VT [28]. ECMO as well as extracorporeal carbon dioxide elimination may facilitate even lower VT ventilation in these patients [29, 30]. Most medium-to-high-volume centers currently limit VT and airway pressure in patients managed with ECMO [5] as “lung rest” to prevent VILI [31]. On the other hand, only 27% of these centers have an explicit MV protocol [31], which may be due to the fact that there is a paucity of physiological data describing the immediate physiological effects of different ventilation strategies during ECMO. In an international multicenter prospective cohort, Schmidt et al. reported a rather moderate reduction of VT from 6.4 ± 2.0 to 3.7 ± 2.0 ml, of the RR from 26 ± 8 to 14 ± 6 breaths per minute and of Pdriv from 20 ± 7 to 14 ± 4 cm H2O after the initiation of ECMO [5]. Of note, none of these centers utilized apneic ventilation as a first line ventilation strategy. As shown in the present study, ultraprotective ventilation strategy using even lower VT (tidal volume 3 ml/kg ideal body weight) and RR induced a considerable Pdriv, which has been independently linked with mortality in ARDS patients during ECMO support [7]. In a recent study in ARDS patients treated with ECMO, Del Sorbo et al. demonstrated a linear relationship between inspiratory pressures, the resulting mechanical power and biomarkers of systemic inflammation [32]. Despite using an ultraprotective VT of 2.4 ml/kg, there was substantial risk for biotrauma and ultimately VILI in patients with low respiratory system compliance. The authors concluded that the use of apneic ventilation may be favorable in these patients. Rozencwajg et al. tested three different “ultraprotective” strategies of MV with reduced plateau and driving pressure and consecutively VT. They found a significantly limited pulmonary biotrauma irrespectively of the tested strategy [33]. This is in accordance with the results of a large animal study were ventilation with limited driving pressure, low respiratory rate and thus limited mechanical power decreased lung injury in comparison with a ventilation strategy with higher driving pressures and higher minute ventilation [34].
Air distribution, recruitment and strain
Locoregional excess of overinflation and alveolar cycling in inhomogeneous lung parenchyma are considered as pivotal factors causing VILI and are quantified by Pdriv, stress, strain and transmitted mechanical power [8]. Subsequently, a ventilation strategy for patients with most severe ARDS treated with ECMO should minimize these variables and simultaneously preserve cardiopulmonary function [10]. Therefore, the most “protective” ventilation strategy at least in theory should provide total "lung rest" by the sole application of an adequate level of PEEP, while carbon dioxide is removed through the ECMO membrane [35]. As higher PEEP levels during the first 3 days on ECMO support were independently associated with improved survival [36], the strategies to optimize PEEP warrant careful consideration. In our study, the application of PEEP titrated to the lowest Estat,RS was associated with a reduced atelectatic lung volume in the non-dependent lung and increased aerated lung volume in both lung regions compared to ZEEP (Fig. 2A). On the other hand, this PEEP titration strategy was not able to recruit dependent lung segments, as shown in Fig. 2B. As shown previously PEEP titrated to the lowest Estat,RS might be associated with negative end-expiratory transpulmonary pressure [18]. Aiming for a positive end-expiratory transpulmonary pressure might, therefore, be a viable strategy to further reduce atelectatic lung volume especially in dependent lung regions to reduce cyclic opening and closing of the alveoli (atelectrauma), to homogenize VT distribution and to avoid overdistension [37]. The VT of the ultraprotective ventilation strategy causes additional dynamic strain compared to the static strain induced by PEEP in the inflamed inhomogeneous lung. This resulted in a total mechanical power of approximately 13 J/min transmitted to the lung despite using a ventilation strategy with lower Pdriv than described in two recent studies comparing MV during ECMO [10, 38]. Dynamic strain has been shown to be a more potent inductor of VILI compared to static strain resulting in pulmonary inflammation, edema formation and an increased mortality [39]. It is debatable whether a further reduction of VT or the prevention of dynamic strain altogether would be more beneficial for the patient as the short- and long-term biological consequences of dynamic strain are incompletely understood. Of note, a recent trial by McNamee et al. found no statistically significant reduction in mortality when utilizing extracorporeal carbon dioxide removal devices to reduce VT [40].
On the other hand, there is a paucity of data regarding the long-term consequences of omitting dynamic strain using an apneic ventilation strategy for a prolonged period of time [32].
Clinical implications
Our data suggests that apneic ventilation might be a viable option in the initial phase of ECMO treatment in severe ARDS. Apneic ventilation preserved DO2 and reduced stress, strain and mechanical power transmitted to the lung compared with ultraprotective ventilation. According to current recommendations and clinical practice in medium-to-high-volume ECMO centers, the goal of ECMO treatment is to increase or preserve DO2 while minimizing VILI and prevent iatrogenic harm, especially in the initial phase of ARDS, while lung parenchyma is most vulnerable [5, 6, 31].
With resolving lung dysfunction and hemodynamic stabilization, spontaneous breathing should be considered to prevent diaphragmatic dysfunction [41] and allow ECMO weaning [6]. However, limited data is available regarding the benefits of spontaneous breathing in ARDS patients treated with ECMO and the potential self-inflicted lung injury [25]. Patients in the early phase of ARDS, characterized by low respiratory system compliance and low end-expiratory lung volume, are particularly susceptible to injurious transpulmonary pressure swings [42, 43] and poor patient–ventilator synchrony [44, 45]. This might lead to progression of lung inflammation and damage [42, 43, 46], highlighted in the concept of patient self-inflicted lung injury [47, 48]. Further studies are needed to investigate the optimal balance between spontaneous breathing and avoiding VILI in ARDS patients during ECMO [25].
Limitations
There are some limitations in our study that should be addressed. First, we studied the physiological effects of apneic ventilation on oxygenation, DO2 and lung stress and strain in the initial phase of ECMO with deeply sedated and paralyzed patients. Therefore, the results of our study apply only to the early treatment phase without spontaneous breathing and with moderate to heavy sedation as recommended by current ELSO guidelines [6]. Neuromuscular blockade was used during the early treatment phase to prevent spontaneous breathing and to minimize airway pressures and transpulmonary pressure swings. This approach is supported by the experimental data of Guldner et al. [46] and clinical data published by Schmidt et al., where higher spontaneous respiratory rates during the first 2 days of ECMO were associated with higher 6-month mortality [5]. During ECMO treatment, discontinuation of neuromuscular blockade to allow spontaneous breathing is required to wean patients from the ECMO circuit. However, the optimal timing to minimize sedation and promote spontaneous breathing in the different phases of ARDS is unclear and needs further investigation [25].
Second, we studied the complex interaction between ventilation strategy, gas-exchange and hemodynamics only in a limited time frame. It is possible that the physiological effects of the ventilation strategy may vary during a prolonged observation period. The lower Pmean in apneic ventilation might promote better drainage of pulmonary interstitial fluid [49]. In contrast, cyclic lung stretch might be important to stimulate surfactant production [50]. Further studies are needed to investigate the long-term consequences of an apneic ventilation strategy and the effects on lung function and healing. As experimentally shown by Kolobow et al. decades ago, apneic ventilation with inadequate PEEP may decrease the functional residual capacity by approximately 50% if sustained for longer periods of time [35]. This may also have affected the data on lung aeration as the CT scan was performed after the comparison of different ventilation strategies. In case of a decreased end-expiratory lung volume, higher ECMO blood flows might be needed to maintain oxygenation despite the resulting “permissive” atelectasis and to protect the right ventricle from volume overload due to pulmonary hypertension. To test the hypothesis generated by our study, prospective trials with patient-centered outcomes are needed.
Third, this is a monocentric study in a specific population of patients with mainly pulmonary ARDS reflecting the specific management standard operating procedure and ventilation protocol of our unit. Therefore, the results may not be generalized to other ARDS subgroups treated with ECMO as there is data supporting the presence of at least two phenotypes in ARDS which might warrant different treatment strategies [51, 52, 53].
Fourth, only one single ventilation setting was investigated and compared to apneic ventilation. Thus, we cannot exclude that the effect of the ventilation strategy on oxygenation, DO2, stress, strain and regional air distribution may differ between different ventilation settings. We titrated PEEP according to the lowest Estat,RS to minimize Pdriv, the only ventilation parameter during ECMO that was independently associated with mortality in a recent analysis [7].
Finally, the calculation of DO2 is based on stroke volume measurements with transpulmonary thermodilution and not echocardiography which is commonly defined as gold standard [54]. Although SV measurement with transpulmonary thermodilution has recently been shown not to be affected by ECMO blood flow [55 we cannot exclude a systematical error of CO measurement.
Conclusions
In patients with severe ARDS managed with ECMO, apneic ventilation decreased oxygenation but preserved DO2 due to an increase in CO. Ultraprotective ventilation considerably increased stress, strain and mechanical power. As the optimal ventilation strategy in ECMO patients is unclear, apneic ventilation might be considered as one of the options in the initial phase of ECMO treatment in severe ARDS patients to facilitate lung rest and prevent VILI.
Availability of data and materials
The data sets used and analyzed during the current study are available from the corresponding author on reasonable request.
Abbreviations
- APACHE II:
-
Acute physiology and chronic health evaluation II score
- ARDS:
-
Acute respiratory distress syndrome
- CO:
-
Cardiac output
- CT:
-
Computed tomography
- CVP:
-
Central venous pressure
- DO2 :
-
Oxygen delivery
- ECMO:
-
Extracorporeal membrane oxygenation
- ELSO:
-
Extracorporeal life support organisation
- E stat,RS :
-
Static elastance of the respiratory system
- E stat,L :
-
Static elastance of the lung
- E stat,CW :
-
Static elastance of the chest wall
- FiO2 :
-
Fraction of inspired oxygen
- Hb:
-
Hemoglobin
- HR:
-
Heart rate
- IBW:
-
Ideal body weight
- ICU:
-
Intensive care unit
- MAP:
-
Mean arterial pressure
- MV:
-
Mechanical ventilation
- PaCO2 :
-
Arterial partial pressure of carbon dioxide
- pH:
-
Negative logarithm of the molar concentration of dissolved hydronium ions
- PaO2 :
-
Arterial partial pressure of oxygen
- P driv :
-
Driving pressure
- PEEP:
-
Positive end-expiratory pressure
- P mean :
-
Mean airway pressure
- P peak :
-
End-inspiratory peak pressure
- P plat :
-
End-inspiratory plateau pressure
- PRESERVE:
-
Predicting Death for Severe ARDS on vv-ECMO score
- RESP:
-
Respiratory ECMO survival prediction score
- RR:
-
Respiratory rate
- SAPS II:
-
Simplified acute physiology score II
- SaO2 :
-
Arterial oxygen saturation
- SOFA:
-
Sequential Organ Failure Assessment
- VCV:
-
Volume-controlled ventilation
- VILI:
-
Ventilator-induced lung injury
- V T :
-
Tidal volume
- ZEEP:
-
Zero end-expiratory pressure
References
Ware LB, Matthay MA. The acute respiratory distress syndrome. N Engl J Med. 2000;342(18):1334–49.
Combes A, Hajage D, Capellier G, Demoule A, Lavoue S, Guervilly C, et al. Extracorporeal membrane oxygenation for severe acute respiratory distress syndrome. N Engl J Med. 2018;378(21):1965–75.
Combes A, Peek GJ, Hajage D, Hardy P, Abrams D, Schmidt M, et al. ECMO for severe ARDS: systematic review and individual patient data meta-analysis. Intensive Care Med. 2020;46(11):2048–57.
Karagiannidis C, Brodie D, Strassmann S, Stoelben E, Philipp A, Bein T, et al. Extracorporeal membrane oxygenation: evolving epidemiology and mortality. Intensive Care Med. 2016;42(5):889–96.
Schmidt M, Pham T, Arcadipane A, Agerstrand C, Ohshimo S, Pellegrino V, et al. Mechanical ventilation management during extracorporeal membrane oxygenation for acute respiratory distress syndrome. An international multicenter prospective cohort. Am J Respir Crit Care Med. 2019;200(8):1002–12.
Tonna JE, Abrams D, Brodie D, Greenwood JC, Rubio Mateo-Sidron JA, Usman A, et al. Management of adult patients supported with venovenous extracorporeal membrane oxygenation (VV ECMO): Guideline from the Extracorporeal Life Support Organization (ELSO). ASAIO J. 2021;67(6):601–10.
Serpa Neto A, Schmidt M, Azevedo LC, Bein T, Brochard L, Beutel G, et al. Associations between ventilator settings during extracorporeal membrane oxygenation for refractory hypoxemia and outcome in patients with acute respiratory distress syndrome: a pooled individual patient data analysis : Mechanical ventilation during ECMO. Intensive Care Med. 2016;42(11):1672–84.
Marini JJ, Rocco PRM, Gattinoni L. Static and dynamic contributors to ventilator-induced lung injury in clinical practice. Pressure, energy, and power. Am J Respir Crit Care Med. 2020;201(7):767–74.
Nakane M. Biological effects of the oxygen molecule in critically ill patients. J Intensive Care. 2020;8(1):95.
Gattinoni L, Tonetti T, Quintel M. How best to set the ventilator on extracorporeal membrane lung oxygenation. Curr Opin Crit Care. 2017;23(1):66–72.
Le Gall JR, Lemeshow S, Saulnier F. A new simplified acute physiology score (SAPS II) based on a European/North American multicenter study. JAMA. 1993;270(24):2957–63.
Vincent JL, Moreno R, Takala J, Willatts S, De Mendonca A, Bruining H, et al. The SOFA (Sepsis-related Organ Failure Assessment) score to describe organ dysfunction/failure. On behalf of the Working Group on Sepsis-Related Problems of the European Society of Intensive Care Medicine. Intensive Care Med. 1996;22(7):707–10.
Knaus WA, Draper EA, Wagner DP, Zimmerman JE. APACHE II: a severity of disease classification system. Crit Care Med. 1985;13(10):818–29.
Schmidt M, Bailey M, Sheldrake J, Hodgson C, Aubron C, Rycus PT, et al. Predicting survival after extracorporeal membrane oxygenation for severe acute respiratory failure. The Respiratory Extracorporeal Membrane Oxygenation Survival Prediction (RESP) score. Am J Respir Crit Care Med. 2014;189(11):1374–82.
Schmidt M, Zogheib E, Roze H, Repesse X, Lebreton G, Luyt CE, et al. The PRESERVE mortality risk score and analysis of long-term outcomes after extracorporeal membrane oxygenation for severe acute respiratory distress syndrome. Intensive Care Med. 2013;39(10):1704–13.
Ely EW, Truman B, Shintani A, Thomason JW, Wheeler AP, Gordon S, et al. Monitoring sedation status over time in ICU patients: reliability and validity of the Richmond Agitation-Sedation Scale (RASS). JAMA. 2003;289(22):2983–91.
Talmor D, Sarge T, O’Donnell CR, Ritz R, Malhotra A, Lisbon A, et al. Esophageal and transpulmonary pressures in acute respiratory failure. Crit Care Med. 2006;34(5):1389–94.
Krebs J, Pelosi P, Rocco PRM, Hagmann M, Luecke T. Positive end-expiratory pressure titrated according to respiratory system mechanics or to ARDSNetwork table did not guarantee positive end-expiratory transpulmonary pressure in acute respiratory distress syndrome. J Crit Care. 2018;48:433–42.
Malbouisson LM, Muller JC, Constantin JM, Lu Q, Puybasset L, Rouby JJ, et al. Computed tomography assessment of positive end-expiratory pressure-induced alveolar recruitment in patients with acute respiratory distress syndrome. Am J Respir Crit Care Med. 2001;163(6):1444–50.
Mekontso Dessap A, Boissier F, Charron C, Begot E, Repesse X, Legras A, et al. Acute cor pulmonale during protective ventilation for acute respiratory distress syndrome: prevalence, predictors, and clinical impact. Intensive Care Med. 2016;42(5):862–70.
Spieth PM, Carvalho AR, Guldner A, Kasper M, Schubert R, Carvalho NC, et al. Pressure support improves oxygenation and lung protection compared to pressure-controlled ventilation and is further improved by random variation of pressure support. Crit Care Med. 2011;39(4):746–55.
Bartlett RH. Physiology of gas exchange during ECMO for respiratory failure. J Intensive Care Med. 2017;32(4):243–8.
Schoettler JJ, Kirschning T, Hagmann M, Hahn B, Fairley AM, Centner FS, et al. Maintaining oxygen delivery is crucial to prevent intestinal ischemia in critical ill patients. PLoS ONE. 2021;16(7): e0254352.
Hayes MA, Timmins AC, Yau EH, Palazzo M, Hinds CJ, Watson D. Elevation of systemic oxygen delivery in the treatment of critically ill patients. N Engl J Med. 1994;330(24):1717–22.
Abrams D, Schmidt M, Pham T, Beitler JR, Fan E, Goligher EC, et al. Mechanical ventilation for acute respiratory distress syndrome during extracorporeal life support. Research and practice. Am J Respir Crit Care Med. 2020;201(5):514–25.
Ferguson ND, Fan E, Camporota L, Antonelli M, Anzueto A, Beale R, et al. The Berlin definition of ARDS: an expanded rationale, justification, and supplementary material. Intensive Care Med. 2012;38(10):1573–82.
Fan E, Del Sorbo L, Goligher EC, Hodgson CL, Munshi L, Walkey AJ, et al. An Official American Thoracic Society/European Society of Intensive Care Medicine/Society of Critical Care Medicine Clinical Practice Guideline: mechanical ventilation in adult patients with acute respiratory distress syndrome. Am J Respir Crit Care Med. 2017;195(9):1253–63.
Terragni PP, Rosboch G, Tealdi A, Corno E, Menaldo E, Davini O, et al. Tidal hyperinflation during low tidal volume ventilation in acute respiratory distress syndrome. Am J Respir Crit Care Med. 2007;175(2):160–6.
Bein T, Weber-Carstens S, Goldmann A, Muller T, Staudinger T, Brederlau J, et al. Lower tidal volume strategy ( approximately 3 ml/kg) combined with extracorporeal CO2 removal versus “conventional” protective ventilation (6 ml/kg) in severe ARDS: the prospective randomized Xtravent-study. Intensive Care Med. 2013;39(5):847–56.
Combes A, Fanelli V, Pham T, Ranieri VM, European Society of Intensive Care Medicine Trials G, the Strategy of Ultra-Protective lung ventilation with Extracorporeal CORfN-OmtsAi. Feasibility and safety of extracorporeal CO2 removal to enhance protective ventilation in acute respiratory distress syndrome: the SUPERNOVA study. Intensive Care Med. 2019;45(5):592–600.
Marhong JD, Telesnicki T, Munshi L, Del Sorbo L, Detsky M, Fan E. Mechanical ventilation during extracorporeal membrane oxygenation. An international survey. Ann Am Thorac Soc. 2014;11(6):956–61.
Del Sorbo L, Goffi A, Tomlinson G, Pettenuzzo T, Facchin F, Vendramin A, et al. Effect of driving pressure change during extracorporeal membrane oxygenation in adults with acute respiratory distress syndrome: a randomized crossover physiologic study. Crit Care Med. 2020;48(12):1771–8.
Rozencwajg S, Guihot A, Franchineau G, Lescroat M, Brechot N, Hekimian G, et al. Ultra-protective ventilation reduces biotrauma in patients on venovenous extracorporeal membrane oxygenation for severe acute respiratory distress syndrome. Crit Care Med. 2019;47(11):1505–12.
Araos J, Alegria L, Garcia P, Cruces P, Soto D, Erranz B, et al. Near-apneic ventilation decreases lung injury and fibroproliferation in an acute respiratory distress syndrome model with extracorporeal membrane oxygenation. Am J Respir Crit Care Med. 2019;199(5):603–12.
Kolobow T, Gattinoni L, Tomlinson T, Pierce JE. An alternative to breathing. J Thorac Cardiovasc Surg. 1978;75(2):261–6.
Schmidt M, Stewart C, Bailey M, Nieszkowska A, Kelly J, Murphy L, et al. Mechanical ventilation management during extracorporeal membrane oxygenation for acute respiratory distress syndrome: a retrospective international multicenter study. Crit Care Med. 2015;43(3):654–64.
Bastia L, Engelberts D, Osada K, Katira BH, Damiani LF, Yoshida T, et al. Role of PEEP and regional transpulmonary pressure in asymmetrical lung injury. Am J Respir Crit Care Med. 2020. https://doi.org/10.1164/rccm.202005-1556OC.
Quintel M, Busana M, Gattinoni L. Breathing and ventilation during extracorporeal membrane oxygenation: how to find the balance between rest and load. Am J Respir Crit Care Med. 2019;200(8):954–6.
Protti A, Andreis DT, Monti M, Santini A, Sparacino CC, Langer T, et al. Lung stress and strain during mechanical ventilation: any difference between statics and dynamics? Crit Care Med. 2013;41(4):1046–55.
McNamee JJ, Gillies MA, Barrett NA, Perkins GD, Tunnicliffe W, Young D, et al. Effect of lower tidal volume ventilation facilitated by extracorporeal carbon dioxide removal vs standard care ventilation on 90-day mortality in patients with acute hypoxemic respiratory failure: the REST randomized clinical trial. JAMA. 2021. https://doi.org/10.1001/jama.2021.21005.
Goligher EC, Dres M, Patel BK, Sahetya SK, Beitler JR, Telias I, et al. Lung- and diaphragm-protective ventilation. Am J Respir Crit Care Med. 2020;202(7):950–61.
Yoshida T, Uchiyama A, Matsuura N, Mashimo T, Fujino Y. The comparison of spontaneous breathing and muscle paralysis in two different severities of experimental lung injury. Crit Care Med. 2013;41(2):536–45.
Yoshida T, Torsani V, Gomes S, De Santis RR, Beraldo MA, Costa EL, et al. Spontaneous effort causes occult pendelluft during mechanical ventilation. Am J Respir Crit Care Med. 2013;188(12):1420–7.
Mauri T, Bellani G, Grasselli G, Confalonieri A, Rona R, Patroniti N, et al. Patient-ventilator interaction in ARDS patients with extremely low compliance undergoing ECMO: a novel approach based on diaphragm electrical activity. Intensive Care Med. 2013;39(2):282–91.
Beitler JR, Sands SA, Loring SH, Owens RL, Malhotra A, Spragg RG, et al. Quantifying unintended exposure to high tidal volumes from breath stacking dyssynchrony in ARDS: the BREATHE criteria. Intensive Care Med. 2016;42(9):1427–36.
Guldner A, Kiss T, Bluth T, Uhlig C, Braune A, Carvalho N, et al. Effects of ultraprotective ventilation, extracorporeal carbon dioxide removal, and spontaneous breathing on lung morphofunction and inflammation in experimental severe acute respiratory distress syndrome. Anesthesiology. 2015;122(3):631–46.
Yoshida T, Amato MBP, Kavanagh BP, Fujino Y. Impact of spontaneous breathing during mechanical ventilation in acute respiratory distress syndrome. Curr Opin Crit Care. 2019;25(2):192–8.
Brochard L, Slutsky A, Pesenti A. Mechanical ventilation to minimize progression of lung injury in acute respiratory failure. Am J Respir Crit Care Med. 2017;195(4):438–42.
Malbrain M, Pelosi P. Open up and keep the lymphatics open: they are the hydraulics of the body! Crit Care Med. 2006;34(11):2860–2.
Edwards YS. Stretch stimulation: its effects on alveolar type II cell function in the lung. Comp Biochem Physiol A Mol Integr Physiol. 2001;129(1):245–60.
Calfee CS, Delucchi K, Parsons PE, Thompson BT, Ware LB, Matthay MA, et al. Subphenotypes in acute respiratory distress syndrome: latent class analysis of data from two randomised controlled trials. Lancet Respir Med. 2014;2(8):611–20.
Famous KR, Delucchi K, Ware LB, Kangelaris KN, Liu KD, Thompson BT, et al. Acute respiratory distress syndrome subphenotypes respond differently to randomized fluid management strategy. Am J Respir Crit Care Med. 2017;195(3):331–8.
Sinha P, Delucchi KL, Thompson BT, McAuley DF, Matthay MA, Calfee CS, et al. Latent class analysis of ARDS subphenotypes: a secondary analysis of the statins for acutely injured lungs from sepsis (SAILS) study. Intensive Care Med. 2018;44(11):1859–69.
Vieillard-Baron A, Matthay M, Teboul JL, Bein T, Schultz M, Magder S, et al. Experts’ opinion on management of hemodynamics in ARDS patients: focus on the effects of mechanical ventilation. Intensive Care Med. 2016;42(5):739–49.
Loosen G, Conrad AM, Hagman M, Essert N, Thiel M, Luecke T, et al. Transpulmonary thermodilution in patients treated with veno-venous extracorporeal membrane oxygenation. Ann Intensive Care. 2021;11(1):101.
Acknowledgements
None.
Funding
Open Access funding enabled and organized by Projekt DEAL. This study was supported by Departmental Funds.
Author information
Authors and Affiliations
Contributions
PTG, CB, TL and JK participated in the study design. PTG, CB, IB and JK performed the study. CB, JB, KWM, PP, TL and JK processed the data and performed the statistical analysis. CB, PP, PRMR, TL and JK wrote the manuscript. All authors read and approved the final manuscript.
Corresponding author
Ethics declarations
Ethics approval and consent to participate
The study was approved (registration number 2012-316 N-MA) by the local ethics committee (Medizinische Ethikkommission II, University Medical Centre Mannheim, Medical Faculty Mannheim of the University of Heidelberg, Mannheim), registered at the German Clinical Trials Register (DRKS00013967) and written informed consent of each patient or next of kin was acquired.
Consent for publication
Not applicable.
Competing interests
The authors declare that they have no competing interests.
Additional information
Publisher's Note
Springer Nature remains neutral with regard to jurisdictional claims in published maps and institutional affiliations.
Supplementary Information
Additional file 1.
Inclusion criteria, exclusion criteria, criteria for discontinuation of the study. Fig. S1. Schematic flow chart of the study design. Ventilator management. Calculation of respiratory system mechanics. Computed tomography assessment.
Rights and permissions
Open Access This article is licensed under a Creative Commons Attribution 4.0 International License, which permits use, sharing, adaptation, distribution and reproduction in any medium or format, as long as you give appropriate credit to the original author(s) and the source, provide a link to the Creative Commons licence, and indicate if changes were made. The images or other third party material in this article are included in the article's Creative Commons licence, unless indicated otherwise in a credit line to the material. If material is not included in the article's Creative Commons licence and your intended use is not permitted by statutory regulation or exceeds the permitted use, you will need to obtain permission directly from the copyright holder. To view a copy of this licence, visit http://creativecommons.org/licenses/by/4.0/. The Creative Commons Public Domain Dedication waiver (http://creativecommons.org/publicdomain/zero/1.0/) applies to the data made available in this article, unless otherwise stated in a credit line to the data.
About this article
Cite this article
Graf, P.T., Boesing, C., Brumm, I. et al. Ultraprotective versus apneic ventilation in acute respiratory distress syndrome patients with extracorporeal membrane oxygenation: a physiological study. j intensive care 10, 12 (2022). https://doi.org/10.1186/s40560-022-00604-9
Received:
Accepted:
Published:
DOI: https://doi.org/10.1186/s40560-022-00604-9