Abstract
Piercing sucking pests are destructive to many strategic crops all over the world. Botanical pesticides can be used to control these pests. A new withanolide derivative 3 named sominone A ((20R,22R)-1α,3β,20,27-tetrahydroxywitha-5,24-dienolide) was isolated from the alkaloid fraction of the whole plant of Withania somnifera. In addition, there are three known compounds named withasomine 1, methyl isoferulate 2, and coagulin Q 4 were also isolated. The structures of isolated compounds were identified using different spectroscopic methods such as 1D, 2D NMR, and HRESIMS spectroscopy. The alkaloid fraction and the four isolated compounds were tested for their pesticidal activity against four piercing sucking pests (Aphis craccivora Koch, Bemisia tabaci Gennadius, Nezara viridula Linnaeus, and Tetranychus urticae Koch) that attack many strategic crops under laboratory conditions, along with azadirachtin (Okios 3.2% EC) as a positive control. The results showed that the alkaloid compound (withasomine 1) was the most toxic to A. craccivora, B. tabaci, N. viridula, and T. urticae, with LC50 values of 15.44, 36.61, 85.11, and 128.28 ppm, respectively, compared with the control. Withanolide compounds had moderate effects on all tested pests. Biochemical parameters of six enzymes; α-esterase, β-esterase, chitinase, acetylcholinesterase, glutathione-S-transferase, and peroxidase of A. craccivora were estimated at the LC50 value of the most potent compound, withasomine 1 and the values were 38.83, 72.86, 31.45, 506.4, 2.62, and 251.0, respectively. The results demonstrated that all enzymes activity levels were increased compared with the control except a remarkable inhibition in AChE enzyme level was observed compared with control. Therefore, the alkaloid fraction of W. somnifera is a promising extract that contains many active compounds that can be used as a natural pesticide against many harmful pests in agriculture crops.
Graphical Abstract

Similar content being viewed by others
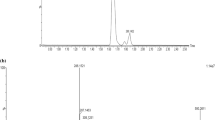
Introduction
Natural pesticides of plant origin occupied an interesting position for scientists all over the world. This is due to the high safety rate of these compounds for humans and the environment in general, in addition to their rapid degradation in the environment. The excessive use of synthetic pesticides cause many harms to humans, animals and plants and led to insect resistance to these pesticides [1]. Withania somnifera Dun, belonging to the Solanaceae family, generally known as India ginseng, has been used as a food for promoting health and longevity by activating the organ systems and immune system [2, 3]. Therefore, using this type of extracts as pesticides to control pests, especially in vegetable crops, is safer for the environment and humans. Many natural product compounds can be used as pesticides, especially alkaloids [4]. Quinolizidine alkaloids like Anagyrine and Spartiene compounds have insecticidal activity against Aphis gosspyii and Amrasca biguttula [5]. Apart from the pesticidal activity, withanolides have other biological activities such as immune booster and anti-viral (COVID-19) [6]. The aphicidal activity of W. somnifera extracts was studied by Noureldeen et al. against rose aphid, Macrosiphum rosae [7]. W. somnifera is rich mainly with withanolides and quinolizidine alkaloids, in addition to phenols, steroids, saponins, flavonoids, and glycosides [8]. The metabolite profiling of W. somnifera root extract was studied by HPLC and different spectroscopic methods that yielded numerous withanolides [9]. Zhao et al. isolated many derivatives of withanolides, such as withanoside VIII, IX, X, and XI, from the roots of W. somnifera and studied their neurite outgrowth activity on a human neuroblastoma SH-SY5Y cell line [10]. Sominone and sominolide were isolated from W. somnifera by Atta-ur-Rahman et al. [11]. The alkaloid content of W. somnifera roots and callus was identified by GC/MS analysis, like withasomnine and somniferine [12]. Nine withanosides were isolated from W. somnifera roots by Misra et al. [13]. Yan et al. used Viscosalactone B, isolated from W. somnifera, as a natural LSD1 inhibitor for the treatment of prostate cancer [14]. Several insects are serious pests that cause damage to many agricultural crops such as Aphis cracivora, Bemisia tabaci, Nezare viridalis and Tetrarhynchus urticae. In addition to this, these insects can also serve as indirect vector of viral diseases [15, 16]. Because of these reasons, there is an urgent need to control these insect pests using extracts from botanical agents. In this study, Withania somnifera Dun plant, which contains different active compounds especially alkaloids, was studied for the insecticidal properties against piercing-sucking pests that are harmful to the agricultural crops.
Materials and methods
Chemicals and instruments
TLC silica gel Merck GF254 precoated plates (20 × 20 cm) on aluminum sheets, alumina (aluminum oxide active neutral LOBA CHEMIE PVT. LTD., India), methanol (MeOH), ethyl acetate (EA), methylene chloride (MC), petroleum ether (60-80ºC) (PE), acetic acid and ammonia solution 25% were obtained from Edwic Company, Egypt. Spray reagent; Dragendorff's reagent. 1H NMR, 13C NMR, and 2D NMR analyses were measured using Bruker 400 MHz in CD3OD and CDCl3 with tetramethylsilane (TMS) as an internal standard. HRESI/MS spectrum in positive mode were carried out on an UPLC MS/MS "Agilent" 3100 "USA" with a TQ detector. A polarimeter, WXG-4, was used for optical rotation measurement. UV spectra were measured using the PG Instruments T80 + UV/vis spectrometer, UK. IR spectrum was recorded on ThermoFisher Nicolete IS10, USA.
Plant materials
Whole parts of the Withania somnifera plant, family Solanacease, were collected in August 2019 from Mansoura University Gardens, Mansoura City, Dakahlia, Egypt (31.04595°N–31.35402°E). The plant was identified by Dr. Maha El-Shamy, Associate Professor of Plant Ecology, Botany Department, Faculty of Science, Mansoura University, Egypt, according to Boulos [17].
Phytochemistry of W. somnifera
Extraction and liquid–liquid partitioning
The air-dried powder of the whole plant, W. somnifera (6 kg), was extracted with MeOH (5 L × 3), then evaporated at 40 ºC under reduced pressure till dryness. The dried extract was kept in the refrigerator until use. The alkaloid fraction of the plant was isolated according to the acid–base method. MeOH extract was dissolved in a small amount of methanol, and then acetic acid was added till pH 3 with stirring for 24 h. The non-alkaloid fraction was removed by MC solvent using a separating funnel. The aqueous layer was basified with 25% ammonium hydroxide to a pH 11, and then the alkaloid fraction was extracted by MC and yielded 8 g.
Processing of alkaloid fraction of W. somnifera
The alkaloid fraction (7 g) was separated by neutral aluminum oxide column chromatography using PT/EA as a mobile phase. Then, 100% MC was used, and MeOH was added to increase the polarity gradually. Six subfractions were obtained; subfraction I (170 mg, at the eluent system MC/MeOH, 95:5) was purified using PTLC (silica gel, EA/PT, 1:2, Rf 0.41, 9 mg) to give compound 1. Compound 2 was isolated from subfraction II (210 mg, MC/MeOH, 90:10) by PTLC (silica gel, MC, 100%, Rf 0.78, 14 mg). Subfraction IV (240 mg) that was obtained at the eluent system (MC/MeOH, 90:20) gave compound 3 after further purification using PTLC (silica gel, MC/MeOH, 9.5:0.5, Rf 0.44, 20 mg). Subfraction VI (430 mg, at the eluent system MC/MeOH, 70:30) gave compound 4 after purification by PTLC (silica gel, MC/MeOH, 4:1, Rf 0.41, 22 mg). Compounds 1, 3, and 4 gave an orange color on TLC with Dragendorff's reagent.
Characterization of separated compounds 1–4
The structural elucidation of the bioactive compounds (1–4) was carried out by different spectroscopic methods.
Compound 1: Pale yellow residue (9 mg), 1H NMR (400 MHz, CDCl3, δH, ppm, J, Hz): δH 7.82 (s, H-4), 7.45 (dd, J = 7.8, 1.2 Hz, 2H-2',6'), 7.36 (t, J = 7.8 Hz, 2H-3',5'), 7.19 (t, J = 7.8 Hz, H-4'), 4.19 (t, J = 7.5 Hz, 2H-5), 3.10 (t, J = 7.5 Hz, 2H-7), 2.69 (m, 2H-6).
Compound 2: Crystal sheets, m.p. 65–67 o; 1H NMR (400 MHz, CD3OD, δH, ppm, J, Hz): δH 7.60 (d, J = 15.9 Hz, H-7), 6.35 (d, J = 15.9 Hz, H-8), 7.17 (d, J = 1.8 Hz, H-2), 7.06 (dd, J = 8.2, 1.8 Hz, H-6), 6.80 (d, J = 8.2 Hz, H-5), 3.88 (s, 3H-11), 3.76 (s, 3H-10).
Compound 3: Amorphous powder (20 mg); [α]D21 + 15.87 (c 0.036, MeOH); IR υmax (film): 3309, 2940, 1696, 1601, 1456, 1385, 1133 and 1031 cm−1; UV λmax (MeOH): 428, 398, 284, 251 nm; 1H and 13C NMR (CDCl3) data (Table 1); HRESIMS (positive-ion mode) m/z 475.3199 [M + H]+ (calcd for C28H43O6, 475.3060).
Compound 4: Amorphous powder (22 mg),1H-NMR (400 MHz, CD3OD, δH, ppm, J, Hz): δH 3.80 (t, W1/2 = 5.0 Hz, H-1), 1.82 (m, H-2a), 2.13(m, H-2b), 4.05 (m, H-3), 2.32 (m, H-4a), 2.46 (m, H-4b), 5.51 (d, J = 5.0 Hz, H-6), 1.60 (m, H-7a), 1.96 (m, H-7b), 1.49 (m, H-8), 1.70 (m, H-9), 1.52 (m, 2H-11), 1.41 (m, H-12a), 2.00 (m, H-12b), 1.14 (m, H-14), 1.16 (m, H-15a), 1.69 (m, H-15b), 1.67 (m, H-16a), 1.89 (m, H-16b), 1.79 (m, H-17), 0.87 (s, 3H-18), 1.01 (s, 3H-19), 1.26 (s, 3H-21), 1.99 (s, 3H-26), 1.85 (s, 3H-27), 4.24 (dd, J = 3.4, 13.2 Hz, H-22), 2.29 (dd, J = 18.1, 2.6 Hz, H-23a), 2.57 (t, J = 15.8 Hz, H-23b), 4.37 (d, J = 7.8 Hz, H-1'), 3.14 (t, J = 7.8 Hz, H-2'), 3.36 (m, H-3'), 3.25 (m, H-4'), 3.27 (m, H-5'), 3.64 (dd, J = 11.6, 4.9 Hz, H-6a), 3.85(d, J = 11.6 Hz, H-6b). 13C-NMR (100 MHz, CD3OD): δC 168.9 (C-26), 152.9 (C-24), 139.0 (C-5), 125.5 (C-6), 122.0 (C-25), 102.6 (C-1'), 82.7 (C-22), 78.0 (C-3'),77.9 (C-5'), 76.3 (C-20), 75.1 (C-2'), 74.9 (C-3), 73.5 (C-1), 71.7 (C-4'), 62.8 (C-6'), 58.1 (C-14), 55.8 (C-17), 42.7 (C-10), 43.9 (C-13), 42.5 (C-9), 39.1 (C-4), 37.6 (C-2), 40.9 (C-12), 32.8 (C-7), 32.2 (C-23), 32.6 (C-8), 23.0 (C-16), 25.0 (C-15), 20.5 (C-28), 21.1 (C-11), 20.8 (C-21), 20.0 (C-19), 14.3 (C-18), 12.4 (C-27) (Additional file 1).
Evaluation of the insecticidal activity
Rearing of tested pests
Aphis craccivora
Aphis craccivora strains were collected from the farm of Faculty of Agriculture, Mansoura University, and they were known to be free from any contamination of insecticides. The aphids were maintained on cowpea plants under normal conditions in plastic greenhouse (3 × 2 × 2 m) [18].
Bemicia tabaci
Adult whiteflies (Bemicia tabaci) were collected from the farm of Faculty of Agriculture, Mansoura University. The whiteflies were maintained on tomato plants in entomological cages (1.5 × 1.5 × 1.0 m) under normal conditions [18].
Nezara viridula
The green stink bugs (N. viridula) were obtained from the farm of Faculty of Agriculture, Mansoura University, and reared on okra leaves (Abelmoschus esculentus) in the laboratory at a fixed temperature of 25 ± 2 °C, 65 ± 5% RH, and 12:12 (L:D) hr [19].
Tetranychus urticae
The spider mites, Tetranychus urticae Koch, were collected from an infested castor tree. The collected pest was transferred to freshly cleaned castor leaves on moist cotton in petri dishes. They were kept under laboratory conditions (25 ± 2 °C and 60 ± 5% RH) and continued for two generations to be sensitive and homogenous before use in toxicity tests [20].
Biological bioassays
A. craccivora
The tested plant samples were examined as insecticides by the spray method on cowpea leaves. Five concentrations of the alkaloid fraction (10, 20, 40, 80 and 160 ppm) and their isolated compounds (compound 1: 5, 10, 20, 40 and 80; 2: 20, 40, 60, 80 and 100; 3 and 4: 50, 100,150, 200 and 250 ppm) were prepared using Tween 80 with distilled water for each treatment (four replicates per concentration). Ten aphids in each replicate were transferred on a disc of cowpea leaf (4.0 cm in diameter) placed in petri dishes over agar (1.5%) before 30 min of treatment. Finally, each treatment was sprayed with 2 ml of each tested solution, then covered and kept at room temperature. Whereas, the control treatment was sprayed with distilled water containing Tween 80. Mortality was count after 24 h. Mortality% were corrected by using Abbott equation [21]. The LC50's, LC90's values and their confidence limits were obtained according to Finney [22], in addition to the slope of regression lines (LC-P line). Also, the toxicity index was measured by the sun equation [23].
Bemisia tabaci nymphs
Adults of B. tabaci (20 insects per cage) were collected and placed in cages containing tomato plant leaves. After 15 days, sections of tomato leaves (2 cm in diameter) containing 30 of the 3rd nymphs of B. tabaci were transferred over 2% agar medium into petri dishes. Five concentrations (four replicates for each one) of the W. somnifera alkaloid fraction (15, 30, 60, 120 and 240 ppm) and their isolated compounds (compound 1: 10, 20, 40, 80 and 160; 2: 25, 50, 75, 100 and 125; 3 and 4: 75, 150, 225, 300 and 375 ppm) were prepared. Only 2 ml of diluted solution were sprayed on each treatment, and water was used only in the control treatment. Mortality was accounted for after 24 h of treatment [24].
Nezara viridula
Five concentrations (4 replicates for each one) of the alkaloid fraction (100, 200, 300, 400 and 500 ppm) and its isolated compounds (compound 1: 25, 50, 100, 200 and 400; 2: 50, 100, 200, 400 and 800; 3 and 4: 150, 300,450, 600 and 750 ppm) were prepared. In each replicate, 10 adults of N. viridula were transferred to okra leaves in a glass jar. Each treatment was sprayed with 2 ml of test solution, and the control treatment was sprayed with distilled water containing Tween 80 and then covered. The mortality was recorded after 24 h [19].
Acaricidal activity
The effects of W. somnifera alkaloid fraction and its isolated compounds were evaluated against adult female T. urticae by the leaf-dip technique using five concentrations (200, 400, 600, 800 and 1000 ppm) and (compound 1: 50, 100, 150, 200 and 250; 2: 100, 200, 300, 400 and 500; 3 and 4: 250, 500, 750, 1000 and 1250 ppm), respectively according to Dawidar [25].
Biochemical investigation of A. craccivora
The most toxic compound (1) at LC50 value was used for measuring enzyme activities. Fifty individuals of A. craccivora were sprayed with one milliliter of compound (1) solution, in addition to a control treatment that sprayed with distilled water only. After 24 h of treatment, the live aphids were weighed and kept frozen in a suitable tube, according to Elhefni et al. [18]. The insect enzymes α-esterase (α-EST) and β-esterases (β-EST) were determined using α- and β-naphthol acetate as substrates, respectively. The mixture was incubated for exactly 15 min at 27 °C then 1 ml of diazoblue colour reagent was added. The change in colour was read at 600 and 555 nm for α- and β-naphthol, respectively. The activity was expressed as µg α- or β-naphthol released /min/g body weight [26]. Acetylcholinesterase (AChE) activity was measured by Simpson et al. method [27] using acetylcholine bromide (AChBr) at a level of 6 × 10–3 M and the reading was measured at 515 nm. Peroxidase (POD) activity was determined by Hammerschmidt et al. method. The reading was taken at 420 nm to a mixture of Pyrogallol (0.05 M) and 100 µl aphid enzyme extract. The enzyme activity was expressed as change in absorbance/min/g sample [28]. Glutathione-S-transferase (GST) was determined by added 25 µl of the substrate 1-chloro 2,4-dinitrobenzene solution (CDNB) to reaction mixture (1 ml of phosphate buffer, pH 6.5, 100 µl of glutathione (GSH) and 200 µl of aphid extract). The absorbance was taken at 340 nm and the enzyme activity was expressed as mmol/min/mg protein [29, 30]. Chitinase (CTase) was assayed according to the method described by Ishaaya and Casida [31]. The reaction mixture consisted of 0.12 ml (0.2 M) phosphate buffer (pH 6.6); 0.3 ml 0.5% colloidal chitin; 0.18 ml aphid extract. After 60 min. incubation at 37 °C, enzyme activity was terminated by adding 1.2 ml 3,5-dinitrosalycilic acid reagent (DNSA). The reaction mixture was heated at 100 °C for 5 min then cooled and diluted with 1.2 ml distilled water. Undigested chitin was sedimented by centrifugation for 15 min. at 6000 rpm and the absorbance was measured at 550 nm. Chitinase activity is expressed as µg N-acetylglucosamine released/g body weight/min. All analyses were assessed in the Analysis Unit of the Plant Protection Research Institute, Agriculture Research Center. Total protein contents were determined according to Bradford [32].
Statistical analysis
Data were analyzed using MINITAB®software program (version Minitab®21.4.1). Statistical differences between the two means was calculated using two-sample T-test. Differences are significant when P < 0.05. The LC50 and LC90 values of all treatments were determined using probit analysis.
Results and discussion
Identification of compounds (1–4)
Compound 1 (Fig. 1) was isolated as a pale yellow residue that gave a positive result with Dragendorff's reagent. 1H NMR data showed the presence of a monosubstituted benzene ring at δH 7.45 (dd, J = 7.8, 1.2 Hz, 2H-2', 6'), 7.36 (t, J = 7.8 Hz, 2H-3',5'), 7.19 (t, J = 7.8 Hz, H-4'). As well as the down-field proton of the pyrazole ring at δH 7.82 (s, H-4). Also, three different aliphatic methylene groups appeared at δH 4.19 (t, J = 7.5 Hz, 2H-5), 3.10 (t, J = 7.5 Hz, 2H-7), and 2.69 (m, 2H-6). These data were in agreement with those identified before as withasomine [33]. Compound 2 was isolated as a crystal sheets with m.p. 65–67 o. 1H NMR analysis indicated the presence of the ABX system at δH 7.17 (d, J = 1.8 Hz, H-2), 7.06 (dd, J = 8.2, 1.8 Hz, H-6), 6.80 (d, J = 8.2 Hz, H-5), in addition to two olefinic trans protons at δH 7.60 (d, J = 15.9 Hz, H-7), and 6.35 (d, J = 15.9 Hz, H-8). The above data indicated its identity as methyl isoferulate 2, which was confirmed by comparing their data with the isolated data by Bowden et al. [34].
Compound 3 (Fig. 1) was isolated as an amorphous powder that gave a positive test with Dragendorff's [35]. 13C NMR (Table 1) and HSQC (Hetereonuclear Single Quantum Coherence) spectra indicated signals for 28 carbon atoms, including 4 methyl, 9 methylene, 8 methine groups, and 7 quaternary carbon atoms containing one carbonyl group at δC 166.3 ppm. The HRESIMS spectrum of compound 3 showed a [M + H]+ peak at m/z 475.3199, in agreement with the molecular formula C28H43O6. IR analysis indicated the presence of an unsaturated six-membered lactone ring at 1696 and 1601 cm−1. The 1H NMR spectrum (Table 1) of compound 3 showed four proton singlets for quaternary methyl groups at δH 0.86, 1.02, 1.28, and 2.06 ppm for the C-18, C-19, C-21, and C-28 protons, respectively. Two doublet signals at δH 4.39 and 4.33 ppm (J = 12.5 Hz) were ascribed to methylenic protons of C-27. A methine proton signal was assigned at δH 4.28 (dd, J = 13.3, 3.3 Hz) that related to the proton of C-22 for lactone moiety. The above observations supported the presence of a tetracyclic steroidal skeleton with a lactone substituent [11].
Two oxygenated methine protons appeared at δH 3.83 (t, W1/2 = 5.0 Hz, H-1) and 3.97 (m, H-3) related to C-1 and C-3, respectively. The stereochemistry of the C-l (α) and C-3 (β) hydroxyl groups was assigned by comparing the coupling constant values and chemical shifts with the reported before withanolide lα, 3β, 20-trihydroxy (20R, 22R)-witha-5,24-dienolide [11, 36]. Also, the down-field proton signal at δH 5.57 (d, J = 5.0 Hz) was ascribed to the proton of C-6. The cross peaks between (H-3, 3.97) and (H-2, 1.73 and 2H-4, 2.37, 2.30), as well as the cross peaks between (H-22, 4.28) and (H-23, 2.53), were assigned by the H–H COSY spectrum (Fig. 2). The HMBC (Heteronuclear Multiple Bond Correlation) spectrum (Fig. 2) confirmed the correlation of 2H-27 at δH 4.33 and 4.39 ppm with C-24, C-25, and C-26 at δC 153.3, 125.8, and 166.3 ppm, respectively. As well, the proton at δH 3.83 (H-1) was connected to C-3 and C-5 at δC 66.5 and 137.6 ppm, respectively. Also, the positions of the four methyl groups were confirmed by HMBC analysis (Fig. 2). Therefore, the above-mentioned data led to the assignment of structure (3) ((20R,22R)-1α,3β,20,27-tetrahydroxywitha-5,24-dienolide) to sominone A.
Compound 4 was isolated as an amorphous powder that gave an orange color with Dragendorff's [35]. 13C NMR (Table 1) and HSQC analyses proved the presence of 34 carbon atom signals, including 5 methyl, 9 methylene, 13 methine groups, and 7 quaternary carbon atoms containing one carbonyl group at δc 168.9 ppm. 1H NMR analysis (c.f. experimental) indicated that compound 4 is similar to compound 3, but a methyl group singlet signal was found at δH 1.85 (s, 3H-27) instead of the oxygenated methylene group, as well as a glucose moiety connected to β-OH of C-3 of the withanolide at δH 4.05 (m, H-3). The HMBC spectrum indicated a correlation between the anomeric proton of glucose at δH 4.19 (d, J = 7.8 Hz, H-1') and the C-3 of withanolide at 74.9 ppm. The above-mentioned data were in agreement with the structure of Coagulin Q [37, 38]. Compounds 2, 3, and 4 were not alkaloid compounds but contained α, β-unsaturated ester especially withanolide derivatives that behave like alkaloids and gave positive results with Dragendorff's reagent [35].
Insecticidal activity
The effects of the alkaloid fraction of W. somnifera and their isolated compounds (1–4) (Fig. 1), as well as Azadirachtin (Okios 3.2% EC), against A. craccivora, B. tabaci, N. viridula, and T. urticae, were tested and compared. Generally, it is clear that the alkaloid fraction and their compounds have a toxicity effect against the four pests with different ratios (Tables 2, 3, 4, and 5). Results in Table 2 showed that withasomine 1 was the most potent against A. craccivora, with a LC50 value of 15.44 ppm, compared to alkaloid fraction and the other compounds. The LC50 values of treatments in Table 3 indicated that withasomine 1 and Azadirachtin were the most effective against B. tabaci with LC50 values of 36.61 and 36.78 ppm and against N. viridula with LC50 values of 85.11 and 98.26 ppm, respectively.
As shown in Table 5, the most toxic compound was found to be withasomine 1 toward T. urticae, with a LC50 value of 128.29 ppm. It is clear that withasomine 1, azadirachtin, isoferulate 2, and the alkaloid fraction have strong toxicity against A. craccivora, B. tabaci, and N. viridula, but withanolides, sominone A, and coagulin Q have moderate effects. In the case of T. urticae, withasomine 1 and isoferulate 2 were more potent than other treatments.
Salamatullah (2022) found that the hydroethanol extract of Withania adpressa has insecticidal activity on Callosobruchus maculatus [39]. A methanol extract of W. somnifera was used as an aphicide against the rose aphid Macrosiphum rosae [6]. W. somnifera root extract was toxic to Fall armyworm, Spodoptera frugiperda [40], and Spodoptera litura pupae and larvae [41]. Withania coagulans, a plant from the same genus as W. somnifera, was tested as an insecticide against the green peach aphid, Myzus persicae [42], and B. tabaci [43]. Al-Ani et al. explained the important role of alkaloids as insecticides [44]. Phenolic compounds (ferulic acid) can be used to protect the plants from all kinds of pests [45].
Effect of withasomine (1) on A. craccivora enzyme activity
The effects of the most potent alkaloid compound, withasomine 1, on A. craccivora enzymes (α, β-EST, CTase, AChE, GST, and POD) were measured (Table 6 and Fig. 3). The activity of α-EST and β-EST with values of 38.83 and 72.86 µg, respectively, slightly increased with no significant difference after 24 h of treatment when compared with the control. On the contrary, the activity of the GST enzyme increased with highly significant difference compared with the control (2.62 and 1.92 mmol, respectively). α, β-EST, and GST enzymes have important roles in the elimination of strange compounds like insecticides from insects through metabolic processes [46, 47]. These results were in agreement with the biochemical studies that proved an increase in the detoxifying enzymes activity (α, β-EST, and GST) as a result of the increased insect resistance to insecticides [47, 48]. Also, POD activity (251.0) increased, with significant difference compared to the control (242.3). The POD enzyme is known as an antioxidant enzyme that acts as a protective cellular system by producing H2O from H2O2. This reaction decreases the damage to the biomembrane caused by reactive oxygen species [49, 50]. The activity of CTase (31.45 µg) increased with significant compared to the control (10.53 µg). Previous studies proved that the development of insect resistance to pesticides mainly depends on the thickness of the chitin layer [51]. Increasing the CTase activity led to chitin hydrolysis, in addition to an increase in the cell membrane permeability; therefore, the soluble proteins and sugars were reduced. These results are in agreement with the effect of tea saponins on Ectropis oblique [51, 52].
The activity of AChE showed a significant reduction compared to the control group (506.4 and 643.8 µg AchBr/min/g b wt.), respectively. Inhibition of AChE indicates an interaction between AChE and the bioactive compounds. Phenolic compounds and alkaloids affect the nervous system of insects and inhibit AChE activity [7]. These results were in agreement with the previous studies [53]. Therefore, it is clear to us from the results that withasomine 1 is the active substance that has the greatest effect on the pests under study.
Availability of data and materials
The datasets used and/or analyzed during the current study are available from the corresponding author on reasonable request.
References
Damalas CA, Eleftherohorinos IG (2011) Pesticide exposure, safety issues, and risk assessment indicators. Int J Environ Res Public Health 8(12):1402–1419. https://doi.org/10.3390/ijerph8051402
Mukherjee PK, Banerjee S, Biswas S, Das B, Kar A, Katiyar CK (2021) Withania somnifera (L.) Dunal-Modern perspectives of an ancient Rasayana from Ayurveda. J Ethnopharmacol 264:113157. https://doi.org/10.1016/j.jep.2020.113157
Lee SR, Lee BS, Yu JS, Kang H, Yoo MJ, Yi SY, Han JW, Kim S, Kim JK, Kim JC, Kim KH (2022) Identification of anti-adipogenic withanolides from the roots of Indian ginseng (Withania somnifera). J Ginseng Res 46(3):357–366. https://doi.org/10.1016/j.jgr.2021.09.004
Chowa’nski S, Adamski Z, Marciniak P, Rosi’nski G, Büyükgüzel E, Büyükgüzel K, Falabella P, Scrano L, Ventrella E, Lelario F, Bufo SA (2016) A Review of bioinsecticidal activity of solanaceae alkaloids. Toxins 8:60. https://doi.org/10.3390/toxins8030060
Kamel AI, El-Rokh AR, Dawidar AM, Abdel-Mogib M (2024) Bioactive compounds from Retama raetam (Forssk.) Webb & Berthel. and their insecticidal activity against cotton pests Aphis gosspyii and Amrasca biguttula. Fitoterapia 172:105749. https://doi.org/10.1016/j.fitote.2023.105749
Noureldeen A, Kumar U, Asad M, Darwish H, Alharthi S, Fawzy MA, Al-Barty AM, Alotaibi SS, Fallatah A, Alghamdi A, Albogami B, Alkashgry N (2022) Aphicidal activity of five plant extracts applied singly or in combination with entomopathogenic bacteria, Xenorhabdus budapestensis against rose aphid, Macrosiphum rosae (Hemiptera: Aphididae). J King Saud Univ Sci 34(8):102306. https://doi.org/10.1016/j.jksus.2022.102306
Padgham DE, Kimmins FM, Ranga Rao GV (1990) Resistance in groundnut (Arachis hypogaea L.) to Aphis craccivora (Koch). Ann Appl Biol 177:285–294. https://doi.org/10.1111/j.1744-7348.1990.tb04214.x
Saleem S, Muhammad G, Hussain MA, Altaf M, Nasir S, Bukhari A (2020) Withania somnifera L.: insights into the phytochemical profile, therapeutic potential, clinical trials, and future prospective. Iran J Basic Med Sci 23(12):1501–1526. https://doi.org/10.22038/IJBMS.2020.44254.10378
Trivedi MK, Panda P, Sethi KK, Jana S (2017) Metabolite profiling in Withania somnifera roots hydroalcoholic extract using LC/MS. GC/MS NMR Spectrosc Chem Biodivers 14(3):e1600280. https://doi.org/10.1002/cbdv.201600280
Zhao J, Nakamura N, Hattori M, Kuboyama T, Tohda C, Komatsu K (2002) Withanolide derivatives from the roots of Withania somnifera and their neurite outgrowth activities. Chem Pharm Bull(Tokyo) 50(6):760–765. https://doi.org/10.1248/cpb.50.760
Atta-ur-Rahman JSA, Choudhary MI (1992) Two New Withanolides from Withania somnifera. Heterocycles 34(4):689–698
Sharma RA, Goswami M, Yadav A (2013) GC-MS screening of alklaoids of Withania somnifera L. in vivo and in vitro. Indian J Appl Res 3(8):63–66. https://doi.org/10.36106/ijar
Misra L, Mishra P, Pandey A, Sangwan RS, Sangwan NS, Tuli R (2008) Withanolides from Withania somnifera roots. Phytochemistry 69(4):1000–1004. https://doi.org/10.1016/j.phytochem.2007.10.024
Yan G, Zhang H, Li Y, Miao G, Liu X, Lv Q (2023) Viscosalactone B, a natural LSD1 inhibitor, inhibits proliferation in vitro and in vivo against prostate cancer cells. Invest New Drugs 41(1):134–141. https://doi.org/10.1007/s10637-023-01330-1
Khanala P, Chikhaleb R, Deyc YN, Pashad I, Chande S, Guravf N, Ayyanarg M, Patila BM, Guravh S (2022) Withanolides from Withania somnifera as an immunity booster and their therapeutic options against COVID-19. J Biomol Struct Dyn 40(12):5295–5308. https://doi.org/10.1080/07391102.2020.1869588
Cohen S, Berlinger MJ (1986) Transmission and cultural control of whitefly-borne viruses. Agr Ecosyst Environ 17(1–2):89–97. https://doi.org/10.1016/0167-8809(86)90030-7
Boulos L (2000) Flora of Egypt. Al Hadara Publishing, Cairo, p 288
Elhefni MA, El-Rokh AR, El-Rafey HH, Tadros LK, Taher MA (2023) Phytochemical profiling and isolation of bioactive polyphenols from Ipomoea carnea. Egypt J Chem 66(12):529–543. https://doi.org/10.21608/ejchem.2023.199835.7725
El-Gendy RM, El-Shafiey SN (2018) Eco-friendly control strategies of green stink bug, Nezara viridula L. (Hemiptera: Pentatomidae): repellency and toxicity effects of Callistemon citrinus, bottle brush essential oil. J Plant Prot Pathol 9(12):807–813
Mostafa ME (2021) Acaricidal potential and phytochemical analysis of the indigenous Centaurea aegyptiaca L. against Tetranychus urticae Koch (Acari: Tetranychidae). Int J Entomol Res 6(1):40–45
Abbott WS (1925) A method of computing the effectiveness of an insecticide. J Econ Entomol 18(2):265–267. https://doi.org/10.1093/jee/18.2.265a
Finney DJ (1971) Probit Analysis, a statistical treatment of the sigmoid response curve, 7th edn. Cambridge Univ. Press, Cambridge, p 333
Sun YP (1950) Toxicity index, an improved method of comparing the relative toxicity of insecticides. J Econ Entomol 43:45–53. https://doi.org/10.1186/s12906-020-03100-5
Cruz-Estrada A, Gamboa-Angulo M, Borges-Argáez R, Ruiz-Sánchez E (2013) Insecticidal effects of plant extracts on immature whitefly Bemisia tabaci Genn. (Hemiptera: Aleyroideae). Electron J Biotechnol 16(1):1–9. https://doi.org/10.2225/vol16-issue1-fulltext-6
Dawidar AM, Abdel-Mogib M, El-Naggar ME, Mostafa ME (2009) Acaricidal activity and chemical constituents of Hyoscyamus muticus against Teteranychus urticae Koch. Revista Latinoamericana de Química 37(1):45–55
Van Asperen K (1962) A study of housefly, esterases by means of sensitive colourimetric method. J Insect Physiol 8(4):401–416. https://doi.org/10.1016/0022-1910(62)90074-4
Simpson DR, Bull LD, Lindquist AD (1964) A semi micro techniques for estimation of cholinesterase activity in boll Weevil. Ann Entomol Soc Am 57(3):367–377. https://doi.org/10.1093/aesa/57.3.367
Hammerschmidt R, Nuckles F, Kuc J (1982) Association of enhanced peroxidase activity with induced systemic resistance of cucumber to Colletotrchum lagenarium. Physiol Plant Pathol 20(1):73–82. https://doi.org/10.1016/0048-4059(82)90025-X
Habig WH, Pabst MJ, Jakoby WB (1974) Glutathione-S-transferase the first enzymatic step in mercapturic acid formation. J Biol Chem 249(22):7130–7139
Bosch-Serra D, Rodríguez MA, Avilla J, Sarasúa MJ, Miarnau X (2021) Esterase, glutathione S-transferase and NADPH- cytochrome P450 reductase activity evaluation in Cacopsylla pyri L. (Hemiptera: Psyllidae) individual adults. Insects 12(4):329. https://doi.org/10.3390/insects12040329
Ishaaya I, Casida JE (1974) Dietary TH 6040 alters composition and enzyme activity of housefly larval cuticle. Pesticides Biochem Physiol 4(4):484–490. https://doi.org/10.1016/0048-3575(74)90073-X
Bradford MM (1976) A rapid and sensitive method for the quantitation of microgram quantities of proteins utilizing the principle of protein-dye binding. Anal Biochem 72(1–2):248–254. https://doi.org/10.1016/0003-2697(76)90527-3
Verma D, Kumar R, Irishi N, Namboothiri N (2013) Synthesis of withasomnines and their non-natural analogues from aldehydes and 4-nitro-1-butanol in three steps. J Org Chem 78:3482–3486. https://doi.org/10.1021/jo400207u
Bowden BF, Cambie RC, Parnell JC (1975) Constituents of the fruit of Pseudopanax arboreum (Araliaceae). Aust J Chem 28(1):91–107. https://doi.org/10.1071/CH9750091
Habib AA (1980) False-positive alkaloid reactions. J Pharm Sci 69(1):37–43. https://doi.org/10.1002/jps.2600690111
Velde VV, Lavie D (1981) New withanolides of biogenetic interest from Withania Somnifera. Phytochemistry 20(6):1359–1364. https://doi.org/10.1016/0031-9422(81)80039-8
Ahmad VU, Basha A (2006) Library of congress. Volume 1, spectroscopic data of steroid glycosides: cholestanes, ergostanes, withanolides, stigmastane. Springer Science+Business Media, New York
Atta-ur-Rahman, Shabbir M, Yousaf M, Qureshi S, Dur e-Shahwar, Naz A, Choudhary MI (1999) Three withanolides from Withania coagulans. Phytochemistry 52(7):1361–1364. https://doi.org/10.1016/S0031-9422(99)00416-1
Salamatullah AM (2022) Promising antioxidant and insecticidal properties of chemically characterized hydroethanol extract from Withania adpressa Coss ex. Batt. Horticulturae 8(698):1–11. https://doi.org/10.3390/horticulturae8080698
Ahmed KS, Idrees A, Majeed AZ, Majeed MI, Shehzad MZ, Ullah MI, Afzal A, Li J (2022) Synergized toxicity of promising plant extracts and synthetic chemicals against fall armyworm Spodoptera frugiperda (JE Smith) (Lepidoptera: Noctuidae) in Pakistan. Agronomy 12(6):1289. https://doi.org/10.3390/agronomy12061289
Gaur SK, Kumar K (2019) Sensitivity of tobacco caterpillar, Spodoptera litura, to extract from a medicinal plant, Withania somnifera. Int J Veg Sci 26(1):62–78. https://doi.org/10.1080/19315260.2019.1605556
Hyder M, Li Y, Wang M, Mao J, Zhang L (2022) Insecticidal activity of ethanol and aqueous extracts of medicinal plants against green Peach Aphid (Myzus Persicae). Appl Ecol Environ Res 20(3):2329–2342. https://doi.org/10.15666/aeer/2003_23292342
Hyder M, Li Y, Wang M, Mao J, Mari JM, Bukero A, Soomro HU, Bukero AA, Zhang L (2024) Insecticidal activity, chemical constituents of Trachyspermum ammi, Withania coagulans and Murraya koenigii ethanloic extracts against Bemisia tabaci. Brazilian J Biol 84:e260298. https://doi.org/10.1590/1519-6984.260298
Al-Ani EH, Al-Khazraji HI, Al-Shaibani MB (2022) The use of alkaloids as botanical insecticides, review. ACE Res J Microbiol Biotechnol 2(2):33–41
Patzke H, Schieber A (2018) Growth-inhibitory activity of phenolic compounds applied in an emulsifiable concentrate - ferulic acid as a natural pesticide against Botrytis cinerea. Food Res Int 113:18–23. https://doi.org/10.1016/j.foodres.2018.06.062
Brengues C, Hawkes NJ, Chandre F, McCarroll L, Duchon S, Guillet P, Manguin S, Morgan JC, Hemingway J (2003) Pyrethroid and DDT cross-resistance in Aedes aegypti correlated with novel mutations in the voltage-gated sodium channel gene. Med Vet Entomol 17(1):87–94. https://doi.org/10.1046/j.1365-2915.2003.00412.x
Intirach J, Junkum A, Lumjuan N, Chaithong U, Somboon P, Jitpakdi A, Riyong D, Champakaew D, Muangmoon R, Chansang A, Pitasawat B (2019) Biochemical effects of Petroselinum crispum (Umbellifereae) essential oil on the pyrethroid resistant strains of Aedes aegypti (Diptera: Culicidae). Insects 10(1):1–19. https://doi.org/10.3390/insects10010001
Enayati AA, Ranson H, Hemingwa J (2005) Insect glutathione transferases and insecticide resistance. Insect Mol Biol 14(1):3–8. https://doi.org/10.1111/j.1365-2583.2004.00529.x
Wu J, Li J, Zhang C, Yu X, Cuthbertson AGS, Ali S (2020) Biological impact and enzyme activities of Spodoptera litura (Lepidoptera: Noctuidae) in response to synergistic action of matrine and Beauveria brongniartii. Front Physiol 11:584405. https://doi.org/10.3389/fphys.2020.584405
Ding SY, Li HY, Li XF, Zhang ZY (2001) Effects of two kinds of transgenic poplar on protective enzymes system in the midgut of larvae of American white moth. J For Res 12:119–122. https://doi.org/10.1007/BF02867209
Cui C, Yang Y, Zhao T, Zou K, Peng C, Cai H, Wan X, Hou R (2019) Insecticidal activity and insecticidal mechanism of total saponins from Camellia oleifera. Molecules 24:4518. https://doi.org/10.3390/molecules24244518
Yang X, Zhang H (2012) Synergistic interaction of tea saponin with mancozeb against Pestalotiopsis theae. Crop Prot 40:126–131. https://doi.org/10.1016/j.cropro.2012.04.013
Maazouna AM, Hlela TB, Hamdib SH, Belhadja F, Ben Jemâab JM, Marzouki MN (2017) Screening for insecticidal potential and acetylcholinesterase activity inhibition of Urginea maritima bulbs extract for the control of Sitophilus oryzae (L.). J Asia-Pacific Entomol 20(3):752–760. https://doi.org/10.1016/j.aspen.2017.04.004
Acknowledgements
Great thanks for the kind help of Natural Products Lab, Faculty of Science, Faculty of agriculture, Mansoura University and Plant Protection Research Institute for accommodation.
Funding
Open access funding provided by The Science, Technology & Innovation Funding Authority (STDF) in cooperation with The Egyptian Knowledge Bank (EKB).
Author information
Authors and Affiliations
Contributions
The chemistry experiments were performed by AR. Experimental planning and data analysis were performed by ARE, MAT and HHE. ARE collected the plant materials and performed the insecticidal activity. Conceptualization and supervision performed by ARE, MAT and HHE. The manuscript was written by ARE. All authors reviewed the manuscript.
Corresponding author
Ethics declarations
Competing interests
The authors declare that they have no competing interests.
Additional information
Publisher's Note
Springer Nature remains neutral with regard to jurisdictional claims in published maps and institutional affiliations.
Supplementary Information
Additional file 1.
Supplemental data. Figure S1. 1H NMR (400 MHz) spectrum of withasomine 1 in CDCl3. Figure S1a. Expanded 1H NMR (400 MHz) spectrum of withasomine 1 in CDCl3. Figure S1b. Expanded 1H NMR (400 MHz) spectrum of withasomine 1 in CDCl3. Figure S2. 1H NMR (400 MHz) spectrum of methyl isoferulate 2 in CD3OD. Figure S2a. Expanded 1H NMR (400 MHz) spectrum of isoferulate 2 in CD3OD. Figure S3. 1H NMR (400 MHz) spectrum of sominone A 3 in CDCl3. Figure S3a. Expanded 1H NMR (400 MHz) spectrum of sominone A 3 in CDCl3. Figure S3b. Expanded 1H NMR (400 MHz) spectrum of sominone A 3 in CDCl3. Figure S4. 13C NMR (100 MHz) spectrum of sominone A 3 in CDCl3. Figure S4a. Expanded 13C NMR (100 MHz) spectrum of sominone A 3 in CDCl3. Figure S4b. Expanded 13C NMR (100 MHz) spectrum of sominone A 3 in CDCl3. Figure S5. H–H COSY spectrum of sominone A 3 in CDCl3. Figure S6. Edited HSQC spectrum of sominone A 3 in CDCl3. Figure S6a. Expanded Edited HSQC spectrum of sominone A 3 in CDCl3. Figure S6b. Expanded Edited HSQC spectrum of sominone A 3 in CDCl3. Figure S7. HMBC spectrum of sominone A 3 in CDCl3. Figure S7a. Expanded HMBC spectrum of sominone A 3 in CDCl3. Figure S7b. Expanded HMBC spectrum of sominone A 3 in CDCl3. Figure S8. UV spectrum of sominone A 3. Figure S9. IR spectrum of sominone A 3. Figure S10. HRESIMS spectrum of sominone A 3. Figure S11. 1H NMR (400 MHz) spectrum of coagulin Q 4 in CD3OD. Figure S11a. Expanded 1H NMR (400 MHz) spectrum of coagulin Q 4 in CD3OD. Figure S11b. Expanded 1H NMR (400 MHz) spectrum of coagulin Q 4 in CD3OD. Figure S12. 13C NMR (100 MHz) spectrum of coagulin Q 4 in CD3OD. Figure S12a. Expanded 13C NMR (100 MHz) spectrum of coagulin Q 4 in CD3OD. Figure S13. H–H COSY spectrum of coagulin Q 4 in CD3OD. Figure S14. Edited HSQC spectrum of coagulin Q 4 in CD3OD. Figure S14a. Expanded Edited HSQC spectrum of coagulin Q 4 in CD3OD. Figure S14b. Expanded Edited HSQC spectrum of coagulin Q 4 in CD3OD. Figure S15. HMBC spectrum of coagulin Q 4 in CD3OD. Figure S15a. Expanded HMBC spectrum of coagulin Q 4 in CD3OD. Figure S15b. Expanded HMBC spectrum of coagulin Q 4 in CD3OD. Figure S15C. Expanded HMBC spectrum of coagulin Q 4 in CD3OD.
Rights and permissions
Open Access This article is licensed under a Creative Commons Attribution 4.0 International License, which permits use, sharing, adaptation, distribution and reproduction in any medium or format, as long as you give appropriate credit to the original author(s) and the source, provide a link to the Creative Commons licence, and indicate if changes were made. The images or other third party material in this article are included in the article's Creative Commons licence, unless indicated otherwise in a credit line to the material. If material is not included in the article's Creative Commons licence and your intended use is not permitted by statutory regulation or exceeds the permitted use, you will need to obtain permission directly from the copyright holder. To view a copy of this licence, visit http://creativecommons.org/licenses/by/4.0/.
About this article
Cite this article
Ragab, A., Taher, M.A., El-Rafey, H.H. et al. Bioactive compounds from Withania somnifera dun and their toxicity against some piercing sucking pests. Appl Biol Chem 67, 29 (2024). https://doi.org/10.1186/s13765-024-00880-z
Received:
Accepted:
Published:
DOI: https://doi.org/10.1186/s13765-024-00880-z