Abstract
Objectives
Shiga toxin-producing Escherichia coli strains LAA-positive are important cause of human infection. The capability to adhere to epithelial cells is a key virulence trait, and genes codified in LAA pathogenicity island could be involved in the adhesion during the pathogenesis of LAA-positive STEC strains. Thus, our objectives were to compare hes-negative and hes-positive STEC strains in their adherence capability to epithelial cells (HEp-2) and to evaluate the expression levels of the hes, iha, and tpsA in the bacteria adhered and non-adhered to HEp-2 cells. These genes are encoded in LAA, and are virulence factors that participate in adhesion and autoaggregation.
Results
We could not observe differences between the adhesion of strains but also in the expression level of of hes, iha, and tpsA. Genes encoded in LAA contribute to the adhesion phenotype though the expression of STEC adhesins is a coordinated event that depends not only the strain but also on the environment as well as its genetic background. Therefore, the results of this study suggest that LAA ,the most prevalent PAI among LEE-negative STEC strains, plays a role in pathogenesis.
Similar content being viewed by others
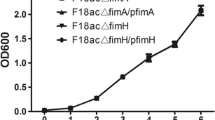
Introduction
Shiga toxin-producing Escherichia coli (STEC) are important pathogens responsible for foodborne diseases. The most virulent STEC strains isolated from human infections belong to the O157:H7 serotype, but other serotypes can cause several diseases [1, 2]. These pathogens are classified by the presence or absence of the Locus of Enterocyte Effacement (LEE) [3] LEE-positive strains can colonize the intestinal mucosa causing the attaching and effacing (A/E) lesion on the intestinal epithelium [3]. Nevertheless, the presence of LEE is not crucial for the pathogenesis of all the STEC strains since some LEE-negative, such as O113:H21 and O91:H21 have been associated with severe disease in humans [4, 5]. Despite the frequent isolation of STEC LEE-negative, the virulence potential and genetic profiles of clinical STEC isolates remain uncharacterized [5]. STEC LEE-negative could possess alternative virulence factors for adherence. Different pathogenicity islands (PAI) have been reported exclusively in LEE-negative STEC strains, such as Locus of Proteolysis Activity [6] and Locus of Adhesion and Autoaggregation (LAA), harboring diverse arrays of virulence factors [7].
LAA is a four-module structure: module I (hes), module II (iha and lesP), module III (pagC and tpsA), and module IV (agn43) [8, 9]. Montero, et al. [8] have reported the complete sequence of this PAI, in which other virulence factors participated in adhesion and autoaggregation. Hes, is a new member of the Heat-resistant agglutinin family (Hra Family), and this protein was named Hemagglutinin from Shiga toxin-producing E. coli, it is a virulence factor that participates in several phenotypes associated with colonization, including adhesion and autoaggregation [8]. Other virulence factors participating in adhesion and autoaggregation are also encoded in LAA, such as Iha an adherence-conferring protein distributed among LEE-negative and LEE-positive STEC strains [10]. TPS systems are TpsA for translocated proteins to the bacterial surface and TpsB for the transporter proteins. TPS systems participate in diverse virulence phenotypes such as adhesion, invasion, and autoaggregation [11, 12].
In light of these observations, our group was investigating genomic analysis, and PCR assays detected hes, iha, and tpsA in a high percentage of LEE-negative STEC strains from different origins and harboring diverse virulence repertoires [10, 13]. However, their expression levels have not been evaluated yet. The capability of bacteria to adhere and cause infection is associated with the expression of specific outer membrane proteins [14]. STEC genome plasticity provides the pathogen a great potential for genome expansion and niche adaptation [15, 16].
Genes codified in LAA could be involved in the adhesion during the pathogenesis of LAA-positive STEC strains, and little is known about the specific virulence factors that contribute to these pathogeneses. Thus, our objectives were to compare hes-negative and hes-positive STEC strains in their adherence capability to HEp-2 cells and to evaluate the expression levels of the hes, iha, and tpsA genes in the bacteria adhered and non-adhered to HEp-2 cells.
Materials and methods
Strains
A total of twenty O91 STEC were analyzed. All isolates are from the Immunochemistry and Biotechnology Laboratory (FCV-UNCPBA, Argentina) collection. The strains were previously isolated from cattle in Argentina and analyzed for the presence of genes encoding for Stx1/2 and their genetic diversity (Table 1) [7, 9, 17,18,19,20]. Seventeen O91 STEC were positive for hes (LAA complete), and three O91 STEC were negative for hes (LAA incomplete) [9]. E. coli HB101 was used as a negative control. Also, the mutant of the O91 STEC strain (VO 7-4-4) generated by the deletion of hes (O91Δhes) and E. coli HB101 transformed with pVB1_hes [8] were included.
Culture and inoculation to HEp-2 cells
The cell line was kindly provided by INTA Castelar, Buenos Aires, Argentina. HEp-2 cells were cultured in Minimal Essential Medium added with 10% of fetal calf serum (Natacor®) at 37 °C with 5% CO2. The cells were seeded in 24 well plates. The supernatant was discarded, and the plates were washed three times with phosphate-buffered saline (PBS). Then, 900 µl of fresh medium was added to each well. To inoculate the cells, each O91 strain was cultured in Luria Bertani broth at 37ºC for 18 h with shaking. One hundred ml of a culture adjusted by OD600 to a concentration of 106 CFU/mL was added to each well. Plates were incubated at 37ºC for 3 h. The monolayer was washed three times with PBS, and 100 µL per well of Trypsin-EDTA was added to recover HEp-2 cells with adhered bacteria. The cells disattached were recovered, and several dilutions of the cell suspensions were seeded onto MacConkey agar plates to quantify colonies corresponding to STEC. The experiments were performed in triplicate [21].
RNA extraction
The total RNA was extracted from three LAA-positive O91 STEC strains (VO 7-4-4, AP16.1, and TRN 5.1.1) and the E. coli HB101 pVB1_hes strain. The expression levels of hes, iha, and tpsA were evaluated in adhered and non-adhered in each strain selected from the HEp-2 cell assay. Briefly, the strains non-adhered were recovered from the supernatant after incubation with HEp-2 cells, and then the total RNA was extracted. As detailed above, the strains that adhered to HEp-2 cells were recovered when washing after adding Trypsin. The RNA of strains was extracted using a TRIzol reagent. Two separate experiments with at least three replicates each were conducted.
The treatment with DNase I was made to reduce gDNA contamination before reverse transcription; for this, 1 µg of RNA was incubated with 20 U of DNase I for 1 h at 37 °C followed by 12 min at 72 °C for inactivation. Afterward, cDNA was synthesized by using the High-Capacity cDNA Reverse Transcription Kit. RNA concentration and integrity were evaluated using a Spectrophotometer and electrophoresis, respectively.
Primer’s design
The primers used to detect iha and tpsA were described by Colello, et al. [9]. For the hes, the strains were previously characterized by PCR with specific primers [8]. The hes_RT primers were designed using the Primer3plus software (https://www.primer3plus.com/) (Table 2).
Quantitative real-time PCR analysis
The reactions consisted of 20 µL: 4 µl of 1/5 diluted cDNA, 10 µl of 2X SYBR Green master mix, and 300 nM of each primer. A no-template control was included in each run to assess for reagent contamination. The PCR was programmed for 40 cycles of 95 °C for 15 s followed by 60 °C for 1 min using the OneStep Plus Real-Time PCR System. The relative quantification was performed for the hes, iha, and tpsA to evaluate the expression of each strain adhered and non-adhered to epithelial cells. For each gene, the expression values were analyzed individually and independently, comparing adhered strains with their respective non-adhered strains. The data for the expression assays were detected in three separate experiments, and negative controls were included in each plate.
The reference gen tufA was used as the housekeeping [22]. The expression level of each gene was calculated by the relative fold change by the ΔΔCt threshold cycle (CT) method using the efficiency corresponding to each gene, which was obtained from the relative standard curves and determined by using the OneStep Plus Real-Time PCR System (Figure S1, S2, S3, and S4) [23]. Data sets were log10-transformed, and if the fold change is positive, the gene is upregulated; if the fold change is negative, it is downregulated [24].
Statistical analysis
Separate experiments were conducted to compare hes-negative and hes-positive STEC strains in their adherence capability to HEp-2, and a general linear model was fitted. A p-value of less than 0.05 was considered significant. The analyzes were performed with the R 3.6.3, lme4, and Multcomp programs [25, 26].
Results and discussion
In recent years, there has been an increasing interest in the study of LEE-negative STEC strains such as O91 because they have been associated with severe disease [5, 27]. The present and previous results have shown that STEC strains can adhere to HEp-2 cells [7, 21]. Some putative adhesins have been characterized to inquire about the adherence mechanisms of STEC strains to epithelial cells [12, 19, 28]. In addition, hes was detected in 40% and 46% of LEE-negative STEC strains isolated from humans and cattle [8, 9]. Therefore, to evaluate whether the hes gene can confer colonization-associated phenotypes, O91 strains and mutant strains were evaluated. We observed that the O91 strains could adhere to HEp-2 cells, whereas the E. coli HB101 strain, used as a negative control, could not (Fig. 1). In the O91Δhes STEC strain and in the hes negative-STEC strains, the capability to adhere to HEp-2 cell was variable. However, a non-significant difference was observed between STEC strains, whether positive to hes (LAA complete) or negative to hes (LAA incomplete) (p = > 0.05). Many different genes mediate the adherence between STEC and host cells and their expression could be affected in a complex way by physiological and environmental factors [29]. Even more, it has been established that STEC strains present varied adherence, different gene expression levels, and alternative genes performing similar functions in host cells. E coli HB101pVB1_hes showed an increase in the number of adherent bacteria compared with E. coli HB101 (p = < 0.05) (Table 1; Fig. 1). These results indicate that Hes is a functional protein in agreement with Montero, et al. [8] and could participate in the adherence to epithelial cells.
Adherence to HEp-2 by the O91 STEC strains
The adhered strains are expressed as the number of CFU per milliliter. O91Δhes STEC and E. coli HB101 pVB1_hes were used to compare their adherence with the adherence of the O91 STEC strains and the capability to adhere to HEp-2 cell was variable. *Green bars are hes positive strains and violet bars are hes negative strains
Since LAA-positive STEC strains carrying the hes, iha, and tpsA genes showed an adherence capability, we performed a quantitative real-time PCR on the total RNA extracted from the adhered and non-adhered bacteria to HEp-2 cells. Expression levels of each strain were expressed as fold change values relative to their non-adhered strain (Table 3, Figure S5 to S16). The amplification efficiencies of each gene were 98% (hes), 91.4% (iha), 92.03% (tpsA), and 99.6% (tufA) (Figure S1, S2, S3, S4).
According to the expression analysis of the hes gene, heterogeneous expression levels were detected among the adhered strains studied. In VO 7-4-4 and TRN 5.1.1 strains, the expression levels were lower than in the non-adhered ones, while the expression levels of AP 16.1 strains were higher than those in non-adhered strains. The expression levels of E. coli HB101pVB1_hes were higher than in the non-adhered strains, detecting a 1.6-fold increase in hes expression. Our study is the first to evaluate the expression of hes in O91 strains, suggesting that hes could participate in the adherence to epithelial cells. Moreover, Montero, et al. [8] demonstrated that sera from patients with HUS are reactive to hes, suggesting that this gene participates in human infection.
For iha gene, the VO 7-4-4 strain showed lower expression levels than the non-adhered strains. The expression levels of TRN 5.1.1 and AP 16.1 strains were higher than in the non-adhered strains (Table 3; Fig. 2). Some researchers have studied the mechanisms and functions of iha expression in STEC O157 in different assays [30, 31]. However, in a previous study, the alignment and phylogenetic analysis revealed that iha LAA had a lower sequence similarity regarding iha gene in STEC O157. These results suggest that iha genes from LEE-negative and LEE-positive STEC strains may have different origins. In addition, many STEC carries two or more copies of iha [10], but we did not know whether iha LAA are functional. For this reason, we made an expression assay of iha LAA to observe whether it confers adherence phenotype, whose results suggested that Iha LAA acts as an adhesin.
Results for tpsA were shown to be the same as for iha genes. The tpsA has different functions that could participate in the interactions of the bacteria with their host tissue. To colonize their animal hosts, STEC produces several adhesive structures and proteins, many of which are important virulence factors [16]. These adhesins have highly diverse structures, which implies that they physically interfere with each other by the presence of one surface structure obstructing the activity of another [14].
LAA was the most prevalent PAI among LEE-negative STEC strains, suggesting it plays an important role in pathogenesis [7, 9]. Genes encoded in LAA contribute to the adhesion phenotype, but it is not sufficient on their own to govern the adhesion of cells, and at least another factor show signs of cooperating in the adhesion. The expression of STEC adhesins is a coordinated event depending on the strain and its environment as well as its genetic background, a phenomenon named phenotypic plasticity [32].
Limitation
The main limitation of this study was the differences between STEC strains in the adherence to cells and the expression of genes encoded in LAA, because the strains vary in their genetic background, which allows them, in the absence of some genes, to replace their functions with others. Therefore, this result could be affected in a complex way by physiological and environmental factors. Further research is needed to understand better the mechanism of adhesion and simultaneous expression of genes in the pathogenicity of LEE-negative STEC, a subgroup responsible for illnesses in humans.
Data Availability
All data generated or analyzed during this study are included in this published article.
Abbreviations
- STEC:
-
Shiga toxin-producing Escherichia coli
- LEE:
-
Locus of Enterocyte Effacement
- A/E:
-
Attaching and effacing
- PAI:
-
Pathogenicity island
- LAA:
-
Locus of Adhesion and Autoaggregation
- CT:
-
Threshold cycle
References
Rivas M, Padola NL, Lucchesi PM, Masana M. Diarrheagenic Escherichia coli in Argentina. In: Pathogenic Escherichia coli in Latin America edn. Edited by Torres A; 2010: 348–392.
Torti JF, Cuervo P, Nardello A, Pizarro M. Epidemiology and characterization of Shiga toxin-producing Escherichia coli of hemolytic uremic syndrome in Argentina. Cureus 2021, 13(8).
Herold S, Paton JC, Paton AW. Sab, a novel autotransporter of locus of enterocyte effacement-negative Shiga-toxigenic Escherichia coli O113:H21, contributes to adherence and biofilm formation. Infect Immun. 2009;77(8):3234–43.
Bielaszewska M, Stoewe F, Fruth A, Zhang W, Prager R, Brockmeyer J, Mellmann A, Karch H, Friedrich AW. Shiga toxin, cytolethal distending toxin, and hemolysin repertoires in clinical Escherichia coli O91 isolates. J Clin Microbiol. 2009;47(7):2061–6.
Nüesch-Inderbinen M, Stevens MJ, Cernela N, Müller A, Biggel M, Stephan R. Distribution of virulence factors, antimicrobial resistance genes and phylogenetic relatedness among Shiga toxin-producing Escherichia coli serogroup O91 from human infections. Int J Med Microbiol. 2021;311(8):151541.
Schmidt H, Zhang W-L, Hemmrich U, Jelacic S, Brunder W, Tarr P, Dobrindt U, Hacker J, Karch H. Identification and characterization of a novel genomic island integrated at selC in locus of enterocyte effacement-negative, Shiga toxin-producing Escherichia coli. Infect Immunol. 2001;69(11):6863–73.
Montero DA, Canto FD, Velasco J, Colello R, Padola NL, Salazar JC, Martin CS, Oñate A, Blanco J, Rasko DA. Cumulative acquisition of pathogenicity islands has shaped virulence potential and contributed to the emergence of LEE-negative Shiga toxin-producing Escherichia coli strains. Emerg Microbes Infect. 2019;8(1):486–502.
Montero DA, Velasco J, Del Canto F, Puente JL, Padola NL, Rasko DA, Farfán M, Salazar JC, Vidal R. Locus of adhesion and autoaggregation (LAA), a pathogenicity island present in emerging Shiga toxin–producing Escherichia coli strains. Sci Rep. 2017;7(1):7011.
Colello R, Vélez MV, González J, Montero DA, Bustamante AV, Del Canto F, Etcheverría AI, Vidal R, Padola NL. First report of the distribution of locus of adhesion and autoaggregation (LAA) pathogenicity island in LEE-negative Shiga toxin-producing Escherichia coli isolates from Argentina. Microb Pathog. 2018;123:259–63.
Colello R, Krüger A, Velez MV, Del Canto F, Etcheverría AI, Vidal R, Padola NL. Identification and detection of iha subtypes in LEE-negative Shiga toxin-producing Escherichia coli (STEC) strains isolated from humans, cattle and food. Heliyon. 2019;5(12):e03015.
Jacob-Dubuisson F, Fernandez R, Coutte L. Protein secretion through autotransporter and two-partner pathways. BBA-Mol Cell Res. 2004;1694(1–3):235–57.
Jacob-Dubuisson F, Guérin J, Baelen S, Clantin B. Two-partner secretion: as simple as it sounds? Res Microbiol. 2013;164(6):583–95.
Velez MV, Colello R, Etcheverria SG, Etcheverría AI, Padola NL. Biofilm formation by LEE-negative Shiga toxin–producing Escherichia coli strains. Microb Pathog 2021, 157.
Ulett GC, Webb RI, Schembri MA. Antigen-43-mediated autoaggregation impairs motility in Escherichia coli. Microbiol. 2006;152(7):2101–10.
Brzuszkiewicz E, Gottschalk G, Ron E, Hacker J, Dobrindt U. Adaptation of pathogenic E. coli to various niches: genome flexibility is the key. In: Microb Pathogenomics. Volume 6, edn.: Karger Publishers; 2009: 110–125.
Carter MQ, Laniohan N, Lo C-C, Chain PS. Comparative Genomics Applied to systematically assess pathogenicity potential in Shiga Toxin-Producing Escherichia coli O145:H28. Microorganisms. 2022;10(5):866.
Sanz M, Viñas M, Parma A. Prevalence of bovine verotoxin-producing Escherichia coli in Argentina. Eur J Epidemiol. 1998;14(4):399–403.
Padola NL, Sanz ME, Blanco JE, Blanco M, Blanco J, Etcheverria AaI, Arroyo GH, Usera MA, Parma AE. Serotypes and virulence genes of bovine shigatoxigenic Escherichia coli (STEC) isolated from a feedlot in Argentina. Vet Microbiol. 2004;100(1):3–9.
Hernandez LB, Cadona JS, Christensen M, Fernández D, Padola NL, Bustamante AV, Sanso AM. Virulence genes and genetic diversity assessment of Shiga toxin-producing Escherichia coli O91 strains from cattle, beef and poultry products. Microb Pathog. 2018;125:463–7.
Fernández D, Irino K, Sanz M, Padola N, Parma A. Characterization of Shiga toxin-producing Escherichia coli isolated from dairy cows in Argentina. Lett Appl Microbiol. 2010;51(4):377–82.
Etcheverría A, Arroyo G, Alzola R, Parma A. Reduction of adherence of E. coli O157: H7 to HEp-2 cells and to bovine large intestinal mucosal explants by colicinogenic E. coli. ISRN Microbiol 2011, 2011.
de Sablet T, Bertin Y, Vareille M, Girardeau J-P, Garrivier A, Gobert AP, Martin C. Differential expression of stx2 variants in Shiga toxin-producing Escherichia coli belonging to seropathotypes a and C. Microbiology. 2008;154(1):176–86.
Pfaffl MW. A new mathematical model for relative quantification in real-time RT–PCR. Nucleic Acids Res. 2001;29(9):e45–5.
Livak KJ, Schmittgen TD. Analysis of relative gene expression data using real-time quantitative PCR and the 2 – ∆∆CT method. Methods. 2001;25(4):402–8.
Hothorn T, Bretz F, Westfall P. Simultaneous inference in general parametric models. J Math Meth Biosci. 2008;50(3):346–63.
Bates D, Mächler M, Bolker B, Walker S. Fitting linear mixed-effects models using lme4. J Stat Softw. 2014;6:1–48.
Steyert SR, Sahl JW, Fraser CM, Teel LD, Scheutz F, Rasko DA. Comparative genomics and stx phage characterization of LEE-negative Shiga toxin-producing Escherichia coli. Front Cell Infect Microbiol 2012, 2.
Cadona JS, Burgán J, González J, Bustamante AV, Sanso AM. Differential expression of the virulence gene nleB among Shiga toxin-producing Escherichia coli strains. Heliyon. 2020;6(6):e04277.
Tran SL, Billoud L, Lewis SB, Phillips AD, Schüller S. Shiga toxin production and translocation during microaerobic human colonic infection with Shiga toxin-producing E. coli O157:H7 and O104:H4. Cell Microbiol. 2014;16(8):1255–66.
Rashid RA, Tarr PI, Moseley SL. Expression of the Escherichia coli IrgA homolog adhesin is regulated by the ferric uptake regulation protein. Microb Pathog. 2006;41(6):207–17.
Léveillé S, Caza M, Johnson JR, Clabots C, Sabri M, Dozois CM. Iha from an Escherichia coli urinary tract infection outbreak clonal group a strain is expressed in vivo in the mouse urinary tract and functions as a catecholate siderophore receptor. Infect Immun. 2006;74(6):3427–36.
Roberfroid S, Vanderleyden J, Steenackers H. Gene expression variability in clonal populations: causes and consequences. Crit Rev Microbiol. 2016;42(6):969–84.
Acknowledgements
The authors thank Analía Gandur for correcting the manuscript.
Funding
This work was supported by PICT 2018–03599 and CIC from Argentina.
Author information
Authors and Affiliations
Contributions
Author Contributions: Conceptualization, R.C., M.V.V, and N.L.P.; Funding acquisition, R.C. and N.L.P.; Investigation, R.C. and M.V.V.; Methodology, R.C., M.R. and M.V.V.; Supervision, N.L.P. and A.I.E.; Validation, R.C., M.V.F., and M.V.V.; Visualization, R.C., M.V.V., D.M., A.I.E., and N.L.P.; Writing-original draft, R.C. and M.V.V.; Writing-review and editing, R.C., M.V.V., M.V.F., M.R., D.M., R.V., A.I.E. and N.L.P.
Corresponding author
Ethics declarations
Ethics approval and consent to participate
Not applicable for that section.
Consent for publication
Not applicable for that section.
Competing interests
The authors declare that they have no competing interests.
Additional information
Publisher’s Note
Springer Nature remains neutral with regard to jurisdictional claims in published maps and institutional affiliations.
Electronic supplementary material
Below is the link to the electronic supplementary material.
Rights and permissions
Open Access This article is licensed under a Creative Commons Attribution 4.0 International License, which permits use, sharing, adaptation, distribution and reproduction in any medium or format, as long as you give appropriate credit to the original author(s) and the source, provide a link to the Creative Commons licence, and indicate if changes were made. The images or other third party material in this article are included in the article’s Creative Commons licence, unless indicated otherwise in a credit line to the material. If material is not included in the article’s Creative Commons licence and your intended use is not permitted by statutory regulation or exceeds the permitted use, you will need to obtain permission directly from the copyright holder. To view a copy of this licence, visit http://creativecommons.org/licenses/by/4.0/. The Creative Commons Public Domain Dedication waiver (http://creativecommons.org/publicdomain/zero/1.0/) applies to the data made available in this article, unless otherwise stated in a credit line to the data.
About this article
Cite this article
Colello, R., Vélez, M.V., Farias, M.V.N. et al. Expression of hes, iha, and tpsA codified in locus of adhesion and autoaggregation and their involvement in the capability of shiga toxin-producing Escherichia coli strains to adhere to epithelial cells. BMC Res Notes 16, 163 (2023). https://doi.org/10.1186/s13104-023-06433-9
Received:
Accepted:
Published:
DOI: https://doi.org/10.1186/s13104-023-06433-9