Abstract
Background
Eave spaces are major entry points through which malaria vectors enter houses. Interventions that target mosquitoes at the eaves have recently been developed. However, most of these interventions are based on insecticides for which resistance has been reported. Here we evaluated the efficacy of mosquito electrocuting eave tubes (MEETs) against Anopheles gambiae sensu stricto (An. gambiae s.s.) and Anopheles funestus s.s. under semi-field conditions.
Methods
Experiments were conducted in two semi-field chambers, each containing one experimental hut. Six electrocuting eave tubes were installed in each hut to assess their impact on laboratory-reared An. gambiae s.s. and An. funestus s.s.. Each species was assessed separately over 10 nights by releasing 200 unfed females per night into each chamber. One volunteer slept in each hut from 7 p.m. to 5 a.m. Mosquitoes were collected indoors and outdoors using mouth and Prokopack aspirators.
Results
The placement of MEETs significantly reduced the nightly An. gambiae s.s. indoor and outdoor biting, by 21.1% and 37.4%, respectively. Indoor-biting An. funestus s.s. were reduced by 87.5% while outdoor-biting numbers of An. funestus s.s. declined by 10.4%.
Conclusions
MEETs represent a promising tool for controlling mosquitoes at the point of house entry. Further validation of their potential under natural field conditions is necessary. Several advantages over insecticide-based eave tubes are indicated and discussed in this article.
Graphical Abstract

Similar content being viewed by others
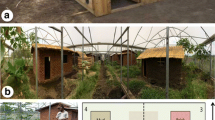
Background
Malaria remains a major public health concern, with about 249 million reported cases of malaria and 608,000 deaths due to this disease in 2022 [1]. The burden is disproportionately higher in sub-Saharan Africa where about 94% and 95% of all global cases and deaths occurred in 2022, respectively [1]. Despite the pivotal role played by vector control measures such as insecticide-treated nets (ITNs) and indoor residual spraying (IRS) in reducing malaria cases and deaths [2], emerging challenges such as insecticide resistance and shifts in mosquito biting behaviour threaten the efficacy of these interventions [3,4,5,6]. The slow-down in the gains in recent years [1] suggests that even with these tools, malaria eradication is unlikely.
In response to the limitations of current vector control strategies, there is an urgent need for innovative and sustainable approaches to malaria prevention. Developing interventions that are not only effective against mosquito vectors but also address the challenges of conventional methods is essential for long-term success in malaria control and ultimately the elimination of malaria [7, 8].
House improvement has been demonstrated to offer protection against malaria [9, 10] and is one of the most preferred interventions for malaria control in southern Tanzania [11, 12]. Several field studies have demonstrated that house design is one of the factors influencing the abundance of indoor malaria vector densities and malaria transmission [13,14,15]. Simple house modifications can significantly reduce malaria transmission, yet a previous study found that communities in rural Tanzania were unable to improve their houses due to financial constraints and competing household priorities [16]. Poor housing is not only associated with high incidences of malaria transmission but also with other numerous infectious diseases, notably insect-borne diseases such as filariasis and arboviruses, which are major public health and economic concerns in most African countries [9, 13, 14, 17, 18]. In Tanzania, approximately 93% of the population lives in areas at risk of malaria [19], which could be largely preventable by, among other measures, living in proper housing. However, housing is not included in control programmes due to high costs and various challenges [12]. Nonetheless, the major malaria vectors in Africa mostly prefer to feed on humans and bite and rest inside houses [20, 21].
Eave tubes have been introduced as a promising alternative for malaria vector control [22]. The method involves closing the eave spaces while leaving openings for eave tubes, thereby facilitating ventilation in the house while channeling human odour outdoors to attract mosquitoes into the tubes [22]. The tubes are fixed at eave height to target mosquitoes as they attempt to enter houses based on the observation that malaria vectors often use eaves as house entry points [23,24,25]. As mosquitoes attempt to enter the houses, they come into contact with netting inside the tubes that is treated with insecticides. The insecticide, in powder form, is bound to the netting using electrostatic forces, which increases mosquito exposure and ensures high efficacy even against pyrethroid -resistant mosquitoes [26]. While the use of eave tubes alone, without insecticides, provides a physical barrier against mosquitoes and thus provides protection to the household, the addition of insecticides offers communal protection by effectively killing them [27, 28].
Eave tubes were found to reduce mosquito densities in semi-field studies conducted in Tanzania [28], Kenya [29] and Ivory Coast [30]. In a cluster-randomized trial in Ivory Coast, a combination of house screening and eave tubes significantly reduced malaria incidence [31, 32], as well as the entomological inoculation rate [32]. However, despite the efficacy of this intervention in reducing and killing mosquitoes, inevitably problems with insecticides will arise. Frequent replacement of eave tube inserts with insecticides boosts labour costs and will ultimately cause resistance. Therefore, non-insecticidal approaches are likely to be more sustainable. These can be integrated in long-term vector control strategies without causing resistance buildup in mosquito populations by eliminating selection pressure.
Mosquito electrocuting eave tubes (MEETs) represent a promising alternative in the fight against malaria without relying on insecticides. The MEET utilizes an electrocuting grid installed inside a standard 6-inch (15.24 cm) polyvinyl chloride (PVC) tube that is installed at eave height while other spaces are sealed. In this study, we assessed the efficacy of MEETs against Anopheles gambiae sensu stricto (An. gambiae s.s.) and Anopheles funestus s.s. in a semi-field setting. The results are discussed in the context of utilizing MEETs for and its contribution to sustainable vector control.
Methods
Description of the study facility
This study was conducted near Ifakara, south-east Tanzania in the ‘mosquito city’ facility, which is located in Kining’ina village, approximately 6 km north of the town of Ifakara (8.10800°S, 36.66585°E). A semi-field system with a total surface area of 553 m2 was utilized (Fig. 1A). The semi-field system comprises of six chambers/compartments, each measuring 9.6 × 9.6 × 4.5 m (length × width × height). Two semi-field chambers within one semi-field system were used. Inside each semi-field chamber, experimental huts measuring 3.1 × 2.7 m were constructed in which the MEETs were installed (Fig. 1B). Each experimental hut had six MEETs. More details on the semi-field systems and experimental huts can be found in previous publications [33,34,35].
Semi-field set-up for the assessment of the efficacy of mosquito electrocuting eave tubes (MEETs). A An experimental hut fitted with 6 MEETs (3 on the front and three at the back). B Magnified view of a MEET with a red dashed circle showing some electrocuted mosquitoes. C A net fitted in the inner side of the MEET trap. D Wiring layout of MEET traps
Mosquito electrocuting eave tubes
The MEETs were made up of standard 6-inch (15.24 cm) PVC tubes, each equipped with an electrocuting grid tightly fitted against the rim of the PVC tube. Grids were removed from commercially available mosquito lamps (model IK GP02; input AC220-240 V 50 Hz; output 800-1000 V through a high-voltage transformer, Tronic, China). The MEETs were serially connected to the mains and were operated permanently by fixing the press button in the ‘on’ mode. Thus, a hut could have all of its grids switched ‘on’ or all of the grids switched ‘off’. To prevent mosquitoes from passing the electrocuting grid and entering the hut, pieces of untreated bednet material were used to cover the tube outlet inside the hut.
Mosquitoes
Female An. gambiae s.s. (Ifakara strain) and An. funestus s.s. (FUMOZ strain) reared in the laboratory and maintained at the Ifakara insectaries were used at age 3 to 6 days in the experiments. Larvae were fed on Tetramin fish food (Tetra Werke, Melle, Germany) and maintained at 26–28 °C. Adults were fed ad libitum with a 10% glucose solution, and the insectary was maintained under 12:12-h light/dark conditions.
Release and recapture of mosquitoes
Each night a total of 200 blood-naive females were released in the semi-field chambers at 7 p.m.−50 mosquitoes in each of the four corners of the semi-field chamber. The mosquitoes had been starved for 6 h prior to the start of the experiment by removing the glucose solution from the insectary. The experiment was conducted for 10 days for each species, with each species released separately.
At 5 a.m., mosquitoes were collected indoors and outdoors using Prokopack and mouth aspirators. The assumption was made that the grids would kill mosquitoes attempting to enter the hut through the PVC tubes. Therefore, the number of live mosquitoes available for collection in the morning was expected to be lower when the grids were ‘on’.
Efficacy of MEETs against An. gambiae s.s. and An. funestus s.s.
A comparative study was undertaken in the semi-field system. Two chambers, each with one experimental hut, were used. Six MEETs were fixed in each hut, three on each side. They were installed with the bottom of the pipe aligned with the wall, while the top part was fitted at a slight angle to guide odours coming out of the hut. All other openings (including eaves) were sealed as much as possible with mud so that airflow only passed through the eaves.
Two volunteers slept in each experimental hut from 7 p.m. to 5 a.m.. Each compartment alternated between having all grids turned on for 5 days and all grids turned off for 5 days. Volunteers were rotated randomly to ensure they slept in each hut for 5 days. They were not informed about whether the grids were on or off. Mosquito collection was done by another team, which comprised two volunteers who were also unaware of whether the grids were turned on or off.
Data analysis
Data were analysed using R software (version 4.1.2) [36]. Data for each species was analysed separately. Descriptive statistics were analysed using the dplyr package [37]. Graphics were generated using ggplot2 [38]. The efficacy of MEETs (percentage reduction in mosquitoes recaptured) was calculated as: \(\frac{Mean\, capture \,in \,control-Mean \,capture \,in \,treatment}{Mean \,capture \,in \,control} \times \) 100, where ‘Control’ was the number of mosquitoes recaptured in the ‘MEETs OFF’ chamber, and ‘Treatment’ was the number of mosquitoes recaptured in the ‘MEETs ON’ chamber. The chi-square test of proportions was used to assess whether there was any statistically significant difference between the percentage reduction of mosquito species released, intervention and location. Lastly, to assess the efficacy of MEETs, a generalized linear mixed model (GLMM) was deployed, the number of mosquitoes recaptured was added as a response variable, while the intervention (ON or OFF) was added as a fixed factor. Volunteer identification (ID), chamber ID and experimental day were added as random factors in the models. Model selection for the inclusion of the random effects (volunteers ID, chamber ID and experimental day) was performed using the Akaike information criterion (AIC); the model with the lowest AIC value was considered to be the best model. the odds ratio (OR) was reported along with the respective 95% confidence interval (CI).
Results
Mosquito recaptures
A total of 8000 female Anopheles mosquitoes were released during the 20-day experiment, of which half were An. gambiae s.s., with An. funestus s.s. accounting for the other half. A total of 2259 (28% of the released) mosquitoes were recaptured from all chambers, irrespective of the intervention and mosquito species released into the chamber. A similar trend in the recapture rate was observed for both species released. For An. gambiae s.s., the recapture rate was lower for the treatment (MEETs ON) chamber than for the control (MEETs OFF) chamber: 28.3% (n = 566 of the released mosquitoes recaptured) versus 44% (n = 889 of the released mosquitoes recaptured), respectively. Similarly, for An. funestus s.s., the recapture rate was lower for the treatment chamber compared with the control chamber: 19.0% (n = 379 of the released mosquitoes) versus 21.5% (n = 430 of the released mosquitoes).
Despite similar trends in recapture rates, there was a significant difference between the species released and location (χ2 = 151.06, df = 1, P < 0.001). The recapture rate of indoor-biting mosquitoes showed a similar catch in the deployed interventions between mosquito species released (χ2 = 2.47, df = 1, P = 0.116). The mean (± standard error [SE] indoor recapture rate for An. gambiae s.s. was 4.5 ± 0.5 and 5.7 ± 1.6 per day for the treatment and control chambers, respectively. Similarly, the mean indoor recapture rate for An. funestus s.s. sensu stricto was 0.1 ± 0.1 and 0.8 ± 0.6 per day for the treatment and control chambers, respectively (Fig. 2).
Assessing the efficacy of mosquito electrocuting eave tubes (MEETs). A Comparison of different recapture rates of Anopheles gambiaes.s. with location. B Comparison of different recapture rate of Anopheles funestus s.s. with location. Black open circles denote number of mosquitoes recaptured nightly; red circles with error bars denote mean recaptures ± standard error. s.s., Sensu stricto
In comparison, there was a significant difference in the mean outdoor recapture rate across mosquito species following the deployment of the intervention (χ2 = 15.44, df = 1, P < 0.001). For An. gambiae s.s., the mean (± SE) outdoor recapture rate was 52.1 ± 7.2 and 83.2 ± 12.7 per day for the treatment and control chambers, respectively. Similarly, the mean outdoor recapture rate for An. funestus s.s. sensu stricto (An. funestus s.s.) was 37.8 ± 5.2 and 42.2 ± 6.5 per day for the treatment and control chambers, respectively (Fig. 2).
Efficacy of MEETs against An. gambiae s.s. and An. funestus s.s.
Placement of MEETs reduced the number of female mosquitoes attempting to bite human volunteers in the treatment chamber. The number of indoor- and outdoor-biting An. gambiae females was reduced by 21.1% and 37.4% in the treatment chambers (MEETs ON), respectively, relative to the control chambers (MEETs OFF). The observed reduction was statistically significant for both indoor- and outdoor-biting mosquitoes (GLMM: OR = 0.82, P < 0.001 and OR = 0.63, P < 0.001, respectively; Table 1). Similarly, the number of indoor- and outdoor-biting An. funestus s.s. was reduced by 87.5% and 10.42% in the treatment chambers (MEETs ON), respectively, relative to the control chambers. The observed reduction was marginally statistically insignificant for indoor-biting mosquitoes and not significant for outdoor-biting mosquitoes (GLMM: OR = 0.12, P = 0.052 and GLMM: OR = 0.88, P = 0.086, respectively; Table 1).
Discussion
Poor house designs in sub-Saharan Africa continue to contribute to malaria transmission [9, 10, 15, 39,40,41]. One key link in this interaction is the presence of an eave space opening created by household owners to reduce indoor heat stress, but which allows the entry of mosquitoes. Studies have shown that Anopheles mosquitoes utilize eave space openings as their main entry point into houses [23,24,25, 42,43,44], a behaviour that has formed the basis for several innovative interventions that will kill or repel mosquitoes on their way into the house. Examples of such interventions include screens treated with biological control agents (e.g. entomopathogenic fungi) [45], insecticidal eave curtains [46, 47], repellent-treated eave ribbons [48,49,50,51,52], insecticide-treated eave tubes [22, 27,28,29,30], among others. All of these interventions have been shown to effectively control malaria vectors in sub-Saharan Africa. Despite their effectiveness, however, the implementation of these tools is hindered by their dependency on chemicals, to which mosquitoes will inevitably develop resistance. Therefore, complementary non-insecticidal approaches in eave spaces are needed that will provide additional benefits by eliminating selection pressure for insecticide resistance (although it is appreciated that active avoidance of tube entry will ultimately pose a form of behavioural resistance).
The semi-field system in which houses are created to mimic the natural village setting provides a real-life scenario to evaluate the efficacy of MEETs under controlled environments. The main finding of this study was a distinct reduction of recaptures of both An. gambiae s.s. and An. funestus s.s. within the ‘MEETs-ON’ chambers. Given the innate behaviours of both mosquito species to feed (endophagy) and rest indoors afterwards (endophily), it may be assumed that tube entry occurred while the mosquitoes were host-seeking and that mosquito electrocution upon contact with the grid caused the decline in numbers recaptured. Endophagy is more pronounced for An. funestus s.s than for An. gambiae s.s., which caused the likely higher reduction of numbers recaptured indoors, with the caveat that numbers were very low indeed.
To the best of our knowledge, this is the first study to directly assess the efficacy of MEETs on mosquitoes. While the objectives were broadly achieved, a number of limitations are noted. First, the study used an indirect measure of reduction in the number of mosquitoes. We could not directly count electrocuted mosquitoes but rather counted recaptured mosquitoes in the chamber. However, this approach has been used in previous studies to assess the effectiveness of eave tubes in Tanzania [28], Kenya [29] and Ivory Coast [30]. Moreover, the Kenya study, in which eave tubes were treated with fluorescent dye that was transferred to mosquitoes upon contact and showed on their bodies after recapture, provided proof of tube entry and contact. Using videography, the same has been shown in open-field studies in Tanzania, where the median contact time of mosquitoes with (treated) netting was 71 s [44]. Second, this study was conducted in a semi-field setting using laboratory-reared mosquitoes; consequently, field trials are required to determine if these efficacies can also be achieved under real-world conditions. A third shortcoming in our study was the large variation in the numbers of recaptured mosquitoes in both the treatment and control chambers during the experimental nights; although the differences were significant, these would likely have been more dramatic if recapture rates had been more consistent, which did occur during previous trials with insecticides in Ifakara [28].
Given that electrocuting grids have been used to study odour-mediated behaviour of mosquitoes [53] and have been considered to be incorporated in traps [54] and as an alternative to human landing catches [55], we assume that approaching mosquitoes were not repelled by the MEETs [56].
Future use of MEETs will likely require their use in areas where houses lack electricity. Given the rapid developments in solar technology, solar conversion efficiency and decreasing costs, it will be relatively easy to run MEETs off-grid in this manner. Moreover, access to solar energy in communities without access to electricity may enhance acceptance of mosquito control tools. Matowo et al. [57] found increased community acceptance of mosquito landing boxes in Tanzania by simply adding solar power to the outdoor devices that rural communities could use for lighting their homes and charging their mobile phones. A trial with odour-baited traps near houses in Kenya achieved the same results through solar panels [58]. Similar approaches could be adopted for scaling up the use of MEETs in similar settings. Considering that the cost of MEETs will be crucial for scalability and accessibility, mass production will be necessary. Fortunately, the electronics of MEETs are already being mass-produced for ‘mosquito rackets’ and are easy to obtain for less than US$1. However, open-field evaluations of MEETs are still necessary in order to obtain a satisfactory prototype for further development.
Conclusions
MEETs can effectively kill mosquitoes and thus significantly reduce their densities and thereby malaria transmission. MEETs not only provide household protection but may also provide communal protection by mass killing, but this aspect needs to be confirmed in open field trials.
Availability of data and materials
The data supporting the findings of the study must be available within the article and/or its supplementary materials, or deposited in a publicly available database.
Abbreviations
- AIC:
-
Akaike information criterion
- CI:
-
Confidence interval
- GLMM:
-
Generalized linear mixed model
- IRS:
-
Indoor residual spraying
- ITNs:
-
Insecticide-treated nets
- MEET:
-
Mosquito electrocuting eave tube
- OR:
-
Odds ratio
- PVC:
-
Polyvinyl chloride
References
WHO. World malaria report 2023. 2023. Geneva: WHO.https://www.who.int/teams/global-malaria-programme/reports/world-malaria-report-2023.
Bhatt S, Weiss DJ, Cameron E, Bisanzio D, Mappin B, Dalrymple U, et al. The effect of malaria control on Plasmodium falciparum in Africa between 2000 and 2015. Nature. 2015;526:207–11.
Hemingway J, Ranson H. Insecticide resistance in insect vectors of human disease. Annu Rev Entomol. 2000;45:79–102.
Ranson H, Lissenden N. Insecticide resistance in African Anopheles mosquitoes: a worsening situation that needs urgent action to maintain malaria control. Trends Parasitol. 2016;32:187–96.
Durnez L, Coosemans M. Residual transmission of malaria: an old issue for new approaches. In: Manguin S, editor. Anopheles mosquitoes: new insights into malaria vectors. Rijeka: InTech; 2013. p. 671–704.
Carnevale P, Manguin S. Review of issues on residual malaria transmission. J Infect Dis. 2021;223:S61-80.
Govella NJ, Ferguson H. Why use of interventions targeting outdoor biting mosquitoes will be necessary to achieve malaria elimination. Front Physiol. 2012;3:199.
Russell TL, Beebe NW, Cooper RD, Lobo NF, Burkot TR. Successful malaria elimination strategies require interventions that target changing vector behaviours. Malar J. 2013;12:56.
Tusting LS, Bottomley C, Gibson H, Kleinschmidt I, Tatem AJ, Lindsay SW, et al. Housing improvements and malaria risk in sub-Saharan Africa: a multi-country analysis of survey data. PLoS Med. 2017;14:e1002234.
Tusting LS, Gething PW, Gibsoni HS, Greenwoodi B, Knudseni J, Lindsayi SW, et al. Housing and child health in sub-Saharan Africa: a cross-sectional analysis. PLoS Med. 2020;17:e1003055.
Bofu RM, Santos EM, Msugupakulya BJ, Kahamba NF, Swilla JD, Njalambaha R, et al. The needs and opportunities for housing improvement for malaria control in southern Tanzania. Malar J. 2023;22:69.
Finda MF, Christofides N, Lezaun J, Tarimo B, Chaki P, Kelly AH, et al. Opinions of key stakeholders on alternative interventions for malaria control and elimination in Tanzania. Malar J. 2020;19:164.
Ghebreyesus TA, Haile M, Witten KH, Getachew A, Yohannes M, Lindsay SW, et al. Household risk factors for malaria among children in the Ethiopian highlands. Trans R Soc Trop Med Hyg. 2000;94:17–21.
Howell PI, Chadee DD. The influence of house construction on the indoor abundance of mosquitoes. J Vector Ecol. 2007;32:69–74.
Ogoma SB, Lweitoijera DW, Ngonyani H, Furer B, Russell TL, Mukabana WR, et al. Screening mosquito house entry points as a potential method for integrated control of endophagic filariasis, arbovirus and malaria vectors. PLoS Negl Trop Dis. 2010;4:e773.
Kaindoa EW, Finda M, Kiplagat J, Mkandawile G, Nyoni A, Coetzee M, et al. Housing gaps, mosquitoes and public viewpoints: a mixed methods assessment of relationships between house characteristics, malaria vector biting risk and community perspectives in rural Tanzania. Malar J. 2018;17:298.
Konradsen F, Amerasinghe P, Van Der Hoek W, Amerasinghe F, Perera D, Piyaratne M. Strong association between house characteristics and malaria vectors in Sri Lanka. Am J Trop Med Hyg. 2003;68:177–81.
Tusting LS, Willey B, Lines J. Building malaria out: improving health in the home. Malar J. 2016;15:320.
Mitchell CL, Ngasala B, Janko MM, Chacky F, Edwards JK, Pence BW, et al. Evaluating malaria prevalence and land cover across varying transmission intensity in Tanzania using a cross-sectional survey of school-aged children. Malar J. 2022;21:80.
Seyoum A, Sikaala CH, Chanda J, Chinula D, Ntamatungiro AJ, Hawela M, et al. Human exposure to anopheline mosquitoes occurs primarily indoors, even for users of insecticide-treated nets in Luangwa Valley, South-east Zambia. Parasit Vectors. 2012;5:101.
Smith A. The preferential indoor resting habits of Anopheles gambiae in the Umbugwe area of Tanganyika. East Afr Med J. 1962;39:631–5.
Knols BGJ, Farenhorst M, Andriessen R, Snetselaar J, Suer RA, Osinga AJ, et al. Eave tubes for malaria control in Africa: an introduction. Malar J. 2016;15:404.
Lindsay SW, Snow RW. The trouble with eaves; house entry by vectors of malaria. Trans R Soc Trop Med Hyg. 1988;82:645–6.
Spitzen J, Koelewijn T, Mukabana WR, Takken W. Visualization of house-entry behaviour of malaria mosquitoes. Malar J. 2016;15:233.
Mburu MM, Juurlink M, Spitzen J, Moraga P, Hiscox A, Mzilahowa T, et al. Impact of partially and fully closed eaves on house entry rates by mosquitoes. Parasit Vectors. 2018;11:383.
Andriessen R, Snetselaar J, Suer RA, Osinga AJ, Deschietere J, Lyimo IN, et al. Electrostatic coating enhances bioavailability of insecticides and breaks pyrethroid resistance in mosquitoes. Proc Natl Acad Sci USA. 2015;112:12081–6.
Okumu F. The paradigm of eave tubes: scaling up house improvement and optimizing insecticide delivery against disease-transmitting mosquitoes. Malar J. 2017;16:207.
Sternberg ED, Ng’habi KR, Lyimo IN, Kessy ST, Farenhorst M, Thomas MB, et al. Eave tubes for malaria control in Africa: initial development and semi-field evaluations in Tanzania. Malar J. 2016;15:447.
Snetselaar J, Njiru BN, Gachie B, Owigo P, Andriessen R, Glunt K, et al. Eave tubes for malaria control in Africa: Prototyping and evaluation against Anopheles gambiae s.s. and Anopheles arabiensis under semi-field conditions in western Kenya. Malar J. 2017;16:276.
Oumbouke WA, Tia IZ, Barreaux AMG, Koffi AA, Sternberg ED, Thomas MB, et al. Screening and field performance of powder-formulated insecticides on eave tube inserts against pyrethroid resistant Anopheles gambiae s.l.: an investigation into “actives” prior to a randomized controlled trial in Côte d’Ivoire. Malar J. 2018;17:374–374.
Cook J, Sternberg E, Aoura CJ, N’Guessan R, Kleinschmidt I, Koffi AA, et al. Housing modification for malaria control: impact of a “lethal house lure” intervention on malaria infection prevalence in a cluster randomised control trial in Côte d’Ivoire. BMC Med. 2023;21:168.
Sternberg ED, Cook J, Alou LPA, Assi SB, Koffi AA, Doudou DT, et al. Impact and cost-effectiveness of a lethal house lure against malaria transmission in central Côte d’Ivoire: A two-arm, cluster-randomised controlled trial. Lancet. 2021;397:805–15.
Ng’Habi KRN, Mwasheshi D, Knols BGJ, Ferguson HM. Establishment of a self-propagating population of the African malaria vector Anopheles arabiensis under semi-field conditions. Malar J. 2010;9:356.
Ferguson HM, Ng’habi KR, Walder T, Kadungula D, Moore SJ, Lyimo I, et al. Establishment of a large semi-field system for experimental study of African malaria vector ecology and control in Tanzania. Malar J. 2008;7:158.
Lwetoijera D, Harris C, Kiware S, Dongus S, Devine GJ, McCall PJ, et al. Effective autodissemination of pyriproxyfen to breeding sites by the exophilic malaria vector Anopheles arabiensis in semi-field settings in Tanzania. Malar J. 2014;13:161.
R Core Team. R: A language and environment for statistical computing. 2017. Vienna: R Foundation for Statistical Computing. https://www.r-project.org/. Accessed 3 Feb 2022.
Wickham H, François R, Henry L, Müller K, Vaughan D. dplyr: a grammar of data manipulation [Internet]. 2023. https://github.com/tidyverse/dplyr. Accessed 4 Oct 2023.
Wickham H. Ggplot2. Wiley Interdiscip Rev Comput Stat. 2011;3:180–5.
Atieli H, Menya D, Githeko A, Scott T. House design modifications reduce indoor resting malaria vector densities in rice irrigation scheme area in western Kenya. Malar J. 2009;8:108.
Snyman K, Mwangwa F, Bigira V, Kapisi J, Clark TD, Osterbauer B, et al. Poor housing construction associated with increased malaria incidence in a cohort of young Ugandan children. Am J Trop Med Hyg. 2015;92:1207–13.
Chaves LF, Ramírez RM, Delgado JS, Prado M, Marín RR. Housing quality improvement is associated with malaria transmission reduction in Costa Rica. Socio-Econ. Plan. Sci. 2021;74:100951.
Ngadjeu CS, Ngadjeu CS, Doumbe-Belisse P, Doumbe-Belisse P, Talipouo A, Talipouo A, et al. Influence of house characteristics on mosquito distribution and malaria transmission in the city of Yaoundé, Cameroon. Malar J. 2020;19:53.
Njie M, Dilger E, Lindsay SW, Kirby MJ. Importance of eaves to house entry by Anopheline, but not Culicine mosquitoes. J Med Entomol. 2009;46:505–10.
Sperling S, Cordel M, Gordon S, Knols BGJ, Rose A. Eave tubes for malaria control in Africa: videographic observations of mosquito behaviour in Tanzania with a simple and rugged video surveillance system. Malariaworld J. 2017;8:9.
Perumal V, Kannan S, Pittarate S, Krutmuang P. A review of entomopathogenic fungi as a potential tool for mosquito vector control: a cost-effective and environmentally friendly approach. Entomol Res. 2024;54(3):e12717.
Odhiambo MTO, Vulule JM, Afrane YA, Ombok M, Bosselmann R, Skovmand O. Supplementary effect and durability of prototype insecticide-treated eave curtains on indoor resting mosquitoes in Kadibo division, Western Kenya. Malariaworld J. 2016;7:11.
Anaele BI, Varshney K, Ugwu FSO, Frasso R. The efficacy of insecticide-treated window screens and eaves against Anopheles mosquitoes: a scoping review. Malar J. 2021;20:388.
Kaindoa EW, Mmbando AS, Shirima R, Hape EE, Okumu FO. Insecticide-treated eave ribbons for malaria vector control in low-income communities. Malar J. 2021;20:415.
Mwanga EP, Mmbando AS, Mrosso PC, Stica C, Mapua SA, Finda MF, et al. Eave ribbons treated with transfluthrin can protect both users and non-users against malaria vectors. Malar J. 2019;18:314.
Swai JK, Mmbando AS, Ngowo HS, Odufuwa OG, Finda MF, Mponzi W, et al. Protecting migratory farmers in rural Tanzania using eave ribbons treated with the spatial mosquito repellent, transfluthrin. Malar J. 2019;18:414.
Mmbando AS, Ngowo H, Limwagu A, Kilalangongono M, Kifungo K, Okumu FO. Eave ribbons treated with the spatial repellent, transfluthrin, can effectively protect against indoor biting and outdoor biting malaria mosquitoes. Malar J. 2018;17:368.
Mmbando AS, Batista EPA, Kilalangongono M, Finda MF, Mwanga EP, Kaindoa EW, et al. Evaluation of a push-pull system consisting of transfluthrin-treated eave ribbons and odour-baited traps for control of indoor- and outdoor-biting malaria vectors. Malar J. 2019;18:87.
Knols BG, Mboera LE, Takken W. Electric nets for studying odour-mediated host-seeking behaviour of mosquitoes. Med Vet Entomol. 1998;12:116–20.
Maliti DV, Govella NJ, Killeen GF, Mirzai N, Johnson PCD, Kreppel K, et al. Development and evaluation of mosquito-electrocuting traps as alternatives to the human landing catch technique for sampling host-seeking malaria vectors. Malar J. 2015;14:502.
Hawkes F, Manin BO, Ng SH, Torr SJ, Drakeley C, Chua TH, et al. Evaluation of electric nets as means to sample mosquito vectors host-seeking on humans and primates. Parasit Vectors. 2017;10:338.
Jobe NB, Chourasia A, Smith BH, Molins E, Rose A, Pavlic TP, et al. Using electric fields to control insects: current applications and future directions. J Insect Sci. 2024;24:8.
Matowo NS, Koekemoer LL, Moore SJ, Mmbando AS, Mapua SA, Coetzee M, et al. Combining synthetic human odours and low-cost electrocuting grids to ttract and kill outdoor-biting mosquitoes: field and semi-field evaluation of an improved mosquito landing box. PLoS ONE. 2016;11:e0145653.
Hiscox A, Homan T, Mweresa CK, Maire N, Di Pasquale A, Masiga D, et al. Mass mosquito trapping for malaria control in western Kenya: study protocol for a stepped wedge cluster-randomised trial. Trials. 2016;17:356.
Acknowledgements
We thank volunteers who helped in collecting mosquitoes in the semi-field system: Moses Mlaganile and Robert Kasubili. We also thank Ms. Rukiyah Mohammad for administrative assistance throughout this study.
Funding
This study was supported by the Ifakara Health Institute (Environmental Health and Ecological Sciences department). EWK was supported by the Consortium for Advanced Research Training in Africa (CARTA). CARTA is jointly led by the African Population and Health Research Center and the University of the Witwatersrand and funded by the Carnegie Corporation of New York (Grant No. G-19-57145), Sida (Grant No: 54100113), Uppsala Monitoring Center and Norwegian Agency for Development Cooperation (Norad). K&S Consulting (Dodewaard, The Netherlands) paid for the electrocuting eave tubes.
Author information
Authors and Affiliations
Contributions
BGJK, DA, SK, CTC, HAS, ML, FOO and EWK conceptualized this study. BGJK, FOO and EWK designed this study, GF constructed the electrocuting eave tubes, BGJK developed the study protocol. RSS, GCK and EWK carried out data collection. RSS, EWK, GCK and ASM were involved in data entry, formal analysis, and manuscript writing. EWK, ASM, BGJK and FOO reviewed and edited drafts of the manuscript. RSS, GCK, EWK, BGJK, ASM, and FOO wrote the final manuscript. All authors read and approved the final manuscript.
Corresponding authors
Ethics declarations
Ethics approval and consent to participate
Study participants submitted signed written informed consent forms. Ethical approval for the study was granted by the Institutional Review Board of Ifakara Health Institute (Clearance no. IHI/IRB/No:37-2022) and the Medical Research Coordination Committee of the National Institute for Medical Research in Tanzania (Clearance no: NIMR/HQ/R.8a/Vol.IX/4156). The permission to publish this manuscript was obtained from the National Institute for Medical Research (NIMR) (Ref. no: BD.242/437/01C/7).
Consent for publication
Not applicable.
Competing interests
Fredros Okumu, a Subject Editor for Parasites and Vectors, was not involved in the peer review of this paper. The authors declare that they have no other competing interests.
Additional information
Publisher's Note
Springer Nature remains neutral with regard to jurisdictional claims in published maps and institutional affiliations.
Rights and permissions
Open Access This article is licensed under a Creative Commons Attribution 4.0 International License, which permits use, sharing, adaptation, distribution and reproduction in any medium or format, as long as you give appropriate credit to the original author(s) and the source, provide a link to the Creative Commons licence, and indicate if changes were made. The images or other third party material in this article are included in the article's Creative Commons licence, unless indicated otherwise in a credit line to the material. If material is not included in the article's Creative Commons licence and your intended use is not permitted by statutory regulation or exceeds the permitted use, you will need to obtain permission directly from the copyright holder. To view a copy of this licence, visit http://creativecommons.org/licenses/by/4.0/. The Creative Commons Public Domain Dedication waiver (http://creativecommons.org/publicdomain/zero/1.0/) applies to the data made available in this article, unless otherwise stated in a credit line to the data.
About this article
Cite this article
Shirima, R.S., Katusi, G.C., Mmbando, A.S. et al. Semi-field evaluation of electrocuting eave tubes for the control of endophagic mosquitoes in south-east Tanzania. Parasites Vectors 17, 349 (2024). https://doi.org/10.1186/s13071-024-06407-1
Received:
Accepted:
Published:
DOI: https://doi.org/10.1186/s13071-024-06407-1